Zhuang medicine Shuanglu Tongnao Compound Recipe treats stroke by affecting the intestinal flora regulated by the TLR4/NF-κB signaling pathway
Highlight box
Key findings
• The Shuanglu Tongnao Compound Recipe (SLTNCR) can alleviate the symptoms of ischemic stroke (IS), and the potential mechanism of its effect is to protect brain tissue by suppressing inflammation.
What is known and what is new?
• Traditional Chinese medicine has potential for the clinical treatment of ischemic stroke.
• SLTNCR can alter the structure and diversity of the bacterial community in IS.
What is the implication, and what should change now?
• This study confirmed for the first time that SLTNCR is effective for the treatment of IS, and thus, the potential of Zhuang Medicine should be further explored.
Introduction
Stroke is a highly prevalent disease of the central nervous system (CNS) worldwide. It is caused by local cerebral vascular embolism that leads to local brain tissue blood supply disorders, brain tissue ischemia, hypoxia, and irreversible pathological changes. Stroke patients are characterized by neurological impairment, dementia, and death (1,2). It is one of the leading causes of death and disability among adults in China (3,4). The incidence of stroke in adults is 1–2%; however, in people over 70 years of age, the incidence is about 10%. In China, the prevalence of stroke in people aged 35–74 years is about 0.9%, among which about 80% of patients have ischemic stroke (IS) (5). Due to the aging population, the incidence of stroke is increasing every year in China, and considering the large population, stroke has resulted in a huge medical burden on families and society. Elucidating the mechanism of stroke occurrence and progression is essential for effective prevention and treatment.
At present, thrombolysis, antiplatelet aggregation, and anticoagulation are still the main treatments for IS. Although these drugs can control the physical symptoms of patients and delay disease progression, improving neurological impairment and enhancing the quality of life of patients remains challenging. The intestinal flora plays a key role in human diseases and the maintenance of health and is also closely related to the brain. The specific metabolites, hormones, and immune factors secreted by intestinal flora are connected to the brain through the immune, endocrine, nervous, and metabolic systems. Previous studies have shown that intestinal flora is closely associated with stroke, and dysregulation of intestinal flora affects poststroke neuroinflammation and prognosis in experimental stroke models (6). Intestinal flora imbalance can increase the risk of stroke and reduce the quality of life of patients, leading to a heavy burden on families and society (7). Nuclear factor-κB (NF-κB) and toll-like receptor 4 (TLR4) are two important cytokines that are involved in the inflammatory response of the CNS. TLR4 can regulate the inflammatory response and promote the release of inflammatory mediators, and NF-κB is an important transcription factor of the TLR4 reaction pathway, which can accelerate the secretion of inflammatory cells. Animal experimental studies have shown that the TLR4/NF-κB signaling pathway is associated with the occurrence and development of acute IS (8,9).
In recent years, the therapeutic effect of traditional Chinese medicine on a variety of diseases has been widely recognized, and its potential in clinical treatment is well known. Zhuang medicine has good treatment efficiency in herpes zoster (10), arthritis (11), psoriasis vulgaris (12), sciatica (13), diabetic gastroparesis (14), and rheumatoid arthritis (15). Moreover, Zhuang medicine exerts a remarkable effect in the treatment of stroke. However, a review of the current research shows that there are few studies on the therapeutic mechanism of Zhuang medicine, and there are still many problems to be addressed. The present study is based on the TLR4/NF-κB pathway and intestinal flora and explores the mechanism of Zhuang medicine Shuanglu Tongnao Compound Recipe (SLTNCR) in treating stroke. We present the following article in accordance with the ARRIVE reporting checklist (available at https://atm.amegroups.com/article/view/10.21037/atm-23-253/rc).
Methods
Animal and drug treatment
Fifty male rats (200±20 g) were purchased from Hunan Slyke Jingda Laboratory Animal Co. Ltd., China. Before the experiment, the animals were housed in a specified pathogen free (SPF) animal room and fed adaptively for 1 week. Experiments were performed under a project license (No. DW20200415-53) granted by the Ethics Committee of the Guangxi University of Traditional Chinese Medicine, in compliance with institutional guidelines for the care and use of animals. A protocol was prepared before the study without registration. The Zhuang medicine SLTNCR was composed of the following ingredients: wintercreeper 20 g, Cassia twig tips 15 g, rhizoma atractylodis 15 g, Rhizoma Pinelliae Preparata 20 g, Poria cocos 15 g, fructus crataegi cuneatae 20 g, pseudo-ginseng 15 g, pericarpium citri reticulatae 15 g, polygala fallax hemsl. 15 g, cistanche 15 g, Semen Cannabis 15 g, fresh ginger 15 g, and honey-fried licorice root 5 g.
The 50 rats were randomly divided into 7 groups: Sham, Injury, Injury + low dose SLTNCR, Injury + middle dose SLTNCR, Injury + high dose SLTNCR groups, Injury + Clostridium butyricum (CB) and Injury + Edaravone groups (n=10 rats per group).
Sham group: an equal volume of normal saline was administered by gavage 30 min before model creation. One hour after awakening, the animals were given a volume of normal saline by gavage for 6 consecutive days, once in the morning and once in the afternoon.
Control group: an equal volume of normal saline was administered by gavage 30 min before model creation. The IS model was successfully established. One hour after awakening, the animals were given a volume of normal saline by gavage for 6 consecutive days, once in the morning and once in the afternoon.
The dosage of SLTNCR was determined according to our preliminary results. Injury + SLTNCR group (low dose: 7.16 g·kg−1, middle dose: 14.33 g·kg−1, and high dose: 28.66 g·kg−1): 30 min before making the model, different doses of SLTNCR were administered by gavage.
2,3,5-triphenyltetrazolium chloride solution (TTC) staining
After anesthesia, the rats were decapitated and their brains were removed, washed with 0.9% sodium chloride, and placed into brain molds. Six 2 mm brain slices were cut isometrically in the coronal view from the optic chiasm. We then placed the brain slices in 2,3,5-triphenyltetrazolium chloride solution (1%, TTC) and incubated them for 15–20 min at 37 ℃ in the dark. The slices were turned over once midway through to ensure uniform staining. The tissue was then fixed in 4% paraformaldehyde and photographed. Analysis of the staining results: red was normal brain tissue and white was the infarcted part. Image J software (National Institutes of Health, USA) was used to measure and calculate the infarct rate: infarcted brain tissue area/contralateral cerebral hemisphere area × 100%. Photographs were taken and the hemispheres of each slice were measured. Cerebral edema was quantified as described by the relative increase in the area of the cerebral hemisphere on the ischemic side compared with the non-ischemic side. The relative cerebral edema ratio = sum of the ischemic side area/sum of the non-ischemic side area.
Detection of water content in brain tissue
The rats were decapitated and their brains were removed after anesthesia. The brain slices were cut isometrically in the coronal view from the optic chiasm. The relative cerebral edema ratio = sum of the ischemic side area/sum of the non-ischemic side area.
Hematoxylin-eosin (HE) staining
The slices were then placed into xylene I (20 min), xylene II (20 min), ethyl alcohol I (5 min), ethyl alcohol II (5 min), and 75% alcohol (5 min), and were then incubated with Hematoxylin dye solution for 3–5 min after washing with tap water. Next, the slices were incubated with 85% alcohol and 95% alcohol and dehydrated for 5 min, respectively, and then incubated with Eosin dye solution and stained for 5 min. After dehydrating with anhydrous alcohol and incubating with xylene, the slices were stored using Permount TM Mounting Medium (Ebioscience, USA)
Neurological score
We tested the neurological function on the 6th day of drug administration. Zea Longa’s 5-level scoring method was used for scoring: Grade 1 (0 point), no signs of neurological deficit, animal behavior was normal; Grade 2 (1 point), unable to fully flex the contralateral forepaw; Grade 3 (2 points), turning to the paralyzed side; Grade 4 (3 points), falling to the paralyzed side; Grade 5 (4 points), unable to walk spontaneously, loss of consciousness. High scores indicated severe cerebral ischemia-reperfusion injury in rats.
Western blotting
The concentrations of protein samples extracted from brain tissue were calculated using a bicinchoninic acid (BCA) kit. Next, we initiated an sodium dodecyl sulphate (SDS) gel electrophoresis and incubated the samples with primary antibody [anti-Caspase-3 antibody, ab184787, abcam, UK], anti-NF-κB p65 antibody (ab239882, abcam), anti-TLR4 antibody (ab217274, abcam)], followed by incubation with the secondary antibody [peroxidase-conjugated goat anti-rabbit immunoglobulin G (IgG) (H+L), 1:10,000] (16).
Enzyme-linked immunosorbent assay (ELISA) assay
Following the administration of the final dose, blood was collected from the abdominal aortas of the rats. Centrifugation was then performed at 3,000 r/min for 10 min (radius of centrifugation: 8.58 cm). After collection, the serum was stored in the refrigerator at −80 ℃. We determined the concentration of protein samples according to the instructions of the ELISA assay kit package (Beyotime Biotechnology, China).
Terminal-deoxynucleoitidyl Transferase Mediated Nick End Labeling (TUNEL) staining
After fixation with formaldehyde, the brain tissue was embedded in paraffin and sectioned. The slices were gradually immersed in xylene, ethanol, Proteinase K, paraformaldehyde, Equilibrate Bretion Buffer, Terminal Deoxynucleotidyl Transferase (TdT) enzyme reaction solution, hydrogen peroxide, Streptavidin horse radish peroxidase (HRP) solution, and 3,3'-diaminobenzidine (DAB) solution. After hematoxylin counterstaining, the sections were dehydrated with alcohol and made transparent with xylene. Finally, the slices were sealed and examined under a microscope (Leica, Germany).
Transmission electron microscopy
The rats were decapitated and sacrificed 6 days after drug administration. The volume of the collected tissue generally did not exceed 1 mm × 1 mm × 1 mm. The specimens were quickly fixed using an electron microscopy fixator (Shanghai Macklin Biochemical Co., Ltd., China) at 4 ℃ for 2–4 h. We then rinsed them with 0.1 M phosphate buffer (PB, pH 7.4) three times (for 15 min each time). A 1% osmic acid PB (0.1 M, pH 7.4) was used to fix the samples for 2 h at room temperature (20 ℃). Next, we rinsed the samples with 0.1 M PB (pH 7.4) three times (for 15 min each time). After dehydration, immersion, embedding, and ultrathin sectioning, the sections were double-stained with uranium lead and dried overnight at room temperature.
Analysis of the gut microbiota
We extracted the genomic DNA from feces and detected the concentration and purity of DNA using agarose gel electrophoresis, with a sample concentration of 1 ng/μL. polymerase chain reaction (PCR) amplification was carried out on the 16S V4 region, and we detected the PCR product using agarose gel at a concentration of 2%. The same amount of samples were mixed based on the concentration of PCR product, and 1× PCR products were purified using agarose gel electrophoresis. Then the gel was cut and the target bands were recovered. Use the library building kit to build the library. Computer sequencing was performed after quantifying the constructed library through Qubit quantification and library detection. The original data obtained from sequencing were spliced to obtain valid data. Based on the valid data, UParse software (https://drive5.com/, USA) was used to cluster the sequences into operational taxonomic units (OTUs) with 97% consistency by default. The Mothur method and the SSUr RNA database of SILVA132 were applied for the species annotation analysis. The sample natural distribution, which reflected the similarity between samples, was depicted by Beta diversity analysis. Using LEfSe analysis, biomarkers were screened according to the linear discriminant analysis (LDA) effect size (LDA >2).
Statistical analysis
Statistical analysis of the experimental results, including the calculation of means and standard deviations (mean ± SD), was performed using ImageJ and GraphPad Prism 5.0. (GraphPad Software, USA) Differences between groups were considered significant when the P value was <0.05 using one-way analysis of variance (ANOVA) and Tukey’s test.
Results
Effects of SLTNCR on brain water content, neurological score, and infarct in stroke rats
Compared with the Sham group rats, the brain water content of the injured rats was significantly increased (P<0.001). Also, compared with the Injury group rats, the brain water content in the Injury + low, Injury + middle, Injury + high, Injury + Clostridium butyricum (CB), and Injury + Edaravone groups decreased significantly (P<0.001, Figure 1A).
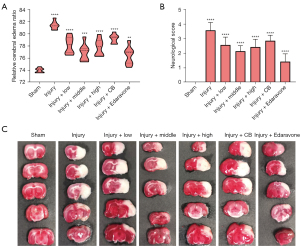
As shown in Figure 1B, compared with the Sham group rats, the neurological score for injured rats increased significantly (P<0.001). Moreover, compared with the Injury group, the neurological scores of the rats in the Injury + low, Injury + middle, Injury + high, Injury + CB, and Injury + Edaravone groups were significantly lower (P<0.05), which indicated that SLTNCR alleviated the brain injury of stroke rats.
TTC staining suggested that the areas of cerebral infarction in the Injury group rats were significantly larger than those in the Sham group. SLTNCR, CB, and Edaravone could significantly improve cerebral infarction after administration, which indicates that their administration has an obvious protective effect on the brain (Figure 1C).
Effects of SLTNCR on the physiological changes in stroke rats
As shown in Figure 2A-2D, the expressions of alanine transaminase (ALT), aspartate aminotransferase (AST), blood urea nitrogen (BUN), and serum creatinine (Scr) were not significantly different between the Injury and Sham groups, while significant decreases in the expressions of tumor necrosis factor (TNF-α) (P<0.01), lipopolysaccharides (LPS) (P<0.01), interleukin-6 (IL-6) (P<0.0001), and IL-1β (P<0.05) were observed in Injury group relative to the Sham group. Furthermore, compared with the Injury group, the administration of SLTNCR at low (P<0.01) and high (P<0.05) doses, and the administration of CB (P<0.01) and Edaravone (P<0.01), for 6 days significantly increased the expression of TNF-α. In addition, as shown in Figure 2E-2H, CB administration significantly increased the levels of LPS (P<0.05), IL-6 (P<0.01), and IL-1β (P<0.01); SLTNCR at high (P<0.05) and low (P<0.01) doses significantly elevated the level of IL-6; and Edaravone administration significantly increased the levels of IL-6 (P<0.0001) and IL-1β (P<0.01).
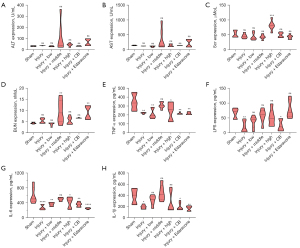
Effects of SLTNCR on the small intestine tissue-related indicators
To investigate the regulatory effect of SLTNCR on intestinal tissue, we evaluated the protein and messenger RNA (mRNA) (Figure 3A-3C) expression of the TLR4/NF-κB signal pathway [TLR4, NF-κB, phosphorylated (p)-NF-κB, inhibitor of nuclear factor kappa B (IκB), and phosphorylated-Inhibitor of nuclear factor kappa B (p-IκB) by western blot analysis], intestinal barrier function-related proteins [zona occludens 1 (ZO-1), occludin, claudin-5 by western blot analysis], and inflammation-related factors (TLR4, TNF-α, IL-6, and IL-1β by qPCR analysis). The expressions of TLR4, NF-κB, p-NF-κB, IκB, and p-IκB in the Injury group were significantly increased compared to those in the Sham group (P<0.0001), and the expressions of TLR4, NF-κB, IκB, p-NF-κB, and p-IκB were significantly decreased in the Injury + low, Injury + middle, and Injury + high groups. Only the expression level of NF-κB was significantly decreased in the Injury + CB and Injury + Edaravone groups (P<0.001), while that of the other proteins showed no significant difference in these two groups compared to the Injury group (Figure 3A).
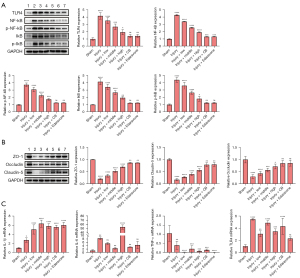
As shown in Figure 3B, the expressions of ZO-1, Occludin, and Claudin-5 were significantly decreased in the Injury group compared to those in the Sham group (P<0.0001) and were significantly increased in the Injury + low, Injury + middle, and Injury + high groups compared to the Injury group. Moreover, the expressions of ZO-1 and Claudin-5 were significantly increased in the Injury + CB and Injury + Edaravone groups (P<0.01). Compared with the Sham group, the expressions of TLR4 (P<0.0001), IL-6 (P<0.01), and IL-1β (P<0.05) were significantly increased, and the expression of TNF-α (P<0.01) was significantly decreased in the Injury group.
Compared with the injury group, the expression of IL-1β in the Injury + low, Injury + middle, Injury + high, Injury + CB, and Injury + Edaravone groups were significantly increased (P<0.0001). Also, the expression of IL-6 was significantly increased only in the Injury + high group (P<0.0001), while that of TNF-α was significantly decreased in the Injury + low (P<0.0001), Injury + middle (P<0.001), Injury + high (P<0.001), Injury + CB (P<0.001), and Injury + Edaravone (P<0.0001) groups. The expression of TLR4 in Injury + low (P<0.01), Injury + middle (P<0.001), Injury + high (P<0.001), and Injury + CB (P<0.0001) groups were significantly decreased.
Histological observation of the small intestine
The HE staining results indicated that the intestinal mucosal layers of rats in the Injury group were damaged to a certain extent compared with the Sham group; the villi were loosened or lost, and the submucosa, muscular layer, and serosal layer were involved, with local perforation or even necrosis. A significant improvement was observed following the administration of SLTNCR, CB, and Edaravone, which indicates that SLTNCR, CB, or Edaravone can protect the small intestine (Figure 4).
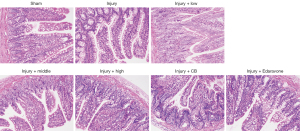
Histological observation of the brain and expression of apoptotic proteins and inflammatory factors
The HE staining results suggested that the number of neurons in the hippocampal CA1 area in the Injury group was significantly reduced compared with the Sham group. SLTNCR, CB, and Edaravone treatment significantly increased the number of neurons in the hippocampal CA1 area in stroke rats (Figure 5A). Moreover, the expressions of Bad (P<0.0001) and c-Caspase-3 (P<0.0001) were significantly increased, and Bcl-2 was significantly decreased (P<0.0001), in the Injury group compared with the Sham group. However, compared with the Injury group, except for the expression of Bcl-2 in the Injury + CB and Injury + Edaravone groups, there was a significant restorative effect in the Injury + low, Injury + middle, Injury + high, Injury + CB, and Injury + Edaravone groups (Figure 5B).
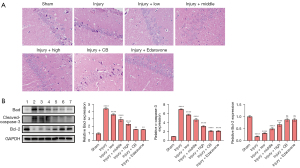
Analysis of gut microbiota
We acquired a total of 4,789,511 high-quality reads from all samples. The rarefaction curve revealed a sufficient sequencing depth (Figure 6A), and the species accumulation curve revealed a sufficient sample size (Figure 6B). Alpha diversity represented the species diversity and abundance in every sample. The Simpson, Chao1, goods coverage, Shannon, observed species, faith pd, and Pielou are the common indices. The Injury group exhibited significantly reduced chao1 (P<0.01), Shannon (P<0.01), Pielou (P<0.05), observed species (P<0.01), faith pd (P<0.001), and increased goods coverage (P<0.05) compared with the Sham group, indicating that the microbial communities were reduced in the Sham group. Faith pd (P<0.05) showed an increasing trend in the high-dose SLTNCR groups, while goods coverage (P<0.05) was lower than that in the Sham group (Figure 6C-6I).
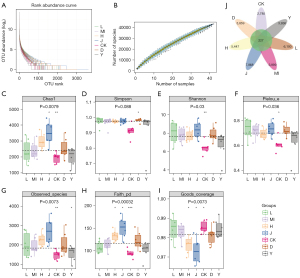
A total of 36,211 OTUs were also obtained (Figure 6J), and OPLS-DA, PCoA, and NMDS analyses were carried out (Figure 7A-7C). There were marked differences among the bacterial members between the Injury and Sham groups. We also observed notable similarities between the SLTNCR administration groups, indicating that SLTNCR induced significant restorative effects on disorderly gut microbiota in the stroke model. The bacterial community structures among each group at the phylum, class, order, family, and genus levels were shown in Figure 7D-7H. We performed a Lefse difference analysis to investigate the key gut microbiota in SLTNCR stroke treatment (Figure 8A). In the Sham group, the relative abundances of Ruminococcaceae, Oscillospira, Ruminococcus, Dokdonella, Desulfovibrionales, Dehalobacteriaceae, Leuconostocaceae, and Butyricicoccus were higher than those in the Sham group. Meanwhile, Lactobacillus and Eubacteriaceae showed greater enrichment in the Sham group. The relative abundances of Erysipelotrichales in the Injury + Edaravone group and Bacteroidales.S24_7 and Sphingomonas in the Injury + CB group were greater than those in the Injury group. Nine gut microbiota (Bacteroidetes, Bacteroides, Arthrobacter, Rikenellaceae, Lachnospira, Corynebacteriaceae, Paracoccus, Clostridiaceae.SMB53, and Planococcaceae) were identified as biomarkers of SLTNCR, and the results are presented in Table 1 and Figure 8B.
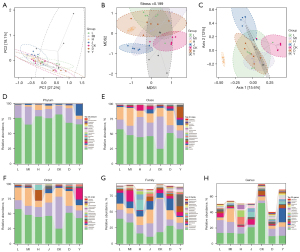
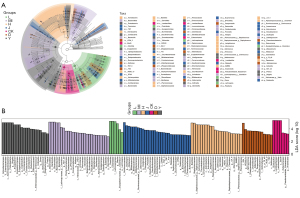
Table 1
Taxa | Abundance | Group | LDA score | P value |
---|---|---|---|---|
Bacteria.Firmicutes.Erysipelotrichi.Erysipelotrichales | 4.9723 | Y | 4.6483 | 0.0004 |
Bacteria.Bacteroidetes | 5.4768 | MI | 5.0756 | 0.0138 |
Bacteria.Bacteroidetes.Bacteroidia.Bacteroidales.Bacteroidaceae.Bacteroides | 5.2402 | MI | 4.9481 | 0.0051 |
Bacteria.Actinobacteria.Actinobacteria.Actinomycetales.Micrococcaceae.Arthrobacter | 2.7085 | MI | 3.3217 | 0.0034 |
Bacteria.Bacteroidetes.Bacteroidia.Bacteroidales.Rikenellaceae | 3.2535 | MI | 3.0192 | 0.0232 |
Bacteria.Firmicutes.Clostridia.Clostridiales.Lachnospiraceae.Lachnospira | 3.7712 | L | 3.4516 | 0.0047 |
Bacteria.Firmicutes.Clostridia.Clostridiales.Ruminococcaceae | 5.3369 | J | 5.0097 | 0.0012 |
Bacteria.Firmicutes.Clostridia.Clostridiales.Ruminococcaceae.Oscillospira | 4.8130 | J | 4.5116 | 0.0013 |
Bacteria.Firmicutes.Clostridia.Clostridiales.Ruminococcaceae.Ruminococcus | 4.4982 | J | 4.1808 | 0.0004 |
Bacteria.Proteobacteria.Gammaproteobacteria.Xanthomonadales.Xanthomonadaceae.Dokdonella | 1.9347 | J | 3.9823 | 0.0244 |
Bacteria.Proteobacteria.Deltaproteobacteria.Desulfovibrionales | 4.0413 | J | 3.7395 | 0.0069 |
Bacteria.Firmicutes.Clostridia.Clostridiales.Dehalobacteriaceae | 2.2937 | J | 3.5410 | 0.0355 |
Bacteria.Firmicutes.Bacilli.Lactobacillales.Leuconostocaceae | 2.9572 | J | 3.0791 | 0.0016 |
Bacteria.Firmicutes.Clostridia.Clostridiales.Ruminococcaceae.Butyricicoccus | 2.9662 | J | 2.9515 | 0.0148 |
Bacteria.Actinobacteria.Actinobacteria.Actinomycetales.Corynebacteriaceae | 4.9165 | H | 4.6264 | 0.0014 |
Bacteria.Proteobacteria.Alphaproteobacteria.Rhodobacterales.Rhodobacteraceae.Paracoccus | 2.8053 | H | 3.5287 | 0.0115 |
Bacteria.Firmicutes.Clostridia.Clostridiales.Clostridiaceae.SMB53 | 3.3983 | H | 3.1995 | 0.0024 |
Bacteria.Firmicutes.Bacilli.Bacillales.Planococcaceae | 3.3486 | H | 3.1671 | 0.0047 |
Bacteria.Bacteroidetes.Bacteroidia.Bacteroidales.S24_7 | 5.2595 | D | 4.9041 | 0.0046 |
Bacteria.Proteobacteria.Alphaproteobacteria.Sphingomonadales.Sphingomonadaceae.Sphingomonas | 2.7429 | D | 3.6945 | 0.0215 |
Bacteria.Firmicutes.Bacilli.Lactobacillales.Lactobacillaceae.Lactobacillus | 5.7149 | CK | 5.3306 | 0.0159 |
Bacteria.Firmicutes.Clostridia.Clostridiales.Eubacteriaceae | 3.6764 | CK | 3.3537 | 0.0008 |
L, Injury + low; MI, Injury + middle; H, Injury + high; J, Sham; CK, Injury; D, Injury + CB; Y, Injury + Edaravone; LDA, linear discriminant analysis.
Discussion
As the second leading cause of death, stroke is characterized by high rates of incidence, mortality, disability, and recurrence (17). In China, the burden of stroke is severe, and it is a primary cause of disability and death in adults (18). In developed countries, the prevalence of stroke in adults is 1–2%, while that in people over 70 years old is about 10%. In China, the prevalence of stroke among people aged 35–74 years is about 0.9%, of which about 85% are IS, accounting for the main proportion of stroke cases (19). According to a report published in The Lancet magazine in 2016, about 24.2% of patients registered with IS in Asia die every year (20). Although the guidelines for IS are constantly updated and the treatment plan is becoming increasingly standardized, the aging population, obesity, and other cerebrovascular disease risk factors remain prominent. Furthermore, the incidence, mortality, and disability rates of IS are still increasing. IS has also become a public health problem in China due to its high morbidity, mortality, and medical burden.
Intestinal flora may be the entry point of IS treatment
In the past, the treatment of IS was mainly focused on neuroprotection or microcirculation improvement. Although animal experimental research has a certain efficacy, the efficacy after clinical research remains far lower than expected (21). An increasing amount of research suggests that intestinal flora is closely associated with brain diseases (22). Study has shown that the brain infarct volume could be reduced by 60% following inhibition of the intestinal flora, and its mechanism of action depends on the regulation of inflammatory cells. This reveals the existence of the “gut-brain axis” and the correlation between cerebral ischemia and intestinal flora, providing new ideas for the treatment of cerebral ischemia (23). Clarifying the correlation and causality between these systems is also an important direction for the future development of neuroscience. Definitive evidence has confirmed the existence of two-way communication (including neural, endocrine, and other channels) between the intestine and its microbiota and the brain, which is known as the bacterial gut-brain axis (24). The intestinal tract is known as the second brain of the human body. The symbiotic microorganisms in the human body are mainly bacteria, most of which are fixed in the intestinal tract, and are collectively referred to as “intestinal flora”. Study has shown that cerebral ischemia will lead to an imbalance of intestinal microbiota, increased intestinal permeability, damage to the intestinal barrier, and the promotion of intestinal microbiota displacement. Meanwhile, repairing the damaged intestine will also have a certain effect on improving the blood-brain barrier function and can promote the recovery of neurological function after stroke (2). The metabolites of intestinal flora, such as short-chain fatty acids, can enter the blood through the endocrine pathway and then enter the brain to regulate the function of brain neurons, microglia, and astrocytes (25). Endotoxins produced by intestinal flora, such as LPS, can (directly or by activating peripheral immune cells) induce neuroinflammation and then migrate to the brain. Additionally, short-chain fatty acids, a metabolite of intestinal flora, can enter the blood and act on the brain through the endocrine pathway, and can also stimulate intestinal immune cells to release related inflammatory factors to affect the brain (26).
Mechanism of the TLR4/NF-κB signaling pathway in the development of IS
Following IS, astrocytes and microglia are activated in succession, and together, they participate in the regulation of the neuroinflammatory response. Ischemia and hypoxia, inflammatory factors, and pathological products all stimulate and activate astrocytes. The activated astrocytes are hyperplastic and hypertrophic and express high levels of glial fibrillary acidic protein. The activated astrocytes also secrete a range of cytokines and other inflammatory factors, which leads to a breakdown of the blood-brain barrier, causing peripheral leukocytes to converge on the infarct core, and the release of inflammatory factors triggers a post-IS cascade of inflammatory responses, ultimately worsening the IS outcomes (27). The TLR4/NF-κB pathway, which is mainly located in neuroglia, including microglia, and astrocytes, is an important inflammation-related signaling pathway (28) and the first line of defense in the brain. TLR4 is a class of transmembrane pattern recognition receptors that are activated by endogenous damage-related molecular patterns released due to ischaemic injury or stress cells, causing neuronal necrosis via apoptosis (29). Under normal conditions, NF-κB is bound to the NF-κB inhibitor protein (IκB) in an inactive form. The activation of IκB-activated kinase complexes phosphorylates and ubiquitinates it and binds to NF-κB in target genes in the nucleus, increasing the expression of NF-κB and initiating the downstream expression of large amounts of inflammatory factors, thereby exacerbating post-ischaemic brain tissue injury (30). In mice with TLR4 knockdown, it was found that IS damage was reduced via the low induction of TLR4 after IS (31), which suggests that modulating the TLR4/NF-κB signaling pathway to reduce neuroinflammatory responses and mitigate post-ischaemic brain tissue damage is important for the treatment of IS and improving its prognosis.
The TLR4/NF-κB signaling pathway mediates the mechanism of action of intestinal flora in IS
The role of gut flora in the pathogenesis of IS involves an inflammatory cascade in the brain. The gut and brain are regulated by bidirectional signaling via neurological, endocrine, and immune pathways, forming a “gut-brain axis” that is involved in the development of stroke (19). Microglia can be activated by amyloid, which is secreted by the intestinal flora, and the continued activation of microglia can further activate astroglial glia. Neuroinflammatory responses expressed by microglia, astrocytes, and complement activation near amyloid plaques are increased in the blood and cerebrospinal fluid of patients with IS (32). A previous study demonstrated that the intestinal flora reduces neuroinflammation by acting on astrocyte and microglia activity (33). LPS are the main metabolite of intestinal flora, which affect the intestinal epithelial function and increase intestinal permeability by mediating the TLR4/NF-κB signaling pathway. When intestinal permeability is increased, LPS and other flora metabolites can enter the bloodstream. Harmful substances in the bloodstream can easily enter the brain tissue and increase neuronal damage to brain cells (34). Short-chain fatty acids are products of bacterial fermentation and include isobutyric acid, isovaleric acid, butyric acid, acetic acid, propionic acid, and formic acid. This change was independent of age, type 2 diabetes, and hypertension (35). In addition, the imbalance of intestinal flora leads to an increased risk factor for stroke, which indirectly leads to stroke. Risk factors for stroke, such as hypertension, obesity, vascular dysfunction such as atherosclerosis, and aging, are linked to gut flora, which increases the risk of stroke.
Short-chain fatty acids can bind to receptors associated with intestinal epithelial cells, thereby activating downstream signaling pathways that act accordingly, such as their role in leading to further NF-κB signaling cascades (36). The high risk of IS is associated with an increased load of pathogenic bacteria in the gut and reduced levels of butyric acid-producing bacteria. Both the transplantation of short-chain fatty acid-rich donor rat feces into antibiotic-rejecting enteric bacteria recipient mice and the supplementation of IS rats with butyric acid are effective in improving neurological deficits in IS rats, and thus, have a therapeutic effect on IS. The results of this study show that the treatment of IS can be achieved by transplanting fecal matter into IS recipient mice. Correlation analysis showed that the increase in plasma LPS, TNF-α, NF-κB, and IL-6 after IS was consistent with an overgrowth of the Mycobacterium phylum (37). In future studies, we will use inhibitors of these pathways to further determine the role of these pathways in stroke.
Conclusions
In conclusion, the altered composition of the gut microbiota after stroke, local accumulation of harmful metabolites due to reduced gastrointestinal motility, and the hyperinflammatory response can all affect the function of the intestinal barrier, resulting in damage to the intestinal mucosa. The Zhuang medicine SLTNCR is commonly used clinically in the hospital; our findings indicate that SLTNCR plays a role in cerebral protection and enteroprotection by regulating the intestinal flora and the TLR4/NF-κB signaling pathway, and never treat stroke.
Acknowledgments
Funding: This study was supported by the Guangxi Natural Science Foundation (No. 2022GXNSFBA035576), the Science and Technology Development Plan Project of Liangqing District (No. 202220), the Guangxi Clinical Research Center for Encephalopathy of Traditional Chinese Medicine (No. AD20238028), the Innovation Team of Clinical Medicine Research and Application of Zhuang Medical Toxicities (No. 04B22058A3), the second batch of “Qihuang Project” High-level Talent Team Cultivation Project of Guangxi University of Traditional Chinese Medicine (No.2021008), the Guangxi Key Discipline of Traditional Chinese Medicine “Zhuang Medical Treatment” (No. GZXK-Z-20-62), the Key Laboratory of Research of Du-related Diseases in Zhuang Medicine (Guangxi University of Chinese Medicine), Education Department of Guangxi Zhuang Autonomous Religion (Gui Ke Jiao Yan[2022] No.10), and Guangxi International Zhuang Medicine Hospital “Green Seedling Project” Talent Cultivation Project.
Footnote
Reporting Checklist: The authors have completed the ARRIVE reporting checklist. Available at https://atm.amegroups.com/article/view/10.21037/atm-23-253/rc
Data Sharing Statement: Available at https://atm.amegroups.com/article/view/10.21037/atm-23-253/dss
Conflicts of Interest: All authors have completed the ICMJE uniform disclosure form (available at https://atm.amegroups.com/article/view/10.21037/atm-23-253/coif). The authors have no conflicts of interest to declare.
Ethical Statement: The authors are accountable for all aspects of the work in ensuring that questions related to the accuracy or integrity of any part of the work are appropriately investigated and resolved. Experiments were performed under a project license (No. DW20200415-53) granted by the Ethics Committee of the Guangxi University of Traditional Chinese Medicine, in compliance with institutional guidelines for the care and use of animals.
Open Access Statement: This is an Open Access article distributed in accordance with the Creative Commons Attribution-NonCommercial-NoDerivs 4.0 International License (CC BY-NC-ND 4.0), which permits the non-commercial replication and distribution of the article with the strict proviso that no changes or edits are made and the original work is properly cited (including links to both the formal publication through the relevant DOI and the license). See: https://creativecommons.org/licenses/by-nc-nd/4.0/.
References
- Lu Y, Zhou W, Lin Y, et al. The effects of traditional Chinese medicine sensory stimulation combined with transcranial direct current stimulation on deglutition and related complications in stroke patients with dysphagia: a randomized trial. Ann Palliat Med 2021;10:6597-605. [Crossref] [PubMed]
- Pluta R, Januszewski S, Czuczwar SJ. The Role of Gut Microbiota in an Ischemic Stroke. Int J Mol Sci 2021;22:915. [Crossref] [PubMed]
- Global, regional, and national burden of stroke and its risk factors, 1990-2019: a systematic analysis for the Global Burden of Disease Study 2019. Lancet Neurol 2021;20:795-820. [Crossref] [PubMed]
- Zhou M, Wang H, Zeng X, et al. Mortality, morbidity, and risk factors in China and its provinces, 1990-2017: a systematic analysis for the Global Burden of Disease Study 2017. Lancet 2019;394:1145-58. [Crossref] [PubMed]
- Benjamin EJ, Blaha MJ, Chiuve SE, et al. Heart Disease and Stroke Statistics-2017 Update: A Report From the American Heart Association. Circulation 2017;135:e146-e603. [Crossref] [PubMed]
- Singh V, Roth S, Llovera G, et al. Microbiota Dysbiosis Controls the Neuroinflammatory Response after Stroke. J Neurosci 2016;36:7428-40. [Crossref] [PubMed]
- Zhang J, Tang Q, Zhu L. Could the Gut Microbiota Serve as a Therapeutic Target in Ischemic Stroke? Evid Based Complement Alternat Med 2021;2021:1391384. [Crossref] [PubMed]
- Pan N, Lu L-y, Liu J, et al. Xyloketal B reduces cerebral infarction and neurologic deficits in a mouse transient middle cerebral artery occlusion model of stroke by suppressing the ROS/TLR4/NF-kappa B inflammatory signaling pathway. Acta Pharmacol Sin 2017;38:1069. [Crossref] [PubMed]
- Yao X, Sun C, Fan B, et al. Neurotropin exerts neuroprotective effects after spinal cord injury by inhibiting apoptosis and modulating cytokines. J Orthop Translat 2021;26:74-83. [Crossref] [PubMed]
- Jingjing L, Meikang L, Jie L, et al. Observation and Analysis of Clinical Efficacy of Zhuang Medicine Lotus Acupuncture Cupping Stasis Therapy on Patients with Postherpetic. Comb Chem High Throughput Screen 2022; Epub ahead of print. [Crossref]
- Yao L, Cheng S, Yang J, et al. Metabolomics reveals the intervention effect of Zhuang medicine Longzuantongbi granules on a collagen-induced arthritis rat model by using UPLC-MS/MS. J Ethnopharmacol 2022;294:115325. [Crossref] [PubMed]
- Pang YZ, Tang J, Zhang QH, et al. Treatment of Psoriasis Vulgaris with Medicated Thread Moxibustion of Zhuang Medicine: A Multicenter Randomized, Parallel Controlled Trial. Chin J Integr Med 2022;28:208-14. [Crossref] [PubMed]
- Tang H, Zheng J, Li T, et al. Effect of combined medicated thread moxibustion plus needle picking therapy of Zhuang nationality medicine on antioxidant levels in a rat model of sciatica. J Tradit Chin Med 2016;36:784-8. [Crossref] [PubMed]
- Zhang H, Miao FR, Luo YY, et al. Effect of moxibustion with ignited Zhuang-medicine medicated-thread on interstitial cells of Cajal in gastric antrum in diabetic gastroparesis rats. Zhen Ci Yan Jiu 2015;40:104-7, 118.
- Luo Y, Xu D, Cao Z, et al. Traditional therapies of Zhuang medicine improve pain and joint dysfunction of patients in rheumatoid arthritis: A protocol for systematic review and meta-analysis. Medicine (Baltimore) 2020;99:e22264. [Crossref] [PubMed]
- Wang T, Li B, Wang Z, et al. Sorafenib promotes sensory conduction function recovery via miR-142-3p/AC9/cAMP axis post dorsal column injury. Neuropharmacology 2019;148:347-57. [Crossref] [PubMed]
- Zerna C, Thomalla G, Campbell BCV, et al. Current practice and future directions in the diagnosis and acute treatment of ischaemic stroke. Lancet 2018;392:1247-56. [Crossref] [PubMed]
- Wardlaw JM, Bath PM. Stroke research in 2018: extended time windows, refined benefit, and lifestyle prevention targets. Lancet Neurol 2019;18:2-3. [Crossref] [PubMed]
- Global, regional, and national incidence, prevalence, and years lived with disability for 310 diseases and injuries, 1990-2015: a systematic analysis for the Global Burden of Disease Study 2015. Lancet 2016;388:1545-602. [Crossref] [PubMed]
- Kelly PJ, Albers GW, Chatzikonstantinou A, et al. Validation and comparison of imaging-based scores for prediction of early stroke risk after transient ischaemic attack: a pooled analysis of individual-patient data from cohort studies. Lancet Neurol 2016;15:1238-47. [Crossref] [PubMed]
- Oliveira FAA, Sampaio Rocha-Filho PA. Headaches Attributed to Ischemic Stroke and Transient Ischemic Attack. Headache 2019;59:469-76. [Crossref] [PubMed]
- Stefano GB, Pilonis N, Ptacek R, et al. Gut, Microbiome, and Brain Regulatory Axis: Relevance to Neurodegenerative and Psychiatric Disorders. Cell Mol Neurobiol 2018;38:1197-206. [Crossref] [PubMed]
- Benakis C, Brea D, Caballero S, et al. Commensal microbiota affects ischemic stroke outcome by regulating intestinal γδ T cells. Nat Med 2016;22:516-23. [Crossref] [PubMed]
- Cox LM, Weiner HL. Microbiota Signaling Pathways that Influence Neurologic Disease. Neurotherapeutics 2018;15:135-45. [Crossref] [PubMed]
- Ristori MV, Quagliariello A, Reddel S, et al. Autism, Gastrointestinal Symptoms and Modulation of Gut Microbiota by Nutritional Interventions. Nutrients 2019;11:2812. [Crossref] [PubMed]
- Li M, van Esch BCAM, Wagenaar GTM, et al. Pro- and anti-inflammatory effects of short chain fatty acids on immune and endothelial cells. Eur J Pharmacol 2018;831:52-9. [Crossref] [PubMed]
- Liu M, Xu Z, Wang L, et al. Cottonseed oil alleviates ischemic stroke injury by inhibiting the inflammatory activation of microglia and astrocyte. J Neuroinflammation 2020;17:270. [Crossref] [PubMed]
- Li Y, Liang W, Guo C, et al. Renshen Shouwu extract enhances neurogenesis and angiogenesis via inhibition of TLR4/NF-κB/NLRP3 signaling pathway following ischemic stroke in rats. J Ethnopharmacol. 2020;253:112616. [Crossref] [PubMed]
- Lyu Q, Pang X, Zhang Z, et al. Microglial V-set and immunoglobulin domain-containing 4 protects against ischemic stroke in mice by suppressing TLR4-regulated inflammatory response. Biochem Biophys Res Commun 2020;522:560-7. [Crossref] [PubMed]
- Huang D, Zhou J, Li W, et al. Casticin protected against neuronal injury and inhibited the TLR4/NF-κB pathway after middle cerebral artery occlusion in rats. Pharmacol Res Perspect 2021;9:e00752. [Crossref] [PubMed]
- Tang AT, Choi JP, Kotzin JJ, et al. Endothelial TLR4 and the microbiome drive cerebral cavernous malformations. Nature 2017;545:305-10. [Crossref] [PubMed]
- Keaney J, Campbell M. The dynamic blood-brain barrier. FEBS J 2015;282:4067-79. [Crossref] [PubMed]
- Xu S, Lu J, Shao A, et al. Glial Cells: Role of the Immune Response in Ischemic Stroke. Front Immunol 2020;11:294. [Crossref] [PubMed]
- Yan T, Nian T, Liao Z, et al. Antidepressant effects of a polysaccharide from okra (Abelmoschus esculentus (L) Moench) by anti-inflammation and rebalancing the gut microbiota. Int J Biol Macromol 2020;144:427-40. [Crossref] [PubMed]
- Vanegas SM, Meydani M, Barnett JB, et al. Substituting whole grains for refined grains in a 6-wk randomized trial has a modest effect on gut microbiota and immune and inflammatory markers of healthy adults. Am J Clin Nutr 2017;105:635-50. [Crossref] [PubMed]
- Kim YA, Keogh JB, Clifton PM. Probiotics, prebiotics, synbiotics and insulin sensitivity. Nutr Res Rev 2018;31:35-51. [Crossref] [PubMed]
- Al-Obaide MAI, Singh R, Datta P, et al. Gut Microbiota-Dependent Trimethylamine-N-oxide and Serum Biomarkers in Patients with T2DM and Advanced CKD. J Clin Med 2017;6:86. [Crossref] [PubMed]
(English Language Editor: A. Kassem)