Gambogic acid affects high glucose-induced apoptosis and inflammation of retinal endothelial cells through the NOX4/NLRP3 pathway
Highlight box
Key findings
• This study found that gambogic acid can inhibit the apoptosis and inflammatory reaction of HRECs under high glucose conditions by inhibiting the activity of the NOX4/NLRP3 pathway.
What is known and what is new?
• Under high glucose conditions, NOX4 can induce the activation of inflammatory bodies such as NLRP3 and induce an inflammatory response. However, it is not clear whether the treatment of diabetic retinopathy with GA is related to the activity of NOX4/NLRP3 pathway.
• Our study explored the effect of gambogic acid on the apoptosis and inflammatory response of retinal endothelial cells through in vitro experiments and investigated the effect of gambogic acid on the activity of the NOX4/NLRP3 pathway under high glucose conditions.
What are the implications, and what should change now?
• Gambogic acid has an obvious inhibitory effect on diabetic retinopathy, which is worthy of follow-up research.
Introduction
Diabetes mellitus is a metabolic disorder that threatens the health of individuals worldwide. It is characterized by abnormally elevated blood glucose. This chronic disease affects a variety of organs and causes various complications in patients over a long period (1). The main effect of diabetes on the eye is diabetic retinopathy. Long-term hyperglycemia can lead to retinal microangiopaplasia, which is a common and extremely serious complication in patients with diabetes (2). Statistics show that more than one-third of diabetic patients are accompanied by retinopathy of varying degrees. Blindness caused by diabetic retinopathy seriously affects the quality of life of patients and increases their health burden (3). With the continuous improvements in the quality of life of the population, it is expected that there will be more than one billion people with diabetes globally by 2030, and more than 90 million patients will have diabetic retinopathy (4). The development of diabetes is closely related to the inflammatory response (5). Researchers have found increased levels of inflammatory factors such as interleukin-1β (IL-1β) and interleukin-6 (IL-6) in aqueous humor and serum of patients with diabetic retinopathy. Endothelial cells are an important part of retinal microvessels and play an important role in maintaining the integrity of retinal structure and function. High glucose stimulation can induce retinal endothelial cell inflammation. NADPH oxidase (NOX) is an important enzyme system to produce oxidative stress in cells. Reactive oxygen species (ROS) produced by NOX4 can cause endothelial cell damage, induce the activation of nucleotide-binding oligomerization domain-like receptor protein 3 (NLRP3) inflammasome and induce inflammatory response. NLRP3 inflammasome consists of NLRP3, Apoptosis-associated speck-like protein containing CARD (ASC) and Caspase-1, which plays an important role in human diseases, especially in the immune system. Inhibition of NOX4/NLRP3 pathway activity can significantly inhibit high glucose-induced retinal endothelial cell injury (6).
Garcinic acid (GA) is one of the main active components extracted from the dried resin secreted by Garcinia tenuifolia, a plant of the family Garcinaceae, which exerts anti-tumor effects and is less toxic to the immune system. In addition, GA also has anti-infection, anti-oxidation, and anti-virus effects (7). A study has shown that GA has a good therapeutic effect on diabetic retinopathy, and it also significantly reduces the inflammatory response of retinal endothelial cells (4).
At present, it is not clear whether the treatment of diabetic retinopathy with GA is related to the activity of NOX4/NLRP3 pathway. This study will explore the effect of GA on the apoptosis and inflammation of retinal endothelial cells through in vitro experiments and investigate the effect of GA on the activity of the NOX4/NLRP3 pathway under high glucose conditions. We present the following article in accordance with the MDAR reporting checklist (available at https://atm.amegroups.com/article/view/10.21037/atm-22-6591/rc).
Methods
Main materials and reagents
The human retinal endothelial cell (HREC) line was purchased from FuHeng Cell Center (Shanghai, China). GA (purity ≥97%), glucose (analytically pure), mannitol (analytically pure), and Radio Immunoprecipitation Assay (RIPA) lysates were purchased from Shanghai MacLean Biochemical Technology Co., Ltd. (China). Dulbecco’s modified eagle medium (DMEM) medium, phosphate buffered saline (PBS) buffer, Tris Buffered Saline with Tween-20 (TBST), skim milk powder, and fetal bovine serum (FBS) were purchased from Shanghai Yuanye Biotechnology Co., Ltd. (China). The Annexin-fluorescein isothiocyanate (FITC)/propidium iodide (PI) Apoptosis Detection Kit and 3-(4,5-dimethylthiazol-2-yl)-2,5-diphenyltetrazolium bromide (MTT) kit were purchased from Beijing Solebo Technology Co., Ltd. (China). The B-cell lymphoma-2 (Bcl-2) antibody, the Bcl-2 homologous water-soluble-related protein (Bcl-2-associated X), Bax antibody, pro-Caspase-3 (p-Caspase-3) antibody, cleaved-Caspase-3 (c-Caspase-3) antibody, pro-Caspase-9 (p-Caspase-9) antibody, cleaved-Caspase-9 (c-Caspase-9) antibody, NOX4 antibody (1:2,000), NLRP3 antibody (1:2,000), pro-Caspase-1 (p-Caspase-1) antibody, cleaved-Caspase-1 (c-Caspase-1) antibody, IL-1β antibody, glyceraldehyde-3-phosphate dehydrogenase (GAPDH) antibody, and horseradish peroxidase-labeled immunoglobulin G (IgG) antibody were purchased from Shanghai Gudao Biotechnology Co., Ltd. (China). IL-1β, IL-8, IL-10, and tumor necrosis factor-α (TNF-α) were used in this study. The TNF-α enzyme linked immunosorbent assay (ELISA) kit was purchased from Shanghai Yiyan Biotechnology Co., Ltd. (China). The RIPA lysate, bicinchoninic acid (BCA) kit, and enhanced chemiluminescence (ECL) chemiluminescence kit were purchased from Apixin (Shanghai) Biotechnology Co., Ltd. (China). The TRIzol reagent, RNA reverse transcription kit, and reverse transcription-polymerase chain reaction (RT-PCR) kit were purchased from Shanghai Siege Biotechnology Co., Ltd. (China).
Cell culture and transfection
Frozen HRECs were routinely resuscitated and seeded into a DMEM medium containing 10% FBS and 1% penicillin-streptomycin solution at 37 ℃ in 5% carbon dioxide (CO2). When the cells were fused to about 80%, they were digested with 0.25% trypsin and subcultured. Cells in the logarithmic growth phase were divided into four groups: the cells cultured without glucose medium were the control group (NC group); the cells cultured with 5.5 mM glucose and 24.5 mM mannitol were the low glucose group (NG group); the cells cultured with 30 mM glucose were the high glucose group (HG group); and the cells cultured with 24.5 mM mannitol were the mannitol group (E421 group).
HRECs in the logarithmic growth phase were obtained in strict accordance with LipofectamineTM. The overexpression empty vector (pcDNA3.1-NC), NOX4 overexpression vector (pcDNA3.1-NOX4), disordered nonsense negative vector (si-NC), and NOX4 inhibitory vector (si-NOX4) were transfected into HRECs. The transfected HRECs cells were divided into an overexpression negative control group (pc-NC group), a NOX4 overexpression group (pc-NOX4 group), an inhibition negative control group (si-NC group), and a NOX4 inhibition group (si-NOX4 group).
The cells were treated with GA
HRECs in the logarithmic growth phase were treated with 0, 0.5, 1, 2, 5, 10, 20, 50, and 100 µM of GA for 24 h, and then the cell viability was detected by MTT. Cells cultured with 30 mM glucose +15 µM gambogic acid were divided into a high glucose + gambogic acid treatment group (HG + GA group).
Flow cytometry was used to detect cell apoptosis
HRECs in the logarithmic growth phase were digested by trypsin and resuspended. Annexin-FITC (10 µL) was added to the resuspended cells, and the cells were incubated for 20 min at room temperature under a light. Then, 5 µL of PI was added to the cells and gently mixed, and the cells were incubated for 15 min at room temperature. Following incubation, 500 µL of buffer was added to the cells, and the apoptosis level of cells in each group was detected by flow cytometry.
Western blotting (WB) was used to detect the protein expression level
RIPA lysate was used to extract the total protein from each group of cells, lysed on ice for 30 min, centrifuged at 4 ℃ for 10 min (12,000 r/min), and the supernatant was collected. The protein purity and concentration were determined by BCA. Proteins were separated by 10% sodium dodecyl sulfate polyacrylamide gel electrophoresis (SDS-PAGE), and the separated proteins were transferred to a polyvinylidene fluoride (PVDF) membrane, which was blocked with 5% skim milk at room temperature. After 2 h, the Bcl-2 (1:2,000), Bax (1:2,000), p-Caspase-3 (1:2,000), c-Caspase-3 (1:2,000), p-Caspase-9 (1:2,000), c-Caspase-9 (1:2,000), c-Caspase-9 (1:2,000), NOX4 (1:2,000), NLRP3 (1:2,000), ASC (1:2,000), p-Caspase-1 (1:2,000), c-Caspase-1 (1:2,000), IL-1β (1:2,000), and GAPDH (1:2,000) antibodies were incubated overnight at 4 ℃.
The next day, the membrane was washed with TBST, then horseradish peroxidase-labeled IgG antibody was added, and the membrane was incubated for 2 h at room temperature. After TBST washing, an ECL chemiluminescence kit was used to develop the film in the dark, a gel imaging system was used to develop the image, and the gray value of the band was scanned using ImageJ software (Media Cybernetics, USA). Each experiment was repeated three times.
The levels of inflammatory cytokines in the cell supernatant were detected by ELISA
The supernatant of each group was collected and transferred to the sample plate, and each well contained 50 µL of cell supernatant. After loading, the plates were sealed and incubated for 30 min in a constant-temperature incubator. After washing, 50 µL of IL-6, IL-8, IL-10, and TNF-α horseradish peroxidase (HRP)-conjugated reagents were added to each well, and the plates were incubated in a constant-temperature incubator for 30 min. Following incubation, a chromogenic reagent was added to each well, and the chromogenic reaction was terminated by adding the stop solution at room temperature for 10 min in the dark. The absorbance value of each well at 450 nm was detected using a microplate reader (Spectrauor, TECAN, Sunrise, Austria), and the concentration of each factor was expressed as pg/mL after conversion. Each experiment was repeated three times.
RT-PCR was used to detect the mRNA levels in the cells
Total RNA in each group was collected using TRIzol extract, and complementary DNA (cDNA) was synthesized after reverse transcription. Using GAPDH as the internal reference, the mRNA levels of each factor were quantified by the RT-PCR kit. The primer sequences are shown in Table 1. The PCR reaction system consisted of 10 µL TB Green Premix Ex Taq II, 0.8 µL positive and negative PCR primers, 0.4 µL ROX reference dye, 2 µL DNA template, and 6 µL on hydrogen peroxide (H2O2). The PCR reaction conditions were as follows: predenaturation at 95 ℃ for 30 s, denaturation at 95 ℃ for 5 s, annealing at 60 ℃ for 34 s, and 40 cycles in total. The relative mRNA expression levels of each factor were determined by 2−ΔΔCt. Each experiment was repeated three times.
Table 1
Target | Primer sequence |
---|---|
GAPDH | F: 5'-GAAGGTGAAGGTCGGAGTC-3' |
R: 5'-GAAGATGGTGATGGGATTTC-3' | |
IL-6 | F: 5'-GGCCCTTGCTTTCTCTCG-3' |
R: 5'-ATAATAAAGTTTTGATTATGT-3' | |
IL-8 | F: 5'-TCTGCAGCTCTGTGTGAAGG-3' |
R: 5'-AATTTCTGTGTTGGCGCAGT-3' | |
IL-10 | F: 5'-CACTGCTCTGTTGCCTGGTC-3' |
R: 5'-TCGAAGCATGTTAGGCAGGTT-3' | |
TNF-α | F: 5'-CAGCCTCTTCTCCTTCCTGA-3' |
R: 5'-GGAAGACCCCTCCCAGATAGA-3' |
F, forward primer, R, reverse primer; GAPDH, glyceraldehyde-3-phosphate dehydrogenase; IL, interleukin; TNF-α, tumor necrosis factor-α.
Cell viability was determined by MTT
HRECs from each group were resuspended and seeded into 96-well plates with 100 µL of cell suspension (1×105) in each well. The cells were incubated in a constant temperature incubator for 24 h. Next, 20 µL of MTT solution (5 mg/mL) was added to each well, and the cells were incubated in a constant temperature incubator for 4 h. One hundred µL dimethyl sulfoxide (DMSO) was added to each well and mixed to stop the reaction. Then, the absorbance value [optical density (OD)] of each well at 490 nm, which reflected the cell activity in each well, was measured using a microplate reader. Each experiment was repeated three times.
Statistical analysis
SPSS 22.0 software (SPSS® Inc., Chicago, IL, USA) was used to analyze the data, and GraphPad Prism 8.2.1 (GraphPad Software, Inc., La Jolla, San Diego, CA, USA) was used to draw the data. Data were expressed as mean ± standard deviation, and pairwise comparison between groups was performed using the t-test. Single factor analysis of variance was used for comparison among groups. P<0.05 was considered statistically significant.
Results
High glucose induces apoptosis and inflammation in HRECs
In this study, HRECs were cultured under high glucose conditions to simulate diabetic retinopathy in vitro. Flow cytometry was used to detect the apoptosis level of HRECs. Compared with the blank control group, the apoptosis levels of the low glucose group and the mannitol group were not significantly changed, while the apoptosis level of the high glucose group was markedly increased (P<0.05), as shown in Figure 1A. The WB assay was used to detect the expression levels of apoptosis-related proteins in HRECs. Compared with the blank control group, the protein expression levels of Bcl-2, Bax, c-Caspase 3, and c-Caspase 9 in the low glucose and mannitol groups did not change significantly. In the high glucose group, the levels of anti-apoptotic protein Bcl-2 were substantially decreased (P<0.05), while the levels of pro-apoptotic proteins Bax, c-Caspase-3, and c-Caspase-9 were notably increased (P<0.05), as shown in Figure 1B.
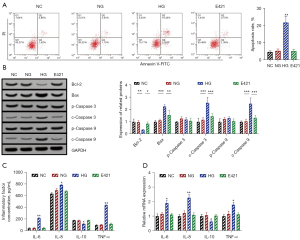
The ELISA assay was applied to detect the levels of inflammatory factors in the supernatant of HRECs in each group. Compared with the blank control group, the levels of IL-6, IL-8, IL-10, and TNF-α in the supernatant of HRECs cells in the low-glucose and mannitol groups showed no significant changes. The levels of IL-6, IL-8, and TNF-α in the supernatant of HRECs cells in the high glucose group were markedly increased (P<0.05), while the level of IL-10 was significantly decreased (P<0.05), as shown in Figure 1C.
RT-qPCR was utilized to detect the relative expression levels of inflammatory cytokines mRNA in each group. Compared with the blank control group, the relative mRNA expression levels of IL-6, IL-8, IL-10, and TNF-α in the low-glucose and mannitol groups did not change notably. In the high glucose group, the relative mRNA expression levels of IL-6, IL-8, and TNF-α were significantly increased (P<0.05), while those of IL-10 were considerably decreased (P<0.05), as shown in Figure 1D.
Effect of GA on the viability of HRECs
The MTT assay was used to detect the cell viability of HRECs. The results showed that the cell viability was not significantly affected when the concentration of GA was less than 20 µM (<20 µM) but was markedly decreased when the concentration was greater than 20 µM (>20 µM) (P<0.05). We also observed an obvious concentration dependence, as shown in Figure 2A.
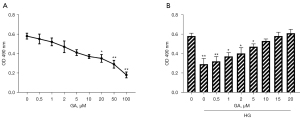
The cell viability of high glucose-treated cells was significantly inhibited (P<0.05). When the concentration of GA reached 15 µM, the cell viability was comparable to that of the blank control group, and there was no damage to the cells. Therefore, 15 µM of GA was selected for subsequent experiments, as shown in Figure 2B.
GA inhibits apoptosis and inflammation of HRECs induced by high glucose
The flow cytometry results showed that 15 µM of GA treatment could significantly inhibit the increase of HRECs apoptosis levels induced by high glucose (P<0.05). At the same time, the intracellular levels of anti-apoptotic Bcl-2 were significantly increased (P<0.05), and the levels of pro-apoptotic proteins Bax, c-Caspase-3, and c-Caspase-9 were markedly decreased (P<0.05), as shown in Figure 3A,3B.
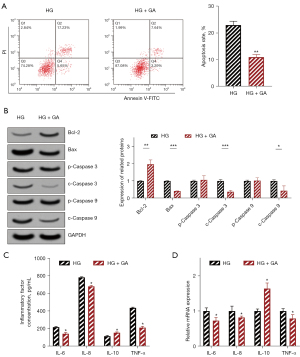
In addition, the ELISA results showed that the levels of IL-6, IL-8, and TNF-α in the supernatant of HRECs cultured in high glucose culture were significantly decreased (P<0.05), and those of IL-10 were increased (P<0.05) after treatment with 15 µM of GA, as shown in Figure 3C. The RT-qPCR results also showed that the relative mRNA expression levels of IL-6, IL-8, and TNF-α were markedly decreased (P<0.05), and those of IL-10 were notably increased (P<0.05) in HRECs treated with 15 µM of GA, as shown in Figure 3D.
GA inhibits high glucose-induced activation of the NOX4/NLRP3 pathway
The WB detection results showed that the protein expression level of NOX4 in HRECs in the high glucose group was significantly increased (P<0.05), and the expression levels of the NLRP3 inflammasome components (NLRP3, ASC, p-Caspase 1, c-Caspase 1) and the downstream molecule, IL-1β, were also notably increased (P<0.05). Compared with the high glucose group, the intracellular protein expression level of NOX4 was decreased after treatment with 15 µM of GA (P<0.05), and the expression levels of the NLRP3 inflammasome components (NLRP3, ASC, p-Caspase 1, c-Caspase 1) and the downstream molecule, IL-1β, were also significantly decreased (P<0.05), as shown in Figure 4.
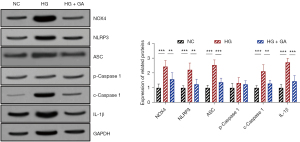
The NOX4/NLRP3 pathway regulates apoptosis and inflammation in HRECs
The activity of the NOX4/NLRP3 pathway in HRECs was regulated by pc-NOX4 or si-NOX4 transfection. The WB assay results showed that the intracellular NOX4 protein and NLRP3 inflammasome components (NLRP3, ASC, p-Caspase 1, c-Caspase 1) were significantly increased after pc-NOX4 transfection (P<0.05), and the expression of the downstream molecule, IL-1β, was also markedly increased (P<0.05). The expression levels of the intracellular NOX4 protein, NLRP3 inflammasome components (NLRP3, ASC, p-Caspase 1, c-Caspase 1), and IL-1β were significantly decreased after transfection with si-NOX4 (P<0.05), suggesting that transfection of pc-NOX4 and si-NOX4 could promote or inhibit the activity of the intracellular NOX4/NLRP3 pathway in HRECs, as shown in Figure 5A.
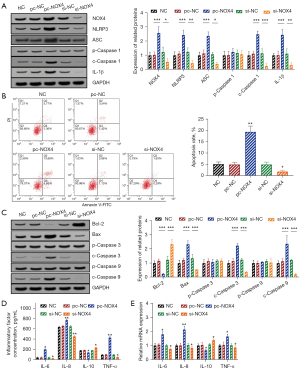
Flow cytometry showed that activation of the NOX4/NLRP3 pathway significantly increased the apoptosis level of HRECs (P<0.05), while inhibition of the NOX4/NLRP3 pathway substantially inhibited the apoptosis of HRECs (P<0.05). The expression levels of apoptosis-related proteins in cells with NOX4/NLRP3 pathway activation or inhibition were also changed (P<0.05), as shown in Figure 5B,5C.
The ELISA results showed that the levels of IL-6, IL-8, and TNF-α in the cell supernatant were significantly increased after activation of the NOX4/NLRP3 pathway (P<0.05), while that of IL-10 was markedly decreased (P<0.05). After inhibiting the NOX4/NLRP3 pathway, the levels of IL-6, IL-8, and TNF-α in the cell supernatant were significantly decreased (P<0.05), while those of IL-10 were notably increased (P<0.05), as shown in Figure 5D.
The RT-qPCR results showed that NOX4/NLRP3 pathway activation significantly up-regulated the relative mRNA expression levels of IL-6, IL-8, and TNF-α (P<0.05) and markedly down-regulated those of IL-10 (P<0.05). NOX4/NLRP3 pathway inhibition had the opposite effect (P<0.05), as shown in Figure 5E.
Discussion
Diabetes mellitus is characterized by abnormally elevated blood glucose, and hyperglycemia is thought to play an important role in diabetic retinopathy (8). At present, the main treatment methods for diabetic retinopathy are surgery and drug therapy but the treatment effects of current therapies are not ideal for some patients (9). A study has shown that the increased apoptosis of retinal microvascular pericytes and endothelial cells under high glucose conditions can lead to negative effects on the retinal microvascular system and also induced a variety of inflammatory responses (10). In this study, HRECs were cultured under high glucose conditions to simulate diabetic retinopathy in vitro. The apoptosis level of HRECs was significantly increased under high glucose conditions, and the expression levels of cell supernatant and intracellular inflammatory factors were also markedly increased, indicating that the established in vitro cell model was successful.
Increasing evidence has shown that the active extracts of various plants, such as curcumin, have good therapeutic effects on diabetic retinopathy (11), astragalus polysaccharide (12), resveratrol (13), naringin (14), etc. GA exerts a variety of biological functions, including anti-inflammatory, antiviral, and antiviral effects (15). In addition, GA has good antitumor activity in vitro, which can inhibit the proliferation and induce the apoptosis of human liver cancer cells, gastric cancer cells, lung cancer cells, pancreatic cancer cells, breast cancer cells, and cervical cancer cells (16). Cui et al. (4) have shown that gambosa can reduce retinal angiogenesis, inhibit the expression of hypoxia inducible factor (HIF)-1α, and the activation of the phosphatidylinositol-4,5-bisphosphate 3-kinase/protein kinase B (PI3K/AKT) signaling pathway in diabetic mice. At the same time, it can inhibit the migration and angiogenesis of retinal endothelial cells induced by high glucose in vitro by inhibiting the HIF-1α/vascular endothelial growth factor (VEGF) and PI3K/AKT signaling pathways. In this study, it was found that a low concentration of GA (<20 µM) had no significant effect on the activity of HRECs, and 15 µM of GA could restore the decreased activity of retinal endothelial cells caused by high glucose. It was also found that 15 µM of GA could significantly inhibit the increase of apoptosis induced by high glucose as well as the levels of inflammatory factors in cell supernatants and cells.
One study has shown that NLRP3 inflammasome activation is closely related to endothelial cell damage in diabetic retinopathy (6). Under high glucose conditions, the increased expression of NOX4 in retinal endothelial cells induces oxidative stress and an inflammatory response. ROS produced during oxidative stress are important intermediate molecules in the activation of the NLRP3 inflammasome. Chen et al. (17) reported that the use of antioxidants or NOX inhibitors inhibits retinal leakage in diabetic mice, and Li et al. (6) showed that NOX4/NLRP3 pathway inhibition can protect against vascular endothelial cell damage in diabetic rat models, suggesting that the NOX4/NLRP3 pathway plays an important role in diabetic ocular lesions.
In this study, we found that the expression levels of NOX4 protein and NLRP3 inflammasome components were increased in HRECs under high glucose conditions, and the expression level of IL-1β, the downstream inflammatory factor of NLRP3, was also significantly increased. NOX4/NLRP3 pathway activation in HRECs was significantly inhibited by 15 µM of GA under high glucose conditions. Transfection of pc-NOX4 or si-NOX4 could regulate the activity of the NOX4/NLRP3 pathway in HRECs. The apoptosis level and inflammatory factors were significantly up-regulated following transfection of pc-NOX4, and si-NOX4 could inhibit cell both apoptosis and the inflammatory response.
Endothelial cells are connected to each other by connexins, such as zonula occludens 1 (ZO-1) and vascular endothelial cadherin (VE-Cad), to maintain endothelial permeability. It has been shown that activation of the NLRP3 inflammasome degrades endothelial connexin (18). At the same time, IL-1β released by NLRP3 inflammasome activation can also reduce the adhesion strength of vascular endothelial cells, lead to increased vascular permeability, and induce retinal microvascular leakage (19), thereby increasing the risk of blindness. In the subsequent study, we will establish a diabetic rat model to investigate the effects of gambosa and NOX4/NLRP3 on diabetic retinopathy at the animal level to provide a theoretical basis for the clinical use of gambosa in the treatment of diabetic retinopathy.
In conclusion, this study found that gambosa could inhibit the apoptosis and inflammatory response of HRECs under high glucose conditions by inhibiting the activity of the NOX4/NLRP3 pathway. This has a significant inhibitory effect on the reduction of diabetic retinopathy, which is worthy of further study.
Conclusions
GA can inhibit Nox4/NLRP3 pathway activity, thus inhibit HRECS cell apoptosis and inflammation under high glucose condition. GA has a significant inhibitory effect on relieving diabetic retinopathy.
Acknowledgments
Funding: None.
Footnote
Reporting Checklist: The authors have completed the MDAR reporting checklist. Available at https://atm.amegroups.com/article/view/10.21037/atm-22-6591/rc
Data Sharing Statement: Available at https://atm.amegroups.com/article/view/10.21037/atm-22-6591/dss
Peer Review File: Available at https://atm.amegroups.com/article/view/10.21037/atm-22-6591/prf
Conflicts of Interest: All authors have completed the ICMJE uniform disclosure form (available at https://atm.amegroups.com/article/view/10.21037/atm-22-6591/coif). The authors have no conflicts of interest to declare.
Ethical Statement: The authors are accountable for all aspects of the work in ensuring that questions related to the accuracy or integrity of any part of the work are appropriately investigated and resolved.
Open Access Statement: This is an Open Access article distributed in accordance with the Creative Commons Attribution-NonCommercial-NoDerivs 4.0 International License (CC BY-NC-ND 4.0), which permits the non-commercial replication and distribution of the article with the strict proviso that no changes or edits are made and the original work is properly cited (including links to both the formal publication through the relevant DOI and the license). See: https://creativecommons.org/licenses/by-nc-nd/4.0/.
References
- Thipsawat S. Early detection of diabetic nephropathy in patient with type 2 diabetes mellitus: A review of the literature. Diab Vasc Dis Res 2021;18:14791641211058856. [Crossref] [PubMed]
- Azad R, Sinha S, Nishant P. Asymmetric diabetic retinopathy. Indian J Ophthalmol 2021;69:3026-34. [Crossref] [PubMed]
- Lin KY, Hsih WH, Lin YB, et al. Update in the epidemiology, risk factors, screening, and treatment of diabetic retinopathy. J Diabetes Investig 2021;12:1322-5. [Crossref] [PubMed]
- Cui J, Gong R, Hu S, et al. Gambogic acid ameliorates diabetes-induced proliferative retinopathy through inhibition of the HIF-1α/VEGF expression via targeting PI3K/AKT pathway. Life Sci 2018;192:293-303. [Crossref] [PubMed]
- Dong GZ, Zhang QY, Jiao YW, et al. The contribution of type 2 diabetes mellitus to hypothalamic inflammation and depressive disorders in young patients with obesity. Ann Transl Med 2022;10:134. [Crossref] [PubMed]
- Li XX, Ling SK, Hu MY, et al. Protective effects of acarbose against vascular endothelial dysfunction through inhibiting Nox4/NLRP3 inflammasome pathway in diabetic rats. Free Radic Biol Med 2019;145:175-86. [Crossref] [PubMed]
- Li M, Su F, Zhu M, et al. Research Progress in the Field of Gambogic Acid and Its Derivatives as Antineoplastic Drugs. Molecules 2022;27:2937. [Crossref] [PubMed]
- Zheng S, Huang K, Tong T. Efficacy and Mechanisms of Oleuropein in Mitigating Diabetes and Diabetes Complications. J Agric Food Chem 2021;69:6145-55. [Crossref] [PubMed]
- Wang W, Lo ACY. Diabetic Retinopathy: Pathophysiology and Treatments. Int J Mol Sci 2018;19:1816. [Crossref] [PubMed]
- Rübsam A, Parikh S, Fort PE. Role of Inflammation in Diabetic Retinopathy. Int J Mol Sci 2018;19:942. [Crossref] [PubMed]
- Radomska-Leśniewska DM, Osiecka-Iwan A, Hyc A, et al. Therapeutic potential of curcumin in eye diseases. Cent Eur J Immunol 2019;44:181-9. [Crossref] [PubMed]
- Zhang Z, Zhang L, Xu H. Effect of Astragalus polysaccharide in treatment of diabetes mellitus: a narrative review. J Tradit Chin Med 2019;39:133-8.
- Huang DD, Shi G, Jiang Y, et al. A review on the potential of Resveratrol in prevention and therapy of diabetes and diabetic complications. Biomed Pharmacother 2020;125:109767. [Crossref] [PubMed]
- Liu L, Zuo Z, Lu S, et al. Naringin attenuates diabetic retinopathy by inhibiting inflammation, oxidative stress and NF-κB activation in vivo and in vitro. Iran J Basic Med Sci 2017;20:813-21. [Crossref] [PubMed]
- Ma J, Huang K, Ma Y, et al. Gambogic acid inhibits LPS-induced macrophage pro-inflammatory cytokine production mainly through suppression of the p38 pathway. Iran J Basic Med Sci 2018;21:717-23. [Crossref] [PubMed]
- Hatami E, Jaggi M, Chauhan SC, et al. Gambogic acid: A shining natural compound to nanomedicine for cancer therapeutics. Biochim Biophys Acta Rev Cancer 2020;1874:188381. [Crossref] [PubMed]
- Chen P, Guo AM, Edwards PA, et al. Role of NADPH oxidase and ANG II in diabetes-induced retinal leukostasis. Am J Physiol Regul Integr Comp Physiol 2007;293:R1619-29. [Crossref] [PubMed]
- Peng Y, Li H, Chen D. Silencing astrocyte elevated gene-1 attenuates lipopolysaccharide-induced inflammation and mucosal barrier injury in NCM460 cells by suppressing the activation of NLRP3 inflammasome. Cell Biol Int 2019;43:56-64. [Crossref] [PubMed]
- Boini KM, Hussain T, Li PL, et al. Trimethylamine-N-Oxide Instigates NLRP3 Inflammasome Activation and Endothelial Dysfunction. Cell Physiol Biochem 2017;44:152-62. [Crossref] [PubMed]
(English Language Editor: A. Kassem)