Esophageal cancer-related gene 4 inhibits gastric cancer growth and metastasis by upregulating Krüppel-like factor 2 expression
Highlight box
Key findings
• Overexpression of ECRG4 inhibited the proliferation, metastasis, and invasion of gastric cancer (GC) cells. RNA sequencing analysis showed that ECRG4 overexpression induced the upregulation of Krüppel-like factor 2 (KLF2).
What is known and what is new?
• The ECRG4 mRNA and protein levels in GC were low and were associated with a poor prognosis;
• RNA sequencing analysis showed that ECRG4 overexpression induced the upregulation of KLF2.
What are the implications, and what should change now?
• Further studies are crucial to elucidating the specific mechanisms and pathways related to the involvement of ECRG4 and KLF2 in GC.
Introduction
Gastric cancer (GC) is the fifth most common cancer and the third leading cause of cancer-related death worldwide (1). The prognosis of GC has improved minimally, and ongoing clinical trials are seeking to identify novel effective combination therapies (2). Despite an extensive search for novel molecular biomarkers, there are still no effective molecular criteria for stratifying patients with GC for optimal treatment based on prognosis. Therefore, effective GC biomarkers along with treatment targets are urgently needed. These developments would facilitate precision medicine for patients with GC (3).
Esophageal cancer-related gene 4 (ECRG4) is located on chromosome 2q12.2 and encodes 148 amino acid products. The 447 bp open reading frame of ECRG4 has four exons (4). The study has proposed aberrant ECRG4 promoter methylation as a possible indicator of early disease and predictor of pathological stage in GC (5). Reversal of ECRG4 hypermethylation using 5-azacytidine has been proposed as a potential GC treatment (6). Overexpression of ECRG4 has been reported to enhance the chemosensitivity of SGC-7901 GC cells to 5-fluorouracil through inducing apoptosis (7). A recent study showed that downregulation of miRNA-196b hindered the progress of GC via modulation of ECRG4 expression, suggesting that miR-196b is a possible treatment target in GC (8).
Krüppel-like factor 2 (KLF2) is a member of the Krüppel-like factor family of transcription factors. Krüppel-like factors regulate various biological processes (9), and KLF2 is thought to act as a tumor suppressor in various cancers. Mao et al. reported that overexpression of KLF2 dampened the proliferation and invasion potential of GC cells and downregulated the expression of proliferating cell nuclear antigen, B-cell lymphoma-2, and matrix metalloproteinase-9 (10). Huo et al. found that the sphingosine kinase 2/KLF2 pathway regulates the proliferation, migration, and invasion of methyltransferase 3-induced GC cells (11).
Currently, the ECRG4 level before treatment could be used as a prognostic factor (12). the mechanisms underlying the association between loss of ECRG4 and a poor prognosis of GC are unclear. Transcriptome sequencing of AGS and HGC27 GC cells overexpressing ECRG4 identified KLF2 as a downstream target of ECRG4. Overexpression of ECRG4 was correlated with a significant reduction in the expression of the epithelial-mesenchymal transition (EMT)-associated factor Snail and the upregulation of epithelial cadherin (E-cadherin). Therefore, to investigate whether ECRG4 and KLF2 modulate GC cell function and to determine their effect on the biological function of GC cells and GC clinicopathological parameters and prognoses, we silenced KLF2 in ECRG4-overexpressing cells. In addition, we carried out animal experiments to validate the inhibitory role of ECRG4 on KLF2 and determine its prognostic value in GC. We present the following article in accordance with the ARRIVE reporting checklist (available at https://atm.amegroups.com/article/view/10.21037/atm-23-139/rc).
Methods
Real-time quantitative PCR (RT-qPCR) analysis
RT-qPCR was done with the SYBR® Premix Ex TaqTM II kit (Tli RNase H Plus, RR820Q). A melt curve was generated to check the specificity of the primers and detect the presence of primer dimers. The primers used were as follows:
- ECRG4-F: 5'-ACTAAGACTAAAGTGGCCGTTG-3';
- ECRG4-R: 5'-AATTTCGCTTCGTCAAAGCCC-3';
- KLF2-F: 5'-CTACACCAAGAGTTCGCATCTG-3';
- KLF2-R: 5'-CCGTGTGCTTTCGGTAGTG-3';
- Human β-actin-F1: 5'-GAAGAGCTACGAGCTGCCTGA-3';
- Human β-actin-R1: 5'-CAGACAGCACTGTGTTGGCG-3'.
The RT-qPCR was done using the following cycling conditions: 95 ℃ for 30 s, 95 ℃ for 5 s, 60 ℃ for 30 s, repeat for 40 cycles. The signal was collected at 95 ℃ for 5 s, 60 ℃ for 60 s, and 95 ℃ for 60 s. The relative gene expression levels were calculated using the 2−ΔΔCt method.
Western blot
The protein concentration of GC cells was measured using the bicinchoninic acid (BCA) assay. First, 50 µg of each protein sample was fractionated on sodium dodecyl-sulfate polyacrylamide gel electrophoresis gels. The proteins were then transferred to polyvinylidene difluoride membranes and blocked for 2 hours at room temperature with 5% skimmed milk/tris-buffered saline with Tween 20. The samples were inoculated for 2 h with horseradish peroxidase-linked (1:1,000 dilution) rabbit anti-goat IgG antibodies (1:2,000 dilution) at 37 ℃. The membranes were then rinsed three times with tris-buffered saline, and the signals were developed using an enhanced chemiluminescence kit (Amersham Biosciences). Finally, the results were analyzed using Quantity One software (Bio-Rad).
Patient data
Paired GC tissues and paraffin sections of adjacent noncancerous stomach tissue microarrays were acquired from patients who underwent surgical resection for GC from the People’s Hospital of Ningxia Hui Autonomous Region between 2009 and 2020. The tissues were deposited in Shanghai Zhangjiang Biobank, China. The patients were followed until death or 1st January 2019, whichever came first. In total, paraffin-embedded GC tissue samples were obtained from 136 patients (72 males and 64 females, median age: 54 years, age range, 18–78 years). The enrolled patients were followed up through telephone interviews for 1–120 months (mean follow-up period: 39.7 months). In addition, a panel of six fresh tumor tissues and adjacent noncancerous tissues obtained from patients with GC who underwent surgical resection at the People’s Hospital of Ningxia Hui Autonomous Region, China, in 2020 and 2021, was used. Clinicopathologic data were abstracted from the patients’ medical records. None of the patients had received chemotherapy or radiation therapy before sample collection. The following patients were excluded: (I) patients with atrial fibrillation or serious heart disease; (II) patients with Alzheimer’s disease; and (III) patients who had experienced burns, trauma, or stress recently (i.e., within 1 month). The study was conducted in accordance with the Declaration of Helsinki (as revised in 2013). The study was approved by ethics board of People’s Hospital of Ningxia Hui Autonomous Region (approval No. 2017-034). Informed consent was taken from all the patients.
Immunohistochemistry (IHC)
Immunohistochemical analysis was performed to detect ECRG4 and KLF2 protein expression in the GC tissue and para-carcinoma tissue. Tissue sections were first dewaxed and antigen-repaired, before being inoculated overnight with primary antibodies at 4 ℃. After that, the slides were inoculated with secondary antibodies in an automatic IHC instrument. Finally, the signal was developed using color developer dab (DAB) (3,3'-diaminobenzidine) chromogen, and hematoxylin was used for counterstaining. The staining intensity was scored as follows: 0= negative staining, 1= mild intensity, 2= moderate intensity, and 3= strong intensity. The IHC score took into account both the staining intensity and the positive rate. The total score (0–300%) was determined using the formula: intensity of staining (0, 1, 2, or 3) × extent of staining (0–100%). The IHC results were independently examined by two pathologists with no knowledge of the clinical data.
Cell culture
The human GC cell lines AGS and HGC27 were obtained from the China and Shanghai National Engineering Research Centre for Biochips. The AGS and HGC27 cells were inoculated in F-12K and RPMI 1640 complete media, respectively. The cell culture media were purchased from Gibco.
Lentivirus infection and gene knockdown
Transfection was performed using the LipofectamineTM 2000 system (Invitrogen) following the instructions provided by the manufacturer. Small interfering (si) RNA against KLF2 was purchased from Invitrogen and had the following sequences: hKLF2 si-1: sense GCACCGACGACGACCUCAATT, antisense UUGAGGUCGUCGUCGGUGCTT; hKLF2 si-2: sense UCAACAGCGUGCUGGACUUTT, antisense AAGUCCAGCACGCUGUUGATT; hKLF2 Si-3: sense UGCUGGAGGCCAAGCCAAATT, antisense UUUGGCUUGGCCUCCAGCATT. The knockdown efficiency was determined using RT-qPCR.
Cell viability analysis
Cell viability was assessed with Cell Counting Kit-8 (CCK-8) assays (Beyotime Biotechnology, Shanghai, China). After cell transfection, the cell suspensions were inoculated on a 96-well plate (100 µL/well), and 10 µL/well of CCK-8 was added. The cells were then left to grow for 2 h, and the optical density (OD) was read using a microplate reader at a wavelength of 450 nm.
Colony formation assay
For colony formation assays, 300 cells/well were inoculated in triplicate onto 6-well plates. The cells were carefully rinsed twice with precooled phosphate-buffered saline, and then fixed with 2 mL of precooled 4% paraformaldehyde for 10–15 min. After the removal of the fixation solution, 2 mL of crystal violet staining solution was introduced for 10 min. The cells were then washed, dried, and counted. The clone formation rate was computed as follows: rate of clone formation = (clone number/inoculated cell number) ×100%.
Apoptosis analysis
Apoptosis was analyzed using Hoechst 33342/propidium iodide (PI) double staining. First, the cell density was adjusted to 1×105 cells per mL. Then, 100 µL of cell suspension was inoculated on a 96-well plate for 24 h at 37 ℃, after which 100 µL staining solution (Hochest 10 µg/mL, PI 20 µM) was introduced into every well and the cells were incubated at 37 ℃ for 20 min in the dark. Finally, Acumen heat was turned on and scans were adjusted for apoptosis detection. The total number and intensity of the output fluorescent objects were calculated using the Acumen machine's automated apoptosis detection system.
Wound healing assay
The migration potential of GC cells was explored via wound healing assays. At 24 h after transfection, the cell monolayers were scratched with a 200 µL pipette tip, and photos of the wound were taken. After 24 h, the cells were imaged under a microscope. The scratch area was quantified using Image J software. The area recovery percentage was calculated as follows: area recovery % = (0-h scratch area – 24-h scratch area) scratch area/0-h scratch area ×100%.
Transwell cell migration and invasion assay
Transwell cell migration and invasion assay was performed to analyze cell invasion and migration. First, a Transwell chamber was put on a 24-well culture plate, and 100 µL of transfected cells in a serum-free medium (cell density: 1×105 cells/mL) were inoculated into its upper chamber. Then, 600 µL of complete medium enriched with 10% fetal bovine serum was introduced into the lower chamber. After 5 days of culture, the cells were stained with crystal violet, then imaged, and the number of migrating cells was counted.
RNA sequence and pathway enrichment analysis
Isolation of total RNA from AGS cells in ECRG4-overexpressing and negative control (NC) groups was performed with TRIzol reagent (Invitrogen). The RNA quantity and quality were assessed with a NanoDrop ND-2000 and Agilent Bioanalyzer 4200 (Agilent Technologies, USA), and the RNA was sequenced after inspection. The Seqtk software was used for preprocessing of the sequencing data. The HISAT2 software was used for genome mapping analysis, mRNA quantification, and differential analysis. The trimmed mean of M-values algorithm in edgeR was used to normalize the fragment counts. Finally, multiple hypothesis testing and P value corrections were done to obtain the false discovery rate (q-value). Fold-change was calculated based on fragments per kilobase million (FPKM) values. Fisher’s exact test was used for gene ontology (GO) analysis of differentially expressed genes (DEGs). The number of DEGs in each Kyoto Encyclopedia of Genes and Genomes (KEGG) pathway group was statistically analyzed.
In vivo tumor xenograft experiment
Animal experiments were performed under a project license (No. 2017-034-2) granted by ethics board of NingXia Medical University, in compliance with national guidelines for the care and use of animals. A protocol was prepared before the study without registration. Five-week-old male BALB/c nude mice were Purchased from Hangzhou Ziyuan Experimental Animal Technology Co., Ltd. in this study. Each mouse was subcutaneously injected with 5×106 cells into the right armpit. The weight and tumor volume of the mice were assessed every 3 days for 24 consecutive days. After 24 days, the mice were sacrificed. The tumors were imaged, and their sizes and weights were recorded.
Statistical analyses
Statistical analyses were conducted using SPSS 19.0 (SPSS Inc., Chicago, IL, USA). An independent t-test was adopted to assess differences in ECRG4 expression between GC and neighboring non-tumorous tissues. Pearson’s χ2 and Fisher’s exact tests were used to analyze the correlations between ECRG4 expression and various clinicopathological factors in GC. The Kaplan-Meier estimator and log-rank test were used to assess the survival of patients with GC. The prognostic implications of ECRG4 expression and clinicopathological parameters were assessed using Cox univariate and multivariate proportional hazards models. The Student’s t-test (two-tailed), one-way ANOVA, and the Mann-Whitney U test were used to analyze the in vitro and in vivo data. Statistically significant differences were indicated by P≤0.05.
Results
Downregulation of ECRG4 is correlated with a poor GC prognosis
The RT-qPCR analysis of the ECRG4 mRNA content in 45 paired GC tissue samples and adjacent histologically non-tumorous tissue samples revealed that ECRG4 expression was remarkably lower in GC tissues (P≤0.01, Figure 1A). Kaplan-Meier analysis showed that the group with low ECRG4 mRNA levels had worse survival than the group with high ECRG4 mRNA levels (P=0.028, Figure 1B). These data implicate abnormal ECRG4 expression in the pathogenesis of GC.
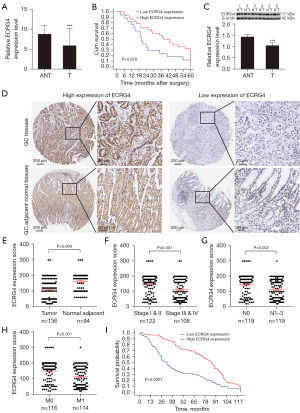
Western blot analysis of the ECRG4 content in six GC tissues and paired adjacent non-tumorous tissues revealed remarkably lower ECRG4 levels in the GC tissues (Figure 1C). Immunohistochemical analysis of the ECRG4 expression levels in microarrays of 136 GC tissues and 94 adjacent non-GC tissues (Figure 1D) revealed that ECRG4 was upregulated in 52.94% (72/136) of the GC tissues, downregulated in 47.06% (64/136) of the GC tissues, upregulated in 70.21% (66/94) of the paracancerous non-tumor tissues, and downregulated in 20.59% (28/136) of the non-tumorous adjacent tissues (Figure 1E).
Analysis of IHC and clinicopathological parameters showed that in patients with GC, ECRG4 expression was linked with age (P=0.004), clinical stage (P=0.006), lymph node metastasis (P=0.011), and distant metastasis (P=0.002, Table 1). The ECRG4 expression levels of patients with clinical stage III/IV (P≤0.001), lymph node metastasis (P=0.002), and distant metastasis (P≤0.001) were remarkably lower than those of patients in the control group (Figure 1F-1H). Deng et al.’s research shows that the expression level of ECRG4 is positively related to lymph node metastasis (6). Moreover, Kaplan-Meier analysis showed that patients with GC who had low ECRG4 expression had poor overall survival (P<0.0001, Figure 1I). Age, stage, lymph nodes, distant metastasis, and ECRG4 expression were all linked to prognosis (P<0.01, Table 2). Of note, ECRG4 was established as an independent prognostic factor in patients with GC [hazard ratio (HR) =0.442, 95% confidence interval (CI): 0.291–0.672, P<0.01]. In addition, distant metastasis was identified as an independent risk predictor for overall survival (HR =2.072, 95% CI: 1.170–3.669, P=0.012, Table 2).
Table 1
Variables | Total (n=136) | ECRG4 expression, n (%) | KLF2 expression, n (%) | |||||||
---|---|---|---|---|---|---|---|---|---|---|
Low | High | χ2 | P value | Low | High | χ2 | P value | |||
Sex | 0.217 | 0.641 | 0.062 | 0.804 | ||||||
Male | 73 | 33 | 40 | 34 | 39 | |||||
Female | 63 | 31 | 32 | 28 | 35 | |||||
Age | 8.172 | 0.004* | 11.326 | 0.001* | ||||||
<65 years | 74 | 26 | 48 | 24 | 50 | |||||
≥65 years | 62 | 37 | 25 | 38 | 24 | |||||
Tumor stage | 7.451 | 0.006* | 11.659 | 0.001* | ||||||
I/II | 70 | 25 | 45 | 22 | 48 | |||||
III/IV | 66 | 39 | 27 | 40 | 26 | |||||
N stage | 6.498 | 0.011* | 18.172 | 0* | ||||||
N0 | 71 | 26 | 45 | 20 | 51 | |||||
N1–N3 | 65 | 38 | 27 | 42 | 23 | |||||
Metastatic stage | 9.563 | 0.002* | 17.074 | 0* | ||||||
M0 | 68 | 23 | 45 | 19 | 49 | |||||
M1 | 68 | 41 | 27 | 43 | 25 | |||||
Tumor size | 0.347 | 0.556 | 0.170 | 0.680 | ||||||
<3 cm | 75 | 37 | 38 | 33 | 42 | |||||
≥3 cm | 61 | 27 | 34 | 29 | 32 | |||||
H. pylori infection | 0.292 | 0.589 | 2.780 | 0.095 | ||||||
Yes | 84 | 38 | 46 | 43 | 41 | |||||
No | 52 | 26 | 26 | 19 | 33 |
*, P<0.05. ECRG4, esophageal cancer-related gene 4; KLF2, Krüppel-like factor 2.
Table 2
Parameter | Univariate analysis | Multivariate analysis | |||||
---|---|---|---|---|---|---|---|
HR | 95 % CI | P value | HR | 95 % CI | P value | ||
Sex (female vs. male) | 0.988 | 0.693–1.406 | 0.944 | – | – | – | |
Age (<65 vs. ≥65 years) | 2.848 | 1.943–4.175 | 8.185e–8** | 3.266 | 0.911–11.715 | 0.069 | |
Tumor stage (I/II vs. III/IV) | 2.500 | 1.736–3.601 | 8.507e–7** | 0.880 | 0.256–3.026 | 0.839 | |
N stage (N0 vs. N1–N3) | 2.446 | 1.703–3.513 | 0** | 1.310 | 0.744–2.308 | 0.35 | |
Metastatic stage (M0 vs. M1) | 2.810 | 1.953–4.045 | 2.666e–8** | 2.072 | 1.170–3.669 | 0.012* | |
T stage (T1/T2 vs. T3/T4) | 1.293 | 0.906–1.846 | 0.157 | – | – | – | |
H. pylori infection (yes vs. no) | 0.925 | 0.643–1.331 | 0.6742 | – | – | – | |
ECRG4 expression (low vs. high) | 0.474 | 0.331–0.680 | 0** | 0.442 | 0.291–0.672 | 0** | |
KLF2 expression (low vs. high) | 0.373 | 0.260–0.535 | 9.134e–8** | 0.484 | 0.327–0.716 | 0** |
*, P<0.05; **, P<0.01. ECRG4, esophageal cancer-related gene 4; KLF2, Krüppel-like factor 2; HR, hazard ratio; CI, confidence interval.
Overexpression of ECRG4 inhibits GC cell proliferation and migration
Stable ECRG4 expression was established in two GC cell lines (AGS and HGC-27) by lentiviral vector-mediated overexpression (Figure 2). Control cells were transfected with an empty vector (pcDNA). Analysis by CCK-8 assay revealed remarkably lower cell viability in the ECRG4-overexpressing group than in the NC group (P=0.00086<0.05, Figure 2A). Clone formation assay further showed that ECRG4 overexpression dampened the proliferation of AGS and HGC27 cells (P=0.00003<0.05, Figure 2B). Also, Hoechst-PI analysis revealed that ECRG4 overexpression remarkably increased the apoptotic rate of AGS and HGC27 cells (P0.000045<0.05, Figure 2C,2D).
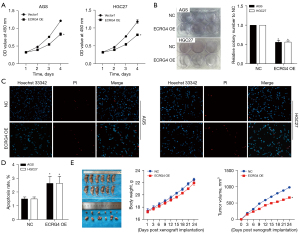
Results from the mouse tumor xenograft model bearing AGS cells with ECRG4 overexpression revealed that the average tumor volume in the ECRG4-overexpressing group was remarkably lower than that in the NC group (Figure 2E). This finding is consistent with those showing that ECRG4 overexpression inhibits GC cell growth.
Transwell and wound healing assay results showed that ECRG4 overexpression also influences the migration and invasion of the GC cell lines, AGS and HCG27. Transwell migration and Matrigel invasion assay results revealed decreased migration and invasion in the ECRG4-overexpressing group compared with the NC group (P=0.00023<0.05, Figure 3A), and wound healing analysis revealed that ECRG4 overexpression inhibits the migration of AGS and HGC27 cells (P=0.0032<0.05, Figure 3B).
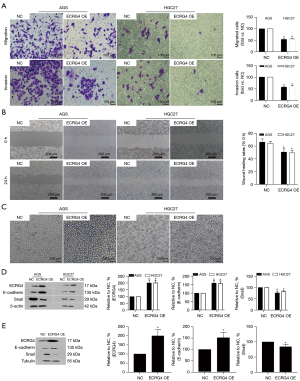
AGS and HGC27 cells with ECRG4 overexpression exhibited spindle-shaped changes, and the connections between the cells became less tight (Figure 3C), suggesting that elevated ECRG4 expression may induce EMT. Next, the expression of the well-known EMT-associated proteins E-cadherin and Snail was investigated. Western blotting showed that ECRG4 overexpression remarkably upregulated the expression of E-cadherin (an anti-EMT factor) but decreased that of Snail (an EMT inducer) (Figure 3D,3E).
KLF2 is a downstream target of ECRG4 in GC
To investigate the mechanisms underlying the function of ECRG4 in GC, we carried out RNA sequencing of cells with ECRG4 overexpression. After KEGG pathway analysis, the number of DEGs in each pathway category were counted, and the results are graphically displayed in Figure 4A. There were 312 upregulated genes and 36 downregulated genes in the ECRG4-overexpressing group compared to the NC group. Next, a volcano map analysis of the overall distribution of the DEGs was done (Figure 4B). Cluster analysis of ECRG4 overexpressed DEGs was performed to identify genes with related expression patterns. Such genes may have common functions or participate in common signaling pathways (Figure 4C). Log 10 (FPKM+1) values were normalized (scale number) and clustered. Among these DEGs, we focused on KLF2, whose expression was remarkably upregulated upon ECRG4 overexpression (P≤0.0001, Figure 4D). KLF2 is known to suppress the growth and invasion of GC cells (10). Western blot analysis showed that KLF2 protein expression levels were increased in the ECRG4-overexpressing group compared with the NC group (P<0.001, Figure 4E,4F; P=0.00032<0.05, Figure 4G).
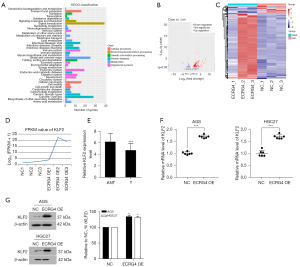
ECRG4 influences GC progression by regulating KLF2
To investigate the role of ECRG4 in GC, we silenced KLF2 in AGS and HGC27 cells using KLF2 short hairpin RNA lentiviral transfection. Results of CCK8 and clone formation analyses revealed that KLF2 knockdown partially rescued the cell viability and proliferation inhibition observed after ECRG4 overexpression (Figure 5A,5B). Western blot analysis showed that KLF2 was effectively knocked out (Figure 5C). Hoechst-PI staining analysis showed that KLF2 knockdown reduced apoptosis in the ECRG4-overexpressing group (Figure 5D). Furthermore, Transwell migration and invasion assays confirmed that KLF knockdown enhanced the ability of cells with ECRG4 overexpression to restrain cell migration and invasion (Figure 5E). Also, KLF knockdown enhanced the wound healing rate (Figure 5F). In vivo tumor growth assays showed that KLF2 knockdown did not reduce the body weight of nude mice (Figure 5G). The average tumor volume in the group with ECRG4 overexpression and KLF2 knockdown was higher than that in the group with only ECRG4 overexpression (Figure 5H), which had been inhibited in cells with ECRG4 overexpression. In vitro and in vivo analyses showed that KLF2 knockdown also reversed the changes to EMT markers induced by ECRG4 overexpression, decreasing E-cadherin and increasing Snail expression (Figure5I). Overexpression of ECRG4 dampened the proliferation and migration of GC cells and promoted apoptosis, while KLF2 counteracted these effects (P≤0.05, Figure 5).
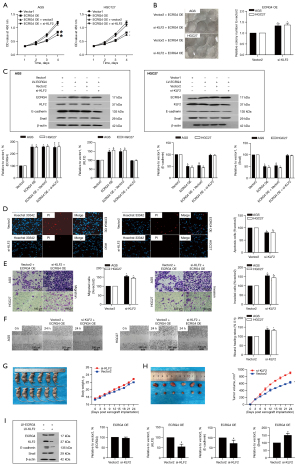
Correlation of ECRG4 and KLF2 expression with GC prognosis
Results of IHC analysis revealed remarkably lower KLF2 levels in GC tissues than in adjacent non-tumorous tissues (P<0.001, Figure 6A,6B). Analysis of the relationships of KLF2 content with clinicopathological features in patients with GC (Table 1) revealed that KLF2 expression was associated with age (P=0.001), clinical stage (P=0.001), lymph node metastasis (P<0.05), and distant metastasis (P=0.0082 <0.05). The rates of lymph node and distant metastases were remarkably lower among patients with clinical stage III/IV GC than among the controls (P=0.00059 <0.001, Figure 6B). Kaplan-Meier survival curve analysis showed that patients with GC with low KLF2 expression had poor overall survival (P<0.0001, Figure 6C). Multivariate Cox survival analysis revealed a positive correlation between KLF2 expression and GC survival (HR =0.484, 95% CI: 0.327–0.716, P=0.00065<0.01, Table 2).
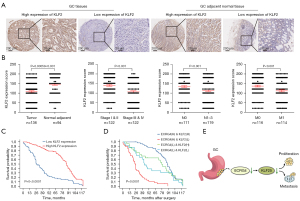
Finally, we assessed whether detecting ECRG4 and KLF2 expression in combination was more accurate in predicting GC prognosis than detecting either ECRG4 or KLF2 expression alone. Based on the IHC results, patients were divided into the high ECRG4/high KLF2, high ECRG4/low KLF2, low ECRG4/high KLF2, and low ECRG4/low KLF2 groups. The high ECRG4/high KLF2 group had a better prognosis than the high ECRG4/low KLF2 group (P=0.034), but there was no significant difference in prognosis between the high ECRG4/low KLF2 group and the low ECRG4/high KLF2 group (P=0.329). The low ECRG4/low KLF2 group had a poorer prognosis than the low ECRG4/high KLF2 group (P=0.0001, Figure 6D). These data suggest that KLF2 influences the development of GC and participates in its ECRG4-mediated progression (Figure 6E).
Discussion
GC has high morbidity and is the second leading cause of cancer-associated death worldwide (13). Before developing an individualized treatment plan for GC, it is essential to evaluate the patient’s condition and prognosis. Accurate prognostic evaluation is necessary to select appropriate treatment strategies for patients with GC. Therefore, effective prognostic biomarkers for GC are urgently needed.
ECRG4 was first reported in 1998 by a team of researchers from Peking Union Medical College of China, who found that it was more upregulated in esophageal tumor-adjacent tissues compared with cancerous tissues (14). ECRG4 is reported to influence a variety of processes, including inflammatory response to injury (15,16), cardiovascular function (17), neural cell senescence, aging (18,19), stress (20), and articular chondrocyte differentiation (21). ECRG4 also works as a tumor repressor in various cancers, including esophageal, stomach, colorectal, and breast cancer (6,22,23). Recent studies indicate that ECRG has a cytokine-like functional pattern. MicroRNA-196b (miR-196b) was significantly up-regulated and ECRG4 was significantly down-regulated in GC cells. Down-regulation of miR-196b can inhibit the proliferation, migration and invasion of GC cells, while down-regulation of ECRG can reverse this effect (8). Upon activation, it is released from the cell membrane and is detected in liquid biopsies, making it suitable for application in precision medicine (24).
In line with previous research findings (25), our study results suggest that ECRG4 is a tumor suppressor and that its high expression is correlated with a better GC prognosis. Using multivariate analysis, we identified ECRG4 expression to be an independent indicator of good overall survival in GC (Table 2). Our data suggest that ECRG4 may play an important role in GC progression.
Further analysis found that overexpression of ECRG4 remarkably inhibited GC cell proliferation and migration, and promoted apoptosis. Previously, ECRG4 overexpression has been reported to markedly inhibit the migration and invasion of various malignant tumor cells (4,26). Experimental results from a nude mouse xenograft tumor model confirmed the dampening effect of ECRG4 on tumor growth in GC. An unanticipated finding of our analysis was that GC cells with ECRG4 overexpression displayed spindle-shaped changes with less tight intercellular connections, which indicated that ECRG4 might induce EMT in GC cells. Western blotting data revealed that ECRG4 overexpression remarkably increased the expression levels of E-cadherin, an anti-EMT factor, in vivo and in vitro, while decreasing the level of Snail, a pro-EMT factor. These results suggest that overexpression of ECRG4 in GC cells may induce EMT. In addition, knockdown of KLF2 knockdown markedly reversed the effects of ECRG4 overexpression on the expression of EMT-linked proteins in GC cells. These findings suggest that KLF2 is a potential downstream target of ECRG4 and may be a mediator of its anticancer effect in GC.
An IHC tissue array analysis of KLF2 levels in GC revealed that KLF2 levels in GC samples were lower than those in adjacent non-tumor samples. The expression level of KLF2 was linked to age, clinical stage, lymph node metastasis, and distant metastasis.
The Kaplan-Meier survival analysis revealed that patients with GC who had high ECRG4/and high KLF2 expression had a better prognosis, which suggests that higher ECRG4 and KLF2 levels restrain GC progression. Our study found the relationship between ECRG4 and KLF2 in GC, which is of certain value in predicting the prognosis and treatment success of patients with GC, and this finding has practical significance for the research on the treatment and management of patients with GC. In nasopharyngeal carcinoma, downregulation of ECRG4 has been found to promote tumor growth and invasion by activating the AKT/GSK3β/β-catenin signaling pathway (27). Furthermore, in esophageal squamous cell carcinoma, ECRG4 suppresses tumor cell migration and invasion without affecting cell adhesion. Li et al. found that ECRG4 might cause cell cycle arrest in the G1 phase in esophageal squamous cell carcinoma, probably by upregulating p53 and p21 (28). Another study on esophageal squamous cell carcinoma discovered that ECRG4 also directly interacts with ECRG1 to upregulate p21, induce G1 phase cell cycle arrest, and inhibit cancer cell proliferation (29). KLF2 has also been found to interact with forkhead box O4 to induce p21 expression. Yuedi et al. reported that downregulation of p21 and forkhead box O4 inhibited KLF2-induced senescence (30). However, it is not clear if ECRG4 and KLF2 are associated with p21 in GC. Therefore, further research is pivotal to elucidate the mechanisms and pathways underlying the involvement of ECRG4 in GC, ECRG4 may serve as a biomarker for risk stratification and local metastasis in patients with GC.
Conclusions
Our study found that ECRG4 acts as a tumor suppressor by inhibiting the growth and metastasis of GC cells. Decreased expression of ECRG4 or KLF2 is correlated with a poor prognosis of GC. Downregulation of KLF2 is involved in ECRG4-mediated GC progression. Therefore, targeting ECRG4 and KLF2 may be a promising strategy for treating GC.
Acknowledgments
We would like to thank all the staff that carried out the intervention and evaluation components of the study for their hard work and dedication.
Funding: This study was supported by grants from The National Natural Science Foundation of China (grant No. 8176100564), Hainan Province Clinical Medical Center Development Program (grant No. 2021818), Ningxia Hui Autonomous Region Key Research and Development Program (grant No. 2019BFG02003), and Ningxia Health Commission Scientific Research Fund Project (grant No. 2019NW024).
Footnote
Reporting Checklist: The authors have completed the ARRIVE reporting checklist. Available at https://atm.amegroups.com/article/view/10.21037/atm-23-139/rc
Data Sharing Statement: Available at https://atm.amegroups.com/article/view/10.21037/atm-23-139/dss
Peer Review File: Available at https://atm.amegroups.com/article/view/10.21037/atm-23-139/prf
Conflicts of Interest: All authors have completed the ICMJE uniform disclosure form (available at https://atm.amegroups.com/article/view/10.21037/atm-23-139/coif). The authors have no conflicts of interest to declare.
Ethical Statement: The authors are accountable for all aspects of the work in ensuring that questions related to the accuracy or integrity of any part of the work are appropriately investigated and resolved. The study was conducted in accordance with the Declaration of Helsinki (as revised in 2013). The study was approved by ethics board of People’s Hospital of Ningxia Hui Autonomous Region (approval No. 2017-034). Informed consent was taken from all the patients. Animal experiments were performed under a project license (No. 2017-034-2) granted by ethics board of Ningxia Medical University, in compliance with national guidelines for the care and use of animals.
Open Access Statement: This is an Open Access article distributed in accordance with the Creative Commons Attribution-NonCommercial-NoDerivs 4.0 International License (CC BY-NC-ND 4.0), which permits the non-commercial replication and distribution of the article with the strict proviso that no changes or edits are made and the original work is properly cited (including links to both the formal publication through the relevant DOI and the license). See: https://creativecommons.org/licenses/by-nc-nd/4.0/.
References
- Thrift AP, El-Serag HB. Burden of Gastric Cancer. Clin Gastroenterol Hepatol 2020;18:534-42. [Crossref] [PubMed]
- Johnston FM, Beckman M. Updates on Management of Gastric Cancer. Curr Oncol Rep 2019;21:67. [Crossref] [PubMed]
- Ishii T, Kawazoe A, Shitara K. Dawn of precision medicine on gastric cancer. Int J Clin Oncol 2019;24:779-88. [Crossref] [PubMed]
- You Y, Yang W, Qin X, et al. ECRG4 acts as a tumor suppressor and as a determinant of chemotherapy resistance in human nasopharyngeal carcinoma. Cell Oncol (Dordr) 2015;38:205-14. [Crossref] [PubMed]
- Wang YB, Ba CF. Promoter methylation of esophageal cancer-related gene 4 in gastric cancer tissue and its clinical significance. Hepatogastroenterology 2012;59:1696-8. [Crossref] [PubMed]
- Deng P, Chang XJ, Gao ZM, et al. Downregulation and DNA methylation of ECRG4 in gastric cancer. Onco Targets Ther 2018;11:4019-28. [Crossref] [PubMed]
- Jiang CP, Wu BH, Wang BQ, et al. Overexpression of ECRG4 enhances chemosensitivity to 5-fluorouracil in the human gastric cancer SGC-7901 cell line. Tumour Biol 2013;34:2269-73. [Crossref] [PubMed]
- Chen L, Tang H, Liu G, et al. MicroRNA-196b promotes gastric cancer progression by targeting ECRG4. Anticancer Drugs 2021;32:127-37. [Crossref] [PubMed]
- McConnell BB, Yang VW. Mammalian Krüppel-like factors in health and diseases. Physiol Rev 2010;90:1337-81. [Crossref] [PubMed]
- Mao QQ, Chen JJ, Dong L, et al. Krüppel-like factor 2 suppresses growth and invasion of gastric cancer cells in vitro and in vivo. J Biol Regul Homeost Agents 2016;30:703-12.
- Huo FC, Zhu ZM, Zhu WT, et al. METTL3-mediated m(6)A methylation of SPHK2 promotes gastric cancer progression by targeting KLF2. Oncogene 2021;40:2968-81. [Crossref] [PubMed]
- Shi LY, Wang YY, Jing Y, et al. Abnormal arginine metabolism is associated with prognosis in patients of gastric cancer. Transl Cancer Res 2021;10:2451-69. [Crossref] [PubMed]
- Machlowska J, Baj J, Sitarz M, et al. Gastric Cancer: Epidemiology, Risk Factors, Classification, Genomic Characteristics and Treatment Strategies. Int J Mol Sci 2020;21:4012. [Crossref] [PubMed]
- Su T, Liu H, Lu S. Cloning and identification of cDNA fragments related to human esophageal cancer. Zhonghua Zhong Liu Za Zhi 1998;20:254-7.
- Dorschner RA, Lee J, Cohen O, et al. ECRG4 regulates neutrophil recruitment and CD44 expression during the inflammatory response to injury. Sci Adv 2020;6:eaay0518. [Crossref] [PubMed]
- Shaterian A, Kao S, Chen L, et al. The candidate tumor suppressor gene Ecrg4 as a wound terminating factor in cutaneous injury. Arch Dermatol Res 2013;305:141-9. [Crossref] [PubMed]
- Shakeel M, Irfan M, Khan IA. Rare genetic mutations in Pakistani patients with dilated cardiomyopathy. Gene 2018;673:134-9. [Crossref] [PubMed]
- Kujuro Y, Suzuki N, Kondo T. Esophageal cancer-related gene 4 is a secreted inducer of cell senescence expressed by aged CNS precursor cells. Proc Natl Acad Sci U S A 2010;107:8259-64. [Crossref] [PubMed]
- Nakatani Y, Kiyonari H, Kondo T. Ecrg4 deficiency extends the replicative capacity of neural stem cells in a Foxg1-dependent manner. Development 2019;146:dev168120. [Crossref] [PubMed]
- Stankiewicz AM, Goscik J, Swiergiel AH, et al. Social stress increases expression of hemoglobin genes in mouse prefrontal cortex. BMC Neurosci 2014;15:130. [Crossref] [PubMed]
- Huh YH, Ryu JH, Shin S, et al. Esophageal cancer related gene 4 (ECRG4) is a marker of articular chondrocyte differentiation and cartilage destruction. Gene 2009;448:7-15. [Crossref] [PubMed]
- Mori Y, Ishiguro H, Kuwabara Y, et al. Expression of ECRG4 is an independent prognostic factor for poor survival in patients with esophageal squamous cell carcinoma. Oncol Rep 2007;18:981-5.
- Götze S, Feldhaus V, Traska T, et al. ECRG4 is a candidate tumor suppressor gene frequently hypermethylated in colorectal carcinoma and glioma. BMC Cancer 2009;9:447. [Crossref] [PubMed]
- Qin X, Zhang P. ECRG4: a new potential target in precision medicine. Front Med 2019;13:540-6. [Crossref] [PubMed]
- You Y, Hu S. Dysregulation of ECRG4 is associated with malignant properties and of prognostic importance in human gastric cancer. Cancer Biomark 2022;34:55-66. [Crossref] [PubMed]
- Luo L, Wu J, Xie J, et al. Downregulated ECRG4 is associated with poor prognosis in renal cell cancer and is regulated by promoter DNA methylation. Tumour Biol 2016;37:1121-9. [Crossref] [PubMed]
- Xie Z, Li W, Ai J, et al. C2orf40 inhibits metastasis and regulates chemo-resistance and radio-resistance of nasopharyngeal carcinoma cells by influencing cell cycle and activating the PI3K/AKT/mTOR signaling pathway. J Transl Med 2022;20:264. [Crossref] [PubMed]
- Li L, Zhang C, Li X, et al. The candidate tumor suppressor gene ECRG4 inhibits cancer cells migration and invasion in esophageal carcinoma. J Exp Clin Cancer Res 2010;29:133. [Crossref] [PubMed]
- Li LW, Li YY, Li XY, et al. A novel tumor suppressor gene ECRG4 interacts directly with TMPRSS11A (ECRG1) to inhibit cancer cell growth in esophageal carcinoma. BMC Cancer 2011;11:52. [Crossref] [PubMed]
- Yuedi D, Houbao L, Pinxiang L, et al. KLF2 induces the senescence of pancreatic cancer cells by cooperating with FOXO4 to upregulate p21. Exp Cell Res 2020;388:111784. [Crossref] [PubMed]
(English Language Editor: J. Reylonds)