SCD1 deficiency exacerbates osteoarthritis by inducing ferroptosis in chondrocytes
Highlight box
Key findings
• Our study demonstrated that SCD1 deficiency exacerbates osteoarthritis by inducing ferroptosis in chondrocytes.
What is known and What is new?
• Abnormal fatty acid metabolism and chondrocyte ferroptosis play a role in osteoarthritis (OA) development. SCD1 inhibits the development of ferroptosis in various kinds of cancer cells.
• Unsaturated fatty acid metabolism is reduced in osteoarthritic chondrocytes. SCD1 deficiency in mouse chondrocytes accelerates cartilage degeneration after injury. The SCD1-PPARG axis regulates ferroptosis in chondrocytes in vivo.
What is the implication, and what should change now?
• The SCD1-PPARG axis is a potential target for the treatment of OA.
Introduction
Osteoarthritis (OA) is the most common degenerative joint disease in the world and is also the leading cause of joint disability in the elderly (1). The main pathological feature of OA is articular cartilage degeneration with a variety of pathological changes, including cartilage loss, subchondral bone sclerosis, and synovial inflammation (2). However, the molecular mechanisms underlying the pathogenesis of OA are mostly unknown. Although OA is reported to be associated with unsaturated fatty acid metabolism disorders (3,4), the significance of different Stearoyl-CoA desaturases in the pathogenesis of OA has not been fully illustrated.
Stearoyl-CoA desaturase (SCD1) is a rate-limiting enzyme in the anabolism of unsaturated fatty acids that transforms saturated fatty acids (SFA) into monounsaturated fatty acids (MUFA). Synthetic MUFA and SFA bind to a wide range of lipid classes, including triglycerides (TG) and phospholipids (PL), which are both essential components of cell membranes (5). SCD1 is considered an essential regulator of lipid homeostasis, particularly in the liver and adipose tissue. The regulation of SCD1 activity has been proven to be associated with the development of metabolic syndrome and inflammatory states (6,7). In addition, SCD1 catalytic product MUFA to compete with polyunsaturated fatty acids (PUFA) for incorporation into phospholipids on the cell membrane and exhibit anti-lipid peroxidation effects in modulating the de novo lipogenesis network (8). Lastly, SCD1 activation has been demonstrated to enhance the Akt pathway, presumably promoting the transcription of lipogenic enzymes, such as the sterol regulatory element binding protein (SREBP) family members, and thereby stimulating lipid synthesis and membrane formation (9). These findings imply that SCD1 is critical in maintaining cell membrane structural components and stability. So, might SCD1 affect chondrocyte destiny and thus the incidence and progression of OA via modulating lipid balance and cell membrane components?
Ferroptosis is a type of programmed cell death that induces lipid peroxidation of highly abundant PUFA on the cell membrane under the catalysis of ferrous iron or ester oxygenase and cell death (10). It is also associated with neurodegenerative diseases, cardiovascular diseases, and various cancers (11,12). Because chondrocytes are the only cell type in articular cartilage, their survival is critical to maintaining the integrity of the cartilage (13). Ferroptosis in OA has been extensively studied. Increasing data suggests that chondrocyte ferroptosis is linked to the etiology of OA (14). In addition, systemic iron overload and enhanced intracellular iron uptake can promote and aggravate OA in animal models (15). Furthermore, it has also been shown that ferrostatin-1 (Fer-1, a ferroptosis inhibitor) can reduce OA progression (16). Meanwhile, it has been proved that a high level of SCD1 can inhibit the development of ferroptosis in various kinds of cancer cells, including lung, pancreatic, and colorectal cancer (17,18). However, the function of SCD1 in chondrocyte ferroptosis is unknown.
Our previous study revealed that SCD1 protein expression was reduced in human OA cartilage tissue specimens. Also, Gene Set Enrichment Analysis (GSEA) and Gene Ontology (GO) analysis of the GSE176199 dataset showed that unsaturated fatty acid synthesis was inhibited in human OA chondrocytes. While differential genes were enriched on cell membrane components, we hypothesize that deficiency of SCD1 exacerbates OA by inducing ferroptosis in chondrocytes.
Therefore, the present study aimed to investigate the role of the SCD1 protein in the degenerative process of OA. We present the following article in accordance with the ARRIVE reporting checklist (available at https://atm.amegroups.com/article/view/10.21037/atm-22-6630/rc).
Methods
Data collection and preparation
The GSE176199 gene expression profile dataset was acquired from the Gene Expression Omnibus (GEO) website; the probes were transformed to the matching gene names based on the annotation information of the GPL16791 high-throughput sequencer platform (Homo Sapiens) (Illumina HiSeq 2500).
Participant consent and ethical approval
Human cartilage samples were collected from 10 patients who had total knee arthroplasty and 5 patients who had traumatic amputation. Inclusive criteria: (I) age 50–70; (II) patients with knee osteoarthritis, K-L grade 3 or above, who need total knee replacement; (III) or healthy patients who need amputation due to trauma. Exclusion criteria: (I) age over 70 years old or less than 50 years old; (II) arthritis patients with rheumatic, rheumatoid, gout, traumatic arthritis or other autoimmune diseases; (III) have received arthroscopic surgery during the period; (IV) patients with serious medical diseases or chronic immune system diseases. The study was conducted in accordance with the Declaration of Helsinki (as revised in 2013). The study was approved by ethics board of Chongqing Medical University’s University-Town Hospital (No. 2021.5.28/LL-202133) and informed consent was taken from all the patients.
Animals and animal experiments
8-week-old male WT (C57BL/6J, weight 18–22 g) mice were provided by the Experimental Animal Center of Chongqing Medical University (Chongqing China) and 8-week-old male SCD1-KO (C57BL/6J, weight 18–22 g) mice were acquired from CYAGEN Biotechnology Company (Serial Number: KOCMP-21645-Scd1). The above mice had been randomly assigned and raised at Chongqing Medical University of Sciences Experimental Animal Center. The mice were housed for 12 hours at a constant temperature of 22.2 ℃, relative humidity of 55%±5%, and in a 12h-12h light-dark cycle condition. Animal experiments were performed under a project license (No. 2021.5.28/LL-202133) granted by the ethics board of the University-Town Hospital of Chongqing Medical University, in compliance with national guidelines for the care and use of animals. The WT mice (C57BL/6J, n=20) were randomly grouped and then subjected to sham or right knee medial meniscus surgery-induced OA (DMM model) (19). The WT (C57BL/6J, n=10) and SCD1-KO (C57BL/6J, n=10) mice were randomly grouped and then subjected to right knee DMM surgery. The mice were sacrificed by cervical dislocation 4 and 8 weeks after surgery. The WT (C57BL/6J, n=10) and SCD1-KO (C57BL/6J, n=10) mice were randomly grouped and not specially treated. The mice were sacrificed by cervical dislocation at 6 months of age. The knees were dissected and immediately immersed in paraformaldehyde or temporarily frozen at −80 ℃ before further processing. A protocol was prepared before the study without registration.
Histology and immunohistochemistry
The human and mouse knee joints were fixed for 48 hours in 4% paraformaldehyde, decalcified for 21 days in 20% ethylenediaminetetraacetic acid (EDTA), embedded in paraffin, and sectioned at 5 µm thickness. The sections were stained using Safranin O/Fast Green and hematoxylin and eosin (HE) staining procedures. The histological scoring method of the Osteoarthritis Research Society International (OARSI) was used to quantify the degree of cartilage degeneration (20). Immunohistochemistry was carried out in accordance with the manufacturer’s instructions (SP-9000 ZSGB-BIO). The sections were treated for 10 minutes with 3% H2O2 to prevent endogenous peroxides. The goat serum was blocked for 1 hour to avoid nonspecific IgG binding. The sections were then incubated with the primary antibody overnight in a humidified atmosphere at 4 ℃. On the next day, the sections were treated for 30 minutes with biotin-associated linkers and then for 1 hour with streptavidin. We used 3,3’-Diaminobenzidine (DAB) as the substrate for the colorimetric detections.
Cell culture and transfection
Lipo8000TM Transfection Reagent was used for the transient transfection experiments, following the manufacturer’s recommended protocol (C0533; Beyotime, China). The ATDC5 chondrocytes were seeded in a six-well plate at a density of 2,000 cells/well for 24 hours before transfection. The transfection solution was prepared at a 25:1 ratio of small interfering ribonucleic acid (siRNA) (100 pmol) to Lipo8000TM (4 µL). The nucleic acid sequences of siRNA and negative control (NC) are shown in Table 1. The samples were incubated in 5% CO2 at 37 ℃ for 48 hours. The cultured cells were isolated and used to extract RNA and proteins.
Table 1
Sequence (5' to 3') | |
---|---|
siRNA-sense | CCGCGCAUCUCUAUGGAUAUCTT |
siRNA-antisense | GAUAUCCAUAGAGAUGCGCGGTT |
NC-sense | UUCUCCGAACGUGUCACGUTT |
NC-antisense | ACGUGACACGUUCGGAGAATT |
NC, negative control.
Chondrocyte oxygen stress and apoptosis assays
The ATDC5 chondrocytes were pretreated for 24 hours with tumor necrosis factor α (TNF-α) (50 ng/mL) or phosphate buffer saline (PBS), depending on the experimental design. Intracellular reactive oxygen species (ROS) levels were determined using a dihydroethidium (DHE) detection kit as specified by the manufacturer’s guidelines (50102ES02; Yeasen, China). To assess cell viability, cell death was determined by an Apoptosis-Hoechst staining kit (C0003; Beyotime, China) after incubation with TNF-α (50 ng/mL) or PBS for 24 hours.
Quantitative reverse transcription PCR (RT-qPCR)
Total RNA was isolated from the ATDC5 chondrocytes using Trizol Reagent (R0016; Beyotime), as directed by the manufacturer. A Prime Script RT Kit was used to reverse transcribe the whole RNA (D7178M; Beyotime). BeyoFastTM SYBR Green qPCR Mix (2X) (D7260; Beyotime) was used for the qPCR in a Light Cycle 480II Real-Time PCR System (Roche). Glyceraldehyde-phosphate dehydrogenase (GAPDH) served as the internal reference for normalization, and the relative expression was calculated using the 2−(ΔΔCT) technique. The primer sequences for the qRT-PCR are shown in Table 2.
Table 2
Primer name | Sequence (5' to 3') |
---|---|
MMP13-F | TGTTTGCAGAGCACTACTTGAA |
MMP13-R | CAGTCACCTCTAAGCCAAAGAAA |
GPX4-F | TGTGCATCCCGCGATGATT |
GPX4-R | CCCTGTACTTATCCAGGCAGA |
SCD1-F | TTCTTGCGATACACTCTGGTGC |
SCD1-R | CGGGATTGAATGTTCTTGTCGT |
P53-F | CCCCTGTCATCTTTTGTCCCT |
P53-R | AGCTGGCAGAATAGCTTATTGAG |
GAPDH-F | TGTGTCCGTCGTGGATCTGA |
GAPDH-R | TTGCTGTTGAAGTCGCAGGAG |
qPCR, quantitative polymerase chain reaction.
Western Blot
A Protein Extraction Kit (P0013; Beyotime) was used to extract total protein from the cartilage and chondrocytes, which was then measured using the BCA Protein Assay Kit (P0010S; Beyotime). Sodium dodecyl sulfate-polyacrylamide gel electrophoresis (SDS-Page) was used to separate the protein samples on a polyvinylidene fluoride (PVDF) membrane. The blot was blocked in a 5% bovine serum albumin (BSA) solution for 1 hour at room temperature before being incubated overnight at 4 ℃ with an appropriate dilution of primary antibody. The membrane was then treated for 60 minutes at 37 ℃ with the appropriate secondary antibody. Finally, the enhanced chemiluminescence (ECL) method was applied to detect the protein of interest, and the signal intensity was detected under ECL chemiluminescence equipment.
Statistical analysis
All the data collected in this study were analyzed using GraphPad Prism 9 software (Version 9.3.1). Normally distributed measurement data are expressed as the mean ± standard deviation (SD), while non-normally distributed measurement data are expressed as the median (interquartile range), and the comparisons were examined by Student’s t-test or Mann-Whitney test (non-parametric distribution). The pretreatment core packages in R (Version 4.1.3, www.r-project.org) were used to normalize the data set and perform the GO analysis, while the GSEA analysis was undertaken with the GSEA app program (Version 4.2.3). A P value <0.05 was considered statistically significant (*, P<0.05, **, P<0.01).
Results
Unsaturated fatty acid metabolism is reduced in osteoarthritic chondrocytes
Abnormal energy metabolism was recently proposed to be correlated with the development of OA (21). To investigate whether metabolic genes were differentially expressed in OA, we downloaded the GSE176199 dataset, which included three normal cartilage stromal cells, three osteoarthritic chondrocytes, and three osteoarthritic cartilage mesenchymal stromal cells. The RNAseq data of normal cartilage stromal cells and osteoarthritic chondrocytes were analyzed by GSEA. Interestingly, even though fatty acid metabolism was significantly enriched in osteoarthritic chondrocytes, unsaturated fatty acid metabolism was significantly inhibited (Figure 1A), which suggests that reduced unsaturated fatty acids might have a crucial role in OA progression. Next, we screened for genes that were significantly differentially expressed between osteoarthritic and normal chondrocytes (P<0.05, logFC >1) (Figure 1B) and performed a GO analysis for the up-regulated or down-regulated genes, respectively. A couple of the shared GO terms indicated that both up- and down-regulated genes were enriched in regulating the functions of cell membrane components (Figure 1C,1D). Thus, unsaturated fatty acid metabolism was inhibited in osteoarthritic chondrocytes.
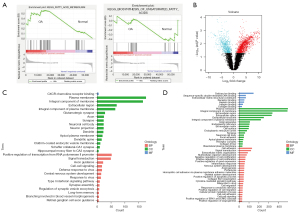
SCD1 expression is reduced in OA articular cartilage
Since PUFA could not be made in vertebrate cell lines, MUFA were synthesized to offset their PUFA deficiency (22). Based on the GSEA analysis, we hypothesized that MUFA biosynthesis was inhibited in OA. As SCD1 is an important rate-limiting enzyme in the anabolic process of MUFAs, the effect of SCD1 alterations in human OA articular cartilage was examined. When the cartilage specimens were stained with Safranin O/fast green and hematoxylin and eosin (HE) to determine the degree of deterioration, we found the superficial portion of normal articular cartilage was smooth, with just minor surface erosions. The superficial portion of OA cartilage, on the other hand, was cracked and fissured, with extensive fibrillation or cartilage erosion reaching down to the subchondral bone (Figure 2A). The Kellgren-Lawrence (K-L) OA stages were significantly higher than those of the controls (Figure 2B). Western blotting was used to compare SCD1 expression in OA cartilage versus normal cartilage (Figure 2C). SCD1 expression was found to be significantly lower in OA cartilage than in normal cartilage. We then performed surgical DMM on two-month-old wild-type C57BL6/J mice. As expected, the DMM operation significantly contributed to the progressive weakening and degradation of the articular cartilage compared with the sham surgery (Figure 2D). The maximal OARSI score of either articular surface showed that cartilage destruction was more severe in DMM mice (Figure 2E). Four weeks after DMM, the steady decrease of SCD1 protein in the articular cartilage was confirmed by Western blot assays (Figure 2F). As a result, we concluded that SCD1 expression is reduced in OA articular cartilage.
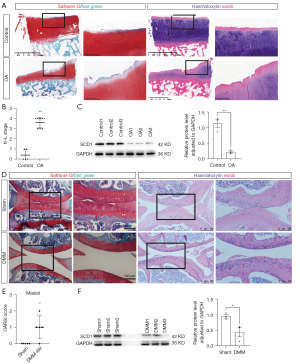
SCD1 deficiency in mouse chondrocytes accelerates cartilage degeneration after injury
To determine whether SCD1 regulates the expression of essential proteins associated with cartilage protection or injury, we generated and subjected the SCD1-KO mice to DMM. The efficiency of SCD1 knockdown in knee cartilage was verified by Western blot (Figure 3A). There were no significant abnormalities in the mice on joint X-ray examination at 6 months of age (Figure S1). In addition, by 6 months of age, SCD1-KO mice exhibited significantly increased expression of the chondrocyte hypertrophy marker, collagen type X (Col-X). In contrast, the expression of lubricin (a surface-active mucinous glycoprotein produced in the synovial joint that plays a crucial role in cartilage integrity) was significantly reduced in SCD1-KO mice compared with WT mice (Figure 3B). Quantification showed that SCD1-KO mice had a lower percentage of lubricin+ chondrocytes and a higher percentage of Col-X+ chondrocytes in both the upper and lower layers than wild-type mice (Figure 3C,3D). In addition, SCD1-KO mice had significantly more severe post-injury-induced cartilage damage and exhibited more severe signs of OA than WT mice at 4 and 8 weeks after DMM surgery (Figure 3E). The OARSI score of the tibia, femur or either articular surface confirmed that cartilage breakdown was more severe in SCD1-KO mice (Figure 3F). These histological changes in SCD1-KO animals reflected a transition from a homeostatic to a more degenerative state, as seen by the altered matrix and enhanced chondrocyte hypertrophy. Thus, these data suggested that SCD1 deficiency accelerated ECM degradation and the progression of OA after injury.
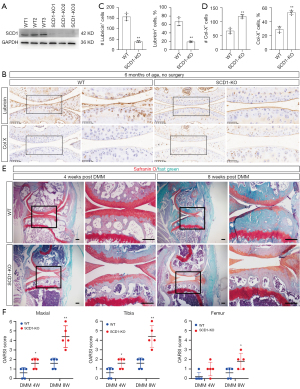
Ferroptosis in OA chondrocytes is regulated by SCD1 in vivo
The primary mechanism of ferroptosis is to induce lipid peroxidation of highly abundant PUFA on the cell membrane under the catalysis of ferrous iron or ester oxygenase. Previous GO analysis in osteoarthritic chondrocytes suggested that up- and down-regulated differentially expressed genes were enriched in the cell membrane component, leading us to speculate that chondrocytes may have undergone lipid peroxidation and ferroptosis in OA progression. To determine whether chondrocyte ferroptosis played a role in the progression of OA, we used immunofluorescence staining to look for the expression of glutathione peroxidase 4 (GPX4) (23), a crucial antioxidant enzyme and regulator of ferroptosis. The results showed that GPX4 expression was significantly lower in human OA cartilage than in normal tissues (Figure 4A,4B). In addition, P53 is another vital target protein for cell ferroptosis, which can potentiate ferroptosis by suppressing the transcription of system xc–subunit SLC7A11 (24). A significantly decreased expression of P53 was confirmed by immunohistochemical staining in the human OA cartilage specimens compared with the controls (Figure 4C,4D). To further verify the association between the deletion of SCD1 and ferroptosis in chondrocytes, we then looked at ferroptosis core regulator expression levels in mouse cartilage. The results showed that GPX4 expression was significantly down-regulated, and P53 expression was significantly elevated in the cartilage of SCD1-KO mice compared with WT mice 4 weeks after DMM surgery (Figure 4E-4G), which was similar to the human specimen results. Furthermore, as was typical of ferroptosis, shrunken mitochondria were seen by transmission electron microscopy (TEM) in the cartilage from SCD1-KO mice 4 weeks after DMM surgery (Figure 4H). In summary, the findings suggested that the deletion of SCD1 exacerbated chondrocyte ferroptosis in vivo.
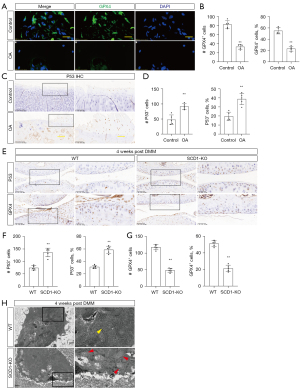
The SCD1-PPARG axis regulates chondrocyte ferroptosis
Peroxisome proliferator-activated receptors γ (PPARG) are ligand-activated nuclear receptors whose expression is directly regulated by long-chain fatty acids (LCFA) and SCD1 (25,26). PPARG deficiency in chondrocytes has previously been shown to lead to abnormal expression of OA-related metabolic and inflammatory genes (27,28). We hypothesized that PPARG was involved in the SCD1/ferroptosis regulatory pathway. To validate the importance of the SCD1-PPARG signaling axis in cartilage homeostasis regulation and determine if SCD1-PPARG could protect against OA-like pathologies in chondrocytes, we used immunohistochemistry to measure PPARG expression levels in SCD1-KO and WT mouse cartilage at 4 weeks after DMM surgery. The results suggested that PPARG expression was significantly lower in SCD1-KO than WT mouse cartilage (Figure 5A,5B). Furthermore, we constructed an OA model in ATDC5 chondrocytes stimulated with TNF-α, which are OA-associated pro-inflammatory cytokines (29). Significantly higher oxygen stress levels were detected by DHE assay (Figure 5C), and significantly increased apoptotic cells were detected by the apoptosis assay in the OA chondrocyte model compared with controls (Figure 5D). In addition, the expression of Mmp-13 mRNA was significantly increased in OA chondrocytes by qPCR analysis. In contrast, GPX4 and SCD1 gene expressions were significantly decreased, and these gene levels were consistent with the protein levels in our previous OA samples (Figure 5E). Next, we investigated the functions of SCD1 using siRNA knockdown in chondrocytes stimulated with TNF-α. Western blotting of SCD1, PPARG, and GPX4 protein expressions were performed and exhibited consistent trends (Figure 5F). The PPARG agonists reversed the reduction in PPARG and GPX4 in ATDC5 chondrocytes stimulated with siRNA (si-SCD1) (Figure 5G). Based on these findings, we propose that an SCD1-PPARG axis regulates ferroptosis in chondrocytes during OA progression.
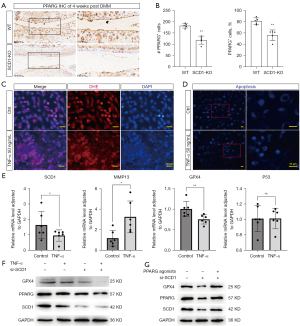
Discussion
In this study, we found reduced SCD1 expression in human OA articular cartilage. Additionally, we produced conclusive genetic evidence that postnatal SCD1 deletion in cartilage causes OA-like diseases and accelerates OA progression after DMM surgery. Furthermore, we found that the SCD1-PPARG axis disrupts articular cartilage homeostasis by inducing catabolic and ferroptosis-related gene expression in ATDC5 chondrocytes. Consequently, our findings provide the first genetic evidence linking lower SCD1 expression to articular cartilage deterioration in OA.
It is suggested that metabolic variables play a role in the complex pathophysiology of OA, resulting in joint degradation and failure (21). In clinical practice, OA frequently co-occurs with metabolic illnesses and comorbidities, such as diabetes, hyperlipidemia, and cardiovascular disease (30). Loef et al. recently discovered a connection between high plasma postprandial SFA and PUFA levels and clinical hand and structural knee OA (31). Lu et al. found that total high fat and SFA diets were associated with greater structural knee OA progression, but MUFA might prevent radiographic advancement, which suggests that MUFA has a protective effect on OA progression (32). In the present study, we found significantly decreased SCD1 in the upper articular chondrocytes of human OA specimens. Although there was no indication of spontaneous OA in SCD1-KO mice up to 6 months old, we found a significant increase in cartilage degeneration markers among articular chondrocytes in the SCD1-KO mice, with increased COL-X expression and decreased lubricin expression. Moreover, significantly aggravated cartilage damage in the SCD1-KO mice was also observed after DMM-induced knee instability. The natural course of OA was established in this SCD1-KO mouse model, supporting the hypothesis that SCD1 deficiency aggravates cartilage degeneration and weakens regenerative ability after cartilage injury. As a result, decreased MUFA uptake likely impacts the health of articular chondrocytes, making them sensitive to OA risk factors in the elderly or those with injuries. Future studies on MUFA metabolism in joint cartilage and the outcome of OA induced by aging or damage are recommended.
Ferroptosis is a newly discovered mode of cell death in many tissues, including articular cartilage. The induction of ferroptosis is linked to cell death and the pathophysiology of OA (33), and the inhibition of ferroptosis relieves the severity of experimentally induced OA (18). We showed that SCD1 deletion reduced the ferroptosis-related protein GPX4 and increased P53 protein in vivo and in vitro, respectively. However, there is no gold standard for detecting ferroptosis because the initial signals and molecular regulators of distinct forms of cell death are similar (12). In different diseases, GPX4 and P53 are both engaged in apoptosis and necrosis (34,35). In ATDC5 chondrocytes activated with TNF-α, we also found apoptosis and enhanced oxygen stress. In this study, we hypothesized that suppressing SCD1 changed the composition of membrane phospholipids, reducing the quantity of monounsaturated fatty acyl chains, increasing polyunsaturated fatty acyl chains, and decreasing membrane-localized antioxidants. This change in membrane composition increased the substrate for membrane lipid oxidation, making cells more vulnerable to ferroptosis inducers, which caused cell death by increasing lipid oxidation. Further studies of the regulatory networks that control ferroptosis via the SCD1 protein in the context of OA are warranted.
The precise mechanism for MUFA metabolism to maintain articular cartilage health is likely multi-faceted and requires additional investigation. Our findings show that cell survival and catabolic gene expression are both sensitive to changes in MUFA absorption. In addition to producing energy, MUFA can be nitrated to produce nitro-oleic acid. Nitro-oleic acid activates several signaling pathways, including those that have anti-inflammatory and antioxidant effects (36). Future research is needed to discover the specific metabolite pathways that connect poor MUFA metabolism to altered gene expression in OA articular cartilage.
There were several limitations in this study. First, this was an animal study with limited evidence for clinical practice. Second, the mechanism of SCD1 in regulating ferroptosis was not investigated. Thus, these results should be interpreted cautiously.
Conclusions
Deficiency of SCD1 is highly associated with OA by inducing ferroptosis in chondrocytes. The SCD1-PPARG axis is a potential target for the treatment of OA.
Acknowledgments
Funding: This work was supported by the National Natural Science Foundation of China (Grant No. 81902293).
Footnote
Reporting Checklist: The authors have completed the ARRIVE reporting checklist. Available at https://atm.amegroups.com/article/view/10.21037/atm-22-6630/rc
Data Sharing Statement: Available at https://atm.amegroups.com/article/view/10.21037/atm-22-6630/dss
Peer Review File: Available at https://atm.amegroups.com/article/view/10.21037/atm-22-6630/prf
Conflicts of Interest: All authors have completed the ICMJE uniform disclosure form (available at https://atm.amegroups.com/article/view/10.21037/atm-22-6630/coif). The authors have no conflicts of interest to declare.
Ethical Statement: The authors are accountable for all aspects of the work in ensuring that questions related to the accuracy or integrity of any part of the work are appropriately investigated and resolved.
Open Access Statement: This is an Open Access article distributed in accordance with the Creative Commons Attribution-NonCommercial-NoDerivs 4.0 International License (CC BY-NC-ND 4.0), which permits the non-commercial replication and distribution of the article with the strict proviso that no changes or edits are made and the original work is properly cited (including links to both the formal publication through the relevant DOI and the license). See: https://creativecommons.org/licenses/by-nc-nd/4.0/.
References
- Hunter DJ, Bierma-Zeinstra S. Osteoarthritis. Lancet 2019;393:1745-59. [Crossref] [PubMed]
- Loeser RF, Collins JA, Diekman BO. Ageing and the pathogenesis of osteoarthritis. Nat Rev Rheumatol 2016;12:412-20. [Crossref] [PubMed]
- Oppedisano F, Bulotta RM, Maiuolo J, et al. The Role of Nutraceuticals in Osteoarthritis Prevention and Treatment: Focus on n-3 PUFAs. Oxid Med Cell Longev 2021;2021:4878562. [Crossref] [PubMed]
- Wu CL, Jain D, McNeill JN, et al. Dietary fatty acid content regulates wound repair and the pathogenesis of osteoarthritis following joint injury. Ann Rheum Dis 2015;74:2076-83. [Crossref] [PubMed]
- ALJohani AM. Syed DN, Ntambi JM. Insights into Stearoyl-CoA Desaturase-1 Regulation of Systemic Metabolism. Trends Endocrinol Metab 2017;28:831-42. [Crossref] [PubMed]
- Peláez R, Pariente A, Pérez-Sala Á, et al. Sterculic Acid: The Mechanisms of Action beyond Stearoyl-CoA Desaturase Inhibition and Therapeutic Opportunities in Human Diseases. Cells 2020;9:140. [Crossref] [PubMed]
- Zhou D, Bao Q, Fu S. Anticancer activity of ursolic acid on retinoblastoma cells determined by bioinformatics analysis and validation. Ann Transl Med 2021;9:1548. [Crossref] [PubMed]
- Tesfay L, Paul BT, Konstorum A, et al. Stearoyl-CoA Desaturase 1 Protects Ovarian Cancer Cells from Ferroptotic Cell Death. Cancer Res 2019;79:5355-66. [Crossref] [PubMed]
- Dziewulska A, Dobosz AM, Dobrzyn A, et al. SCD1 regulates the AMPK/SIRT1 pathway and histone acetylation through changes in adenine nucleotide metabolism in skeletal muscle. J Cell Physiol 2020;235:1129-40. [Crossref] [PubMed]
- Jiang X, Stockwell BR, Conrad M. Ferroptosis: mechanisms, biology and role in disease. Nat Rev Mol Cell Biol 2021;22:266-82. [Crossref] [PubMed]
- Chen X, Kang R, Kroemer G, et al. Broadening horizons: the role of ferroptosis in cancer. Nat Rev Clin Oncol 2021;18:280-96. [Crossref] [PubMed]
- Zheng J, Conrad M. The Metabolic Underpinnings of Ferroptosis. Cell Metab 2020;32:920-37. [Crossref] [PubMed]
- Jiang Y, Tuan RS. Origin and function of cartilage stem/progenitor cells in osteoarthritis. Nat Rev Rheumatol 2015;11:206-12. [Crossref] [PubMed]
- Zhang S, Xu J, Si H, et al. The Role Played by Ferroptosis in Osteoarthritis: Evidence Based on Iron Dyshomeostasis and Lipid Peroxidation. Antioxidants (Basel) 2022;11:1668. [Crossref] [PubMed]
- Burton LH, Radakovich LB, Marolf AJ, et al. Systemic iron overload exacerbates osteoarthritis in the strain 13 guinea pig. Osteoarthritis Cartilage 2020;28:1265-75. [Crossref] [PubMed]
- Yao X, Sun K, Yu S, et al. Chondrocyte ferroptosis contribute to the progression of osteoarthritis. J Orthop Translat 2021;27:33-43. [Crossref] [PubMed]
- Liu MY, Li HM, Wang XY, et al. TIGAR drives colorectal cancer ferroptosis resistance through ROS/AMPK/SCD1 pathway. Free Radic Biol Med 2022;182:219-31. [Crossref] [PubMed]
- Ye Z, Zhuo Q, Hu Q, et al. FBW7-NRA41-SCD1 axis synchronously regulates apoptosis and ferroptosis in pancreatic cancer cells. Redox Biol 2021;38:101807. [Crossref] [PubMed]
- Glasson SS, Blanchet TJ, Morris EA. The surgical destabilization of the medial meniscus (DMM) model of osteoarthritis in the 129/SvEv mouse. Osteoarthritis Cartilage 2007;15:1061-9. [Crossref] [PubMed]
- Glasson SS, Chambers MG, Van Den Berg WB, et al. The OARSI histopathology initiative–- recommendations for histological assessments of osteoarthritis in the mouse. Osteoarthritis Cartilage 2010;18:S17-23. [Crossref] [PubMed]
- Berenbaum F, Wallace IJ, Lieberman DE, et al. Modern-day environmental factors in the pathogenesis of osteoarthritis. Nat Rev Rheumatol 2018;14:674-81. [Crossref] [PubMed]
- Else PL. The highly unnatural fatty acid profile of cells in culture. Prog Lipid Res 2020;77:101017. [Crossref] [PubMed]
- Ursini F, Maiorino M. Lipid peroxidation and ferroptosis: The role of GSH and GPx4. Free Radic Biol Med 2020;152:175-85. [Crossref] [PubMed]
- Liu Y, Gu W. p53 in ferroptosis regulation: the new weapon for the old guardian. Cell Death Differ 2022;29:895-910. [Crossref] [PubMed]
- Nakamura MT, Yudell BE, Loor JJ. Regulation of energy metabolism by long-chain fatty acids. Prog Lipid Res 2014;53:124-44. [Crossref] [PubMed]
- Yao D, Luo J, He Q, et al. SCD1 Alters Long-Chain Fatty Acid (LCFA) Composition and Its Expression Is Directly Regulated by SREBP-1 and PPARγ 1 in Dairy Goat Mammary Cells. J Cell Physiol 2017;232:635-49. [Crossref] [PubMed]
- Vasheghani F, Zhang Y, Li YH, et al. PPARγ deficiency results in severe, accelerated osteoarthritis associated with aberrant mTOR signalling in the articular cartilage. Ann Rheum Dis 2015;74:569-78. [Crossref] [PubMed]
- Zhu X, Chen F, Lu K, et al. PPARγ preservation via promoter demethylation alleviates osteoarthritis in mice. Ann Rheum Dis 2019;78:1420-9. [Crossref] [PubMed]
- Choi WS, Lee G, Song WH, et al. The CH25H-CYP7B1-RORα axis of cholesterol metabolism regulates osteoarthritis. Nature 2019;566:254-8. [Crossref] [PubMed]
- Mandl LA. Osteoarthritis year in review 2018: clinical. Osteoarthritis Cartilage 2019;27:359-64. [Crossref] [PubMed]
- Loef M, Ioan-Facsinay A, Mook-Kanamori DO, et al. The association of plasma fatty acids with hand and knee osteoarthritis: the NEO study. Osteoarthritis Cartilage 2020;28:223-30. [Crossref] [PubMed]
- Lu B, Driban JB, Xu C, et al. Dietary Fat Intake and Radiographic Progression of Knee Osteoarthritis: Data From the Osteoarthritis Initiative. Arthritis Care Res (Hoboken) 2017;69:368-75. [Crossref] [PubMed]
- Miao Y, Chen Y, Xue F, et al. Contribution of ferroptosis and GPX’'s dual functions to osteoarthritis progression. eBioMedicine 2022;76:103847. [Crossref] [PubMed]
- Aubrey BJ, Kelly GL, Janic A, et al. How does p53 induce apoptosis and how does this relate to p53-mediated tumour suppression? Cell Death Differ 2018;25:104-13. [Crossref] [PubMed]
- Seibt TM, Proneth B, Conrad M. Role of GPX4 in ferroptosis and its pharmacological implication. Free Radic Biol Med 2019;133:144-52. [Crossref] [PubMed]
- Piccinin E, Cariello M, De Santis S, et al. Role of Oleic Acid in the Gut-Liver Axis: From Diet to the Regulation of Its Synthesis via Stearoyl-CoA Desaturase 1 (SCD1). Nutrients 2019;11:2283. [Crossref] [PubMed]
(English Language Editor: D. Fitzgerald)