Laxative effect of Wenyang Yiqi Decoction on loperamide-induced astriction model mice
Highlight box
Key findings
• WYD may reduce LIA by regulating intestinal hormones, increasing the number of interstitial cells (ICCs) of Cajal, or adjusting enteral block action.
What is known and what is new?
• LIA is associated with changes in intestinal hormone levels and abnormal expression of interstitial cells of cajal.
• WYD is a prescription under the guidance of traditional Chinese medicine theory, which is effective in clinical treatment of constipation. This experiment has verified that WYD can regulate internal constipation, increasing the number of ICCs of Cajal, or adjusting central block action.
What is the implication, and what should change now?
• WYD is a traditional Chinese medicine compound, and its specific drugs and the relationship between them need to be further studied.
Introduction
Astriction is a universal, limited, common disease with complex causes. Some cases may be primary (idiopathic or functional), and some may be related to many diseases or drugs (1,2). The median prevalence of astriction among all adults is 16%; the incidence is higher among older adults (33.5% among adults aged 60–101 years) (3,4), and the median prevalence ratio value of women to men is 1.5:1 (5).
Astriction is a blockage or obstruction of the bowel. The main causes of ileus are: (I) common intestinal masses blocking the lumen, leading to ileus; (II) the consumption of persimmon which is not easy to digest. If the patient eats multiple persimmons, they may form a phytobezoar, thus blocking the intestinal cavity and resulting in ileus. If the patient has ileus, they will experience pain alongside other potential symptoms such as celialgia, vomiting, bloating, and flatulence. If the patient has severe ileus, their abdominal distension and trapped gas will be more serious, and the release of wind and fecal matter through the anus will cease. The diagnosis of astriction is among the 5 most common diagnoses of the gastrointestinal (GI) tract among outpatients in the United States (6), where the direct medical costs of astriction are estimated to exceed $230 million annually (7). The medical costs for women with astriction are twice as high as those for women without astriction (8). Patients with astriction experience different degrees of mental health problems and social dysfunction (9). For example, a study reported that health scores in astriction patients are similar to those of patients with gastroesophageal reflux disease and depression (10).
Slow-transit astriction (STA) is a more common type of astriction that is characterized by a notable prolongation in enteral transit time (11). STA is also a symptom caused by a variety of diseases. Symptoms generally include the prolonged passage of food through the GI tract, or large intestinal dysfunction characterized by no obvious organic lesions found in the colon and rectum, or a prolonged bowel movement cycle and difficulty due to conduction disorders. By adjusting GI hormones and promoting intestinal peristalsis, traditional Chinese medicine (TCM) pharmacology has revealed great potential in the therapy of STA (12). Nonetheless, to date, the etiology of slow transit constipation (STC) remains unclear, and it is difficult to cure. In modern medicine, surgical methods such as colectomy or subtotal excision are often used, but only a few patients truly require surgical treatment for chronic constipation. This requires strict control of surgical indications, but the long-term results are not very satisfactory. Some studies have shown that STA can be affected by long-term poor lifestyle habits. Further, many studies have suggested that STC is related to the abnormal interstitial cells of Cajal (ICCs), unnatural enteral neurotransmitters, and enteral dysfunction (12-15). A variety of GI hormones, including excitatory and inhibitory transmitters, which work in harmony with each other to complete the physiological function of the gut, are produced in the GI tract (16,17). ICCs are pacemaker cells in the GI tract that generate and transmit slow waves, adjust neurotransmitters, and are involved in both the conversion of the signal of GI neurotransmitters and the adjustment of neurotransmitters (18,19). Tight junction (TJ) dysfunction in the enteral epithelium is closely related with enteral water metabolism, and the level of Claudin-1 is significantly elevated in astriction-predominant irritable bowel syndrome (IBS) patients (20). It is necessary to find an appropriate way to solve the problem of astriction.
With the growth of age, the body function manifests different degrees of decline: the spleen and kidneys gradually enter a state of deficiency, the vital energy (qi) and blood wane, and a variety of chronic diseases may occur, leading to the formation of constipation. TCM believes that constipation is closely related to the spleen and kidney function. The spleen is an important organ that plays a role in internal transportation and is thought to be influenced by emotions such as anger. When the body's functions are impaired, the spleen and stomach may need to work harder to provide qi and blood to the body. This can lead to a deficiency in middle warmer energy and a weakened state, which can manifest as astriction. If the middle warmer energy can be restored, then the qi and blood will become vigorous and unobstructed, and finally constipation will be resolved. The treatment of constipation with TCM has a long history and extensive clinical basis. Recently, investigation of the treatment of STC with TCM has gradually increased (12,21). Wenyang Yiqi Decoction (WYD) is a classic formula of TCM, which is mainly used for facilitating blood circulation and eliminating stasis. WYD is a traditional Chinese medicine preparation made from Jichuan decoction. The key ingredients in this prescription are rougui and roucongrong. The decoction is used to warm the kidney yang, moisten the intestines, and relieve constipation. It is used in conjunction with Wuyao, which helps promote warming, and Huangqi, which greatly replenishes the qi of the spleen and lungs. This combination with Shengbaizhu also helps increase the power to move the spleen. With the addition of Danggui, Niuxi and Shengdihuang, the decoction has the added effect of nourishing blood, moistening dryness, nourishing the kidney and replenishing fluids, and guiding the stool down. The inclusion of benevolent drugs further strengthens the decoction’s ability to moisten the intestines. However, few studies have reported on the treatment of astriction by WYD and its related mechanisms. Based on this, this paper aimed to explore the effects of WYD on loperamide-induced astriction (LIA) and to reveal its possible therapeutic mechanism. We present the following article in accordance with the ARRIVE reporting checklist (available at https://atm.amegroups.com/article/view/10.21037/atm-23-6/rc).
Methods
Animals
We purchased 4-week-old BALB/c male mice (n=60) [certificate number: 20170005058255; tentative animal use license: SYXK (Su) 2017-0040] from Shanghai Slack Laboratory Animal Co., Ltd. (Shanghai, China). The mice were fed standard chow for 1 week in a rearing environment with a room temperature of 20–26 ℃, 40–70% relative humidity (the difference of the daily temperature ≤4 ℃), and artificial lighting with 12 hours of alternating light and dark (lights on at 07:00, lights off at 19:00). A protocol was prepared before the study without registration. Animal experiments were performed under a project license (No. 2021-009) granted by the Ethics Committee of Suzhou TCM Hospital Affiliated to Nanjing University of Chinese Medicine, in accordance with the Guide for the Care and Use of Laboratory Animals of Institutes of Health (Eighth Edition).
Preparation of TCM
All herbs were purchased from Changxing Hospital of Traditional Chinese Medicine, WYD was prepared as follows: a mixture of herbs (157 g) comprising 6 g Rougui (Cortex cinnamomic), 15 g Roucongrong (Herba cistanche), 10 g Niuxi (Radix achyranthis bidentatae), 20 g Huangqi (Astragalus membranaceus), 6 g Wuyao (Radix linderae), 30 g Shengbaizhu (Rhizoma atractyloids macrocephalae), 10 g Shengma (Rhizoma cimicifugae), 15 g Zhishi (Fructus aurantia immaturus), 15 g Danggui (Angelica sinensis), 10 g Shengdihuang (Radix rhemanniae), 10 g Huomaren (Semen cannabis), and 10 g Taoren (Semen persicae) was boiled in 1,800 mL of distilled water, refluxed, and extracted. The liquid was kept at −4 ℃ until use.
Induction of astriction and tentative design
Loperamide (Xi’an Janssen Pharmaceutical Co., Ltd., Shanxi, China; 50 mg/kg weight) was used for induction modeling. Normal saline containing loperamide was administered by intragastric administration once daily for 5 days.
In our experiment, mice were randomly divided as follows: (I) control group (NC group, n=10): gavage with the same volume of physiological saline; (II) Lop + Saline groups (n=10): mice administered with the same volume of loperamide and physiological saline as the WYD-administered group; (III) Lop+different WYD-administered groups, including the Lop + WYD-L (n=10), Lop + WYD-M (n=10), and Lop + WYD-H (n=10) groups: mice were first administered with Lop and 1 hour later administered with 10.2, 20.4, or 40.8 g/kg body weight (BW) of WYD once a day for 5 days; (IV) Lop + Prucalopride (Pru) groups (n=10): mice were first administered with Lop and 1 hour later administered with 0.26 mg/kg BW of Pru once a day for 5 days. Then, 5 days after WYD therapy, all animals were killed, and tissue patterns were obtained and reposited in Eppendorf tubes at −80 ℃ until assayed.
Body weight and fecal parameters
The BW of mice in every group was assessed throughout the experiment. Mice were weighed, and fecal pellets excreted from individual mice were collected on Day 6 of the experiment. The fecal number and fecal water (FW) content were determined. The moisture content calculation method was as follows: FW content = (fecal wet weight-fecal dry weight)/fecal wet weight ×100%.
Enteral transport function assay
On Day 6, we orally injected each mouse with 0.2 mL of Indian ink. After 20 minutes, the experimental mice were euthanized using carbon dioxide. Once the mice were dead, all the enteral segments (pylorus to anus) of the mice were opened and removed, and then the enteral pulsion rate of the mice was measured and calculated as (D) = the travel extent of the ink in the enteral (L2)/the total length of the enteral (L1) ×100%.
Enzyme-linked immunosorbent assay (ELISA)
Serum was not obtained from the mouse eyeballs, and the concentrations of relevant markers in mouse serum were concluded by the corresponding assay kits in line with the manufacturer’s explanations until the mice were dead. We purchased ELISA kits for gastrin (Gas; #CSB-E12924m), AChE (#CSB-E17521m), Substance P (SP; #CSB-E08359m), and Vasoactive Intestinal Peptide (VIP; #CSB-E08356m) from CUSABIO Co., Ltd. Mouse MTL ELISA Kit (YKW-20113) was obtained from Shanghai Youkewei Biotechnology Co., Ltd.
Colon histology assay
Fresh colon tissue was collected after the mice were sacrificed. Samples were processed according to histological methods. Sections were stained with hematoxylin and eosin (H&E) staining.
Quantitative real-time polymerase chain reaction (qRT-PCR)
TRIzol (Life Technologies Corp., Carlsbad, CA, USA) was used as the extraction reagent to extract total RNA from mouse colon tissues, and we performed reverse transcription in line with the kit explanations. SYBR Green (#RR820A) was used as a fluorescent marker; β-actin was used as the internal reference, and 1 µL of complementary DNA (cDNA) was added to the 20 µL reaction system. The amplification circumstances were 30 s at 95 ℃, 1 cycle, 95 ℃ for 5 s, 60 ℃ for 30 s, 40 cycles, 95 ℃ for 15 s, then 60 ℃ for 60 s, 1 cycle. We obtained the relative levels of messenger RNA (mRNA) using the 2−ΔΔCt method. The primer sequences are displayed in Table 1.
Table 1
Primordial name | Primordial consecutiveness (5'-3') |
---|---|
m-SCF-F | 5'-ATAGTGGATGACCTCGTGTTA-3' |
m-SCF-R | 5'-GAATCTTTCTCGGGACCTAAT-3' |
m-c-kit-F | 5'-CCGACGCAACTTCCTTATGAT-3' |
m-c-kit-R | 5'-TCAGGACCTTCAGTTCCGACA-3' |
m-ZO-1-F | 5'-TGGGAACAGCACACAGTGAC-3'; |
m-ZO-1-R | 5'-GCTGGCCCTCCTTTAACAC-3' |
m-Cldn-1-F | 5'- CCTCCTGGGAGTGATAGCAAT-3' |
m-Cldn-1-R | 5'-GGCAACTAAAATAGC CAGACCT-3' |
m-Ocln-F | 5'-AAGAGTACATGGCTGCTGCT-3' |
m-Ocln-R | 5'-TTTGCCTCTGGAGAGAATTG-3' |
m-β-actin-F | 5'-CTGTGCCCATCTACGAGGGCTAT-3' |
m-β-actin-R | 5'-TTTGATGTCACGCACGATTTCC-3' |
qRT-PCR, quantitative real-time polymerase chain reaction.
Immunohistochemical (IHC) assay
Paraffin sections were heated in a water bath for 15 minutes for antigen extraction until dewaxing and hydration. Fragments were cultivated with anti-ZO-1 (#sc-33725; Santa Cruz Biotechnology, Dallas, TX, USA), anti-occludin-1 (#71-1500, Invitrogen, Carlsbad, CA, USA), and anti-claudin-1 (#51-9000, Invitrogen, USA) at 4 °C for a whole night, followed by fluorescein isothiocyanate (FITC) goat anti-mouse (1:500, #115-095-062, Jackson ImmunoResearch, West Grove, PA, USA) at 37 ℃ for 1 hour. The cells were soaked in phosphate-buffered saline (PBS) 3 times. For counterstaining, 50–100 µL of prepared 4’,6-diamidino-2-phenylindole (DAPI) dye solution (#KGA215, Nanjing, China) was added dropwise to each slide and incubated at 25 ℃ for 5 minutes in the dark. The slides were mounted with anti-extraction sealing tape and photographed by laser confocal microscopy. Semiquantitative analysis of ZO-1, claudin-1, and occludin-1 protein utterance was performed using the integrated optical density (IOD) and Image-Pro Plus 6.0 graphic analysis software (Media Cybernetics, Rockville, MD, USA).
Western blot analysis
We selected 3 mice from each group, and the mouse tissues were added to the lysate and ground on ice to prepare a homogenate. The supernatant was collected for protein quantification by the bicinchoninic acid (BCA) method (#KGA902, Nanjing, China). Then, 20 µg/well of sample protein was taken from each group, separated by sodium dodecyl sulfate-polyacrylamide gel electrophoresis (SDS-PAGE; 80 V, 100 min), and then transferred to polyvinylidene fluoride (PVDF) membranes by the wet transfer method. The film was kept on a room temperature shaker and shaken in the blocking solution for 2 hours, and c-kit antibody (1:1,000, #14-1172-82, Thermo Fisher, Co., Ltd., Waltham, MA, USA)/SCF antibody (1:1,000, #26852-1-AP, Wuhan, China) was appended and blocked for a whole night at 4 ℃. After washing the membrane by shaking in tris-buffered saline (TBS), the medium antibody (1:10,000) was added and incubated at 25 ℃ for 1 hour in the dark. After washing the membrane, enhanced chemiluminescence (ECL) solution (#KGP116, Nanjing, China) was added and reacted for 3–5 minutes and the panniculus was disclosed to cinematograph for 5 minutes. The film was rinsed in developing solution and fixing solution, soaked in water, and finally air dried. Graphics were obtained and analyzed using a gel imager. Rabbit anti-GAPDH protein (#KGAA002, Nanjing, China) was used as an internal reference. Each experiment was repeated 3 times. Imaging was carried by the ChemiDoc MP Imaging System (Bio-Rad, Hercules, CA, USA), and the results were analyzed in grayscale using Gel-Pro32 software (Media Cybernetics, Marlow, UK) for image analysis.
Statistical analysis
GraphPad Prism 6.0 software (GraphPad Software, LA Jolla, CA, USA) was used to carry out statistical analysis, and the results are represented as the mean ± SEM. Data were statistically assessed by one-way analysis of variance (ANOVA) followed by Dunnett’s test for parabole between the NC group and multiple dose groups. The standard of significance was set at a P value of 0.05.
Results
Body weight and fecal pellet parameters
The condition of constipated mice was rated by the number of fecal pellets and FW content to determine the effect of WYD on the Lop-induced astriction (LIA) model mice. There were no significant differences in BW among mice in all experimental groups (Figure 1A), and changes in fecal pellet parameters were attained in all mouse groups to investigate the laxative effect of WYD on the astriction model mice. The data showed that the number of fecal pellets was significantly reduced in the Lop + Saline group compared with the No group (P<0.05). Whereas, in the Lop + WYD-M, Lop + WYD-H, Lop + Pru, the number of fecal pellets was comparatively significantly increased compared with Lop + Saline groups (P<0.05; Figure 1B). Compared with the No group, the total water content of fecal pellets gathered over 24 hours in the Lop + Saline group decreased by nearly 50% (P<0.05; Figure 1C). These results suggest that WYD treatment can alleviate symptoms in astriction model mice by increasing the number of fecal pellets and increasing FW content.
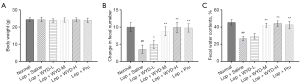
Enteral transport function
As shown in Figure 2A, compared with the NC group, the enteral transit ratio of the mice in the Lop + Saline groups was meaningfully lower (P<0.05). Compared with the Lop + Saline group, the enteral transit ratio of the Lop + WYD-M, Lop + WYD-H, and Lop + Pru groups was significantly accelerated (P<0.05). No significant difference between the Lop + WYD-H and Lop + Pru groups could be found (P>0.05).
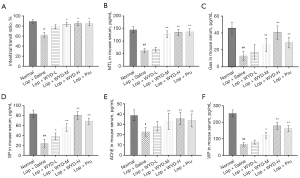
Parameters of serum
In this paper, ELISA was used to detect the changes of several serum elements connected with GI metabolism in serum of mice in each group, so as to evaluate the effects of WYD on serum biochemical components of mice with astriction. As shown in Figure 2B-2F, compared with the normal group, serum modulus were meaningfully shortened in the Lop + Saline groups (P<0.05). However, compared with Lop + Saline, these parameters, especially Gas, SP, and VIP, were elevated in a dose-dependent manner after WYD treatment (P<0.05; Figure 2C,2D,2F). Further, significant differences were observed between the Lop + WYD-H groups and the Lop + Pru groups in the Gas and VIP parameters (P<0.05, Figure 2C,2D). Therefore, these results suggest that the mechanism by which WYD alleviates LIA in model mice may be related to the elevation of serum factors related to GI motility.
Histological changes in the colon of LIA mice
Histological changes in the colons of mice with LIA in each group after WYD treatment were investigated by H&E staining (Figure 3). Compared with the NC group, mice treated with loperamide had loss of colonic epithelial cells, with crypt damage and partial loss of goblet cells. After WYD treatment, compared with that in the loperamide group, the destruction of epithelial cells improved, and goblet cells and epithelial cells were more intact in a dose-dependent manner.
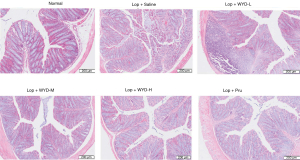
Effect of WYD on the levels of c-Kit and SCF in the colons of LIA mice
In this study, we investigated the effect of WYD on the levels of c-Kit and SCF genes and proteins in ICCs in the colon tissue of the astriction model mice. The c-Kit and SCF mRNA standards in the colon tissue of the Lop + Saline groups were significantly shortened (Figure 4A,4B), and WYD treatment significantly elevated c-Kit and SCF mRNA standards in a dose-dependent manner. Similar to the RT-PCR results, western blot analysis revealed that WYD also increased the expression of c-Kit and SCF (Figure 4C-4E). Notably, the expression of c-Kit in the Lop + WYD-H groups was upper than that in the Lop + Pru groups (P<0.05, Figure 4A,4D).
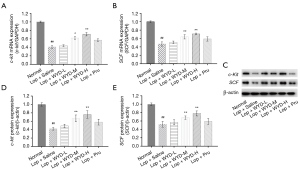
Effect of WYD on the expression levels of colonic epithelial TJ-related proteins in the colons of mice with LIA
To investigate whether WYD treatment affects the enteral barrier function of astriction model mice, the gene and protein standards of colonic epithelial TJ (Ocln, ZO-1, and Cldn-1) proteins were analyzed by qRT-PCR and immunohistochemistry.
The mRNA detection results of ZO-1, Cldn-1, and Ocln were basically consistent with the IHC detection results (Figure 5A-5D).The expression of ZO-1 was significantly upregulated after WYD intervention, and a significant difference was not found between the WYD-H group and the Lop + Pru groups (P<0.05, Figure 5A,5B). In addition, in terms of WYD’s downregulation of the expression of Cldn-1, we observed significantly differences in comparison with that in the Lop + Saline groups (P<0.05, Figure 5A,5C). In terms of the results of Ocln mRNA, no significant difference was observed between LIA and normal mice (P>0.05, Figure 5D). The results of IHC showed that the TJ proteins (ZO-1, Cldn-1) in the colon of the astriction model mice in the Lop + Saline groups had significant changes compared with the normal group (P<0.05; Figure 5A,5E,5F). In addition, immunohistochemical results showed that there was no significant difference in Ocln results between the LIA group and the normal group (P>0.05, Figure 5A,5G).
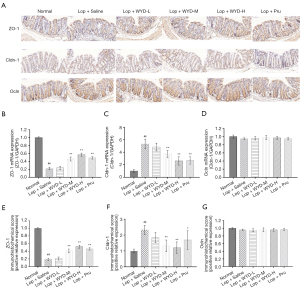
Discussion
STC is characterized by low stool volume, decreased frequency of bowel movements, and dry stools (22). The disease has a high incidence rate, severely influences the standard of life of those affected, and is a difficult clinical problem that urgently needs to be solved (1,2). STC can be caused by a variety of factors, including dietary habits (e.g., lack of fiber), excessive psychological stress, and use of drugs such as morphine (1). Traditional Chinese medicine dialectically treats constipation, which can regulate the yin and yang of the body, and treat constipation from a holistic perspective. However, there are many ingredients in traditional Chinese medicine, and it is difficult to explain the mechanism of constipation treatment clearly, and syndrome differentiation needs to be considered in the treatment of constipation.
WYD is a compound preparation of TCM and is clinically used to treat astriction. Nevertheless, the mechanism of its laxative effect still needs to be elucidated. Consequently, in our work, the laxative effect of WYD was investigated in a mouse model with LIA. We found that WYD alleviated loperamide-induced changes in fecal parameters (fecal number and FW content), enteral transport function, and histopathological changes in mice model with LIA. The results of ELISA showed that WYD modulates the production of GI metabolism-related modules in serum. Western blot analysis and qRT-PCR analysis were applied to reveal the expression levels of c-Kit and SCF. In addition, the expression levels of enteral epithelial TJs (Ocln, ZO-1, Cldn-1) are also detected by qRT-PCR and IHC. According to the results in our research, it can be known that WYD moderates LIA by boosting the criterion of ICCs markers (c-Kit and SCF), as well as regulating enteral barrier function.
Loperamide can be applied to opioid receptors in the intestine, and restrain the secretion of acetylcholine and prostaglandins, and can meaningfully restrain enteral peristalsis and secretion, prolonging the retention time of stool in the bowel and resulting in reduced or difficult bowel movements (22-25). The astriction model established by the loperamide gavage method has a shorter modeling time; the model animals have dry and hard stools, the quality and number of particles are reduced, and the enteral transit is slowed down (26). In this study, we used loperamide to induce astriction and observed that the LIA model mice developed symptoms similar to human astriction.
Fecal number, stool water content, and enteral transit ratio are considered important factors in evaluating astriction symptoms and drug efficacy. In our study, we did not observe any differences in the BW of mice between experimental groups, whereas fecal-related factors such as fecal number, stool water content, and enteral transit ratio were observed to be significantly reduced in mice following loperamide administration. WYD treatment significantly reduced astriction symptoms in model mice by increasing fecal number, FW content, and the enteral carriage rate in a dose-dependent manner.
A variety of GI hormones secreted by endocrine cells of the digestive tract are also involved in the regulation of colonic motility, which can significantly boost enteral motility (27-30). In this study, comparison with the normal group revealed that the ELISA expression levels of MTL, GAS, SP, Ache, and VIP in the model group were significantly decreased, and WYD could significantly raise the expression levels of these GI hormones in a dose-dependent fashion. These results state that WYD alleviates the symptoms of astriction in LIA model mice by increasing the standards of MTL, GAS, SP, AChE, and VIP in mouse serum.
ICCs partake in the GI elementary electrical rhythm governance and neurotransmitter signaling, which are main factors in the elementary functions of the GI meshwork (31). C-kit, as an ICC-specific bookmark, will bind to SCF to motivate lower signaling routes. This binding is crucial for the conservation of ICC development and phenotype (18,32). In this study, qRT-PCR and western blot methods were used to detect the mRNA and protein expression of SCF and c-kit in the colon tissue of mice in each group. The expression of SCF and c-kit in colon tissue decreased most obviously in the Lop + Saline groups, whereas WYD significantly increased their expression levels in a dose-dependent manner. Therefore, WYD improves astriction by restoring the SCF/c-kit signaling routes, boosting the figure of ICC cells, and regulating the contraction rhythm of colonic smooth muscle.
The enteral epithelial barrier is mainly composed of cell membranes and TJs, which close the spaces between adjacent cells (33). Increased enteral permeability has been reported to correlate with the pathophysiology of astriction-predominant irritable bowel syndrome (IBS-C) (34,35), but the relationship with STC is currently less reported. In this study, the histological changes in the colon of constipated mice suggested that loperamide-treated mice lost colonic epithelial cells, had damaged crypts, and lost some goblet cells (Figure 3). Therefore, we conducted an exploratory study on the TJs of the enteral epithelial barrier in a mouse model of astriction. These alterations in ZO-1, Cldn-1, and Ocln in the intestines of each group were analyzed by IHC and qRT-PCR. The results indicated that the expression of ZO-1 was meaningfully shortened in the Lop + Saline groups, and the expression of Cldn-1 was significantly increased. After WYD intervention, ZO-1 showed a rising trend, which was significantly different compared with that of Lop + Saline. Compared with Lop + Saline, the expression of Cldn-1 showed a significantly downward trend, but Ocln did not change significantly before and after treatment (Figure 5). Therefore, we believe that WYD treatment can increase the expression level of ZO-1 and decrease the level of Cldn-1 in the intestine, which may help regain loperamide-induced impairment of colonic barrier function, thereby increasing fecal excretion in mice.
Conclusions
Our study found that WYD can effectively relieve astriction in mice. The underlying mechanism likely involves the regulation of GI hormones. WYD also regulates smooth muscle contraction rhythm by increasing ICCs through the SCF/C-kit signaling routes. WYD can also regulate enteral TJ structure by increasing ZO-1 expression and decreasing Cldn-1 expression. The results of this study support the laxative effect of WYD in a mouse model of astriction. The next step in this vein of research would be to conduct clinical trials to estimate the efficacy and effectiveness of WYD in patients with astriction.
Acknowledgments
Funding: This work was supported by the National Natural Science Foundation of China (No. 82104858); Huzhou Science and Technology Bureau (No. 2018GY43); Suzhou Science and Technology Bureau (No. SYS2020187); Suzhou Gusu District Health Talent Project (No. GSWS2021046); and Suzhou Key Disease Diagnosis and Treatment Technology Project (No. LCZX202114); Natural Science Foundation of Jiangsu Province (No. BK20211085).
Footnote
Reporting Checklist: The authors have completed the ARRIVE reporting checklist. Available at https://atm.amegroups.com/article/view/10.21037/atm-23-6/rc
Data Sharing Statement: Available at https://atm.amegroups.com/article/view/10.21037/atm-23-6/dss
Peer Review File: Available at https://atm.amegroups.com/article/view/10.21037/atm-23-6/prf
Conflicts of Interest: All authors have completed the ICMJE uniform disclosure form (available at https://atm.amegroups.com/article/view/10.21037/atm-23-6/coif). The authors have no conflicts of interest to declare.
Ethical Statement: The authors are accountable for all aspects of the work in ensuring that questions related to the accuracy or integrity of any part of the work are appropriately investigated and resolved. Animal experiments were performed under a project license (No. 2021-009) granted by the Ethics Committee of Suzhou TCM Hospital Affiliated to Nanjing University of Chinese Medicine, in accordance with the Guide for the Care and Use of Laboratory Animals of Institutes of Health (Eighth Edition).
Open Access Statement: This is an Open Access article distributed in accordance with the Creative Commons Attribution-NonCommercial-NoDerivs 4.0 International License (CC BY-NC-ND 4.0), which permits the non-commercial replication and distribution of the article with the strict proviso that no changes or edits are made and the original work is properly cited (including links to both the formal publication through the relevant DOI and the license). See: https://creativecommons.org/licenses/by-nc-nd/4.0/.
References
- Shin JE, Park KS, Nam K. Chronic Functional Constipation. Korean J Gastroenterol 2019;73:92-8. [Crossref] [PubMed]
- Bharucha AE, Lacy BE. Mechanisms, Evaluation, and Management of Chronic Constipation. Gastroenterology 2020;158:1232-49.e3. [Crossref] [PubMed]
- Mugie SM, Benninga MA, Di Lorenzo C. Epidemiology of constipation in children and adults: a systematic review. Best Pract Res Clin Gastroenterol 2011;25:3-18. [Crossref] [PubMed]
- Bharucha AE, Locke GR, Zinsmeister AR, et al. Differences between painless and painful constipation among community women. Am J Gastroenterol 2006;101:604-12. [Crossref] [PubMed]
- Kinnunen O. Study of constipation in a geriatric hospital, day hospital, old people's home and at home. Aging (Milano) 1991;3:161-70. [Crossref] [PubMed]
- Shaheen NJ, Hansen RA, Morgan DR, et al. The burden of gastrointestinal and liver diseases, 2006. Am J Gastroenterol 2006;101:2128-38. [Crossref] [PubMed]
- Martin BC, Barghout V, Cerulli A. Direct medical costs of constipation in the United States. Manag Care Interface 2006;19:43-9.
- Choung RS, Branda ME, Chitkara D, et al. Longitudinal direct medical costs associated with constipation in women. Aliment Pharmacol Ther 2011;33:251-60. [Crossref] [PubMed]
- Belsey J, Greenfield S, Candy D, et al. Systematic review: impact of constipation on quality of life in adults and children. Aliment Pharmacol Ther 2010;31:938-49. [Crossref] [PubMed]
- Yost KJ, Haan MN, Levine RA, et al. Comparing SF-36 scores across three groups of women with different health profiles. Qual Life Res 2005;14:1251-61. [Crossref] [PubMed]
- Sailer M. Slow Transit Constipation. Zentralbl Chir 2019;144:179-89. [Crossref] [PubMed]
- Lv W. Understanding traditional Chinese medicine. Hepatobiliary Surg Nutr 2021;10:846-8. [Crossref] [PubMed]
- Iantorno G, Bassotti G, Kogan Z, et al. The enteric nervous system in chagasic and idiopathic megacolon. Am J Surg Pathol 2007;31:460-8. [Crossref] [PubMed]
- Bassotti G, Villanacci V, Maurer CA, et al. The role of glial cells and apoptosis of enteric neurones in the neuropathology of intractable slow transit constipation. Gut 2006;55:41-6. [Crossref] [PubMed]
- Zhao RH, Baig MK, Thaler KJ, et al. Reduced expression of serotonin receptor(s) in the left colon of patients with colonic inertia. Dis Colon Rectum 2003;46:81-6. [Crossref] [PubMed]
- Kim JE, Kang MJ, Choi JY, et al. Regulation of gastrointestinal hormones during laxative activity of gallotannin-enriched extract isolated from Galla Rhois in loperamide-induced constipation of SD rats. Lab Anim Res 2018;34:223-31. [Crossref] [PubMed]
- Lan J, Wang K, Chen G, et al. Effects of inulin and isomalto-oligosaccharide on diphenoxylate-induced constipation, gastrointestinal motility-related hormones, short-chain fatty acids, and the intestinal flora in rats. Food Funct 2020;11:9216-25. [Crossref] [PubMed]
- Yan S, Yue YZ, Wang XP, et al. Aqueous Extracts of Herba Cistanche Promoted Intestinal Motility in Loperamide-Induced Constipation Rats by Ameliorating the Interstitial Cells of Cajal. Evid Based Complement Alternat Med 2017;2017:6236904. [Crossref] [PubMed]
- Xu J, Chen Y, Liu S, et al. Electroacupuncture regulates apoptosis/proliferation of intramuscular interstitial cells of cajal and restores colonic motility in diabetic constipation rats. Evid Based Complement Alternat Med 2013;2013:584179. [Crossref] [PubMed]
- Cheng P, Yao J, Wang C, et al. Molecular and cellular mechanisms of tight junction dysfunction in the irritable bowel syndrome. Mol Med Rep 2015;12:3257-64. [Crossref] [PubMed]
- Wang L, Wu F, Hong Y, et al. Research progress in the treatment of slow transit constipation by traditional Chinese medicine. J Ethnopharmacol 2022;290:115075. [Crossref] [PubMed]
- Brochard C, Chambaz M, Ropert A, et al. Quality of life in 1870 patients with constipation and/or fecal incontinence: Constipation should not be underestimated. Clin Res Hepatol Gastroenterol 2019;43:682-7.
- Hughes S, Higgs NB, Turnberg LA. Loperamide has antisecretory activity in the human jejunum in vivo. Gut 1984;25:931-5. [Crossref] [PubMed]
- Sohji Y, Kawashima K, Shimizu M. Pharmacological studies of loperamide, an anti-diarrheal agent. III. Interaction between loperamide and various agonists in the guinea pig intestine (author's transl). Nihon Yakurigaku Zasshi 1978;74:213-23. [Crossref] [PubMed]
- Kakino M, Izuta H, Ito T, et al. Agarwood induced laxative effects via acetylcholine receptors on loperamide-induced constipation in mice. Biosci Biotechnol Biochem 2010;74:1550-5. [Crossref] [PubMed]
- Yin J, Liang Y, Wang D, et al. Naringenin induces laxative effects by upregulating the expression levels of c-Kit and SCF, as well as those of aquaporin 3 in mice with loperamide-induced constipation. Int J Mol Med 2018;41:649-58. [Crossref] [PubMed]
- Iijima K, Koike T, Abe Y, et al. Cutoff serum pepsinogen values for predicting gastric acid secretion status. Tohoku J Exp Med 2014;232:293-300. [Crossref] [PubMed]
- Yik YI, Farmer PJ, King SK, et al. Gender differences in reduced substance P (SP) in children with slow-transit constipation. Pediatr Surg Int 2011;27:699-704. [Crossref] [PubMed]
- Deng Z, Fu Z, Yan W, et al. The different effects of Chinese Herb Solid Drink and lactulose on gut microbiota in rats with slow transit constipation induced by compound diphenoxylate. Food Res Int 2021;143:110273. [Crossref] [PubMed]
- Zhang Q, Zhong D, Sun R, et al. Prevention of loperamide induced constipation in mice by KGM and the mechanisms of different gastrointestinal tract microbiota regulation. Carbohydr Polym 2021;256:117418. [Crossref] [PubMed]
- Huizinga JD, Hussain A, Chen JH. Interstitial cells of Cajal and human colon motility in health and disease. Am J Physiol Gastrointest Liver Physiol 2021;321:G552-75. [Crossref] [PubMed]
- Huizinga JD, Chen JH. Interstitial cells of Cajal: update on basic and clinical science. Curr Gastroenterol Rep 2014;16:363. [Crossref] [PubMed]
- Colegio OR, Van Itallie C, Rahner C, et al. Claudin extracellular domains determine paracellular charge selectivity and resistance but not tight junction fibril architecture. Am J Physiol Cell Physiol 2003;284:C1346-54.
- Annaházi A, Ferrier L, Bézirard V, et al. Luminal cysteine-proteases degrade colonic tight junction structure and are responsible for abdominal pain in constipation-predominant IBS. Am J Gastroenterol 2013;108:1322-31. [Crossref] [PubMed]
- Bertiaux-Vandaële N, Youmba SB, Belmonte L, et al. The expression and the cellular distribution of the tight junction proteins are altered in irritable bowel syndrome patients with differences according to the disease subtype. Am J Gastroenterol 2011;106:2165-73. [Crossref] [PubMed]
(English Language Editor: J. Jones)