Inhibition of NF-κB ameliorates aberrant retinal glia activation and inflammatory responses in streptozotocin-induced diabetic rats
Highlight box
Key findings
• IMD-0354 inhibits nuclear factor-κB (NF-κB) activation in two types of glia cells to alleviate the severity of STZ-induced diabetic retinopathy in rats.
What is known and what is new?
• NF-κB is a pivotal transcription factor associated with d diabetes. The activation of microglia and Müller cells is responsible for maintaining retinal homeostasis and is regulated by NF-κB. The inhibitor of NF-κB (IMD-0354) has been proven to attenuate retinal inflammatory and metabolic responses.
• IMD-0354 attenuates oxidative injury, glial cell activation through inhibiting NF-κB activation. IMD-0354 inhibits VEGF secretion, inflammatory responses, and protects neurons from apoptosis in the retina of diabetic rats. Both types of glia (microglia and muller cells) exhibited a similar remissive state in response to IMD-0354.
What is the implication, and what should change now?
• Suppression of NF-κB signaling by IMD-0354 may represent a promising therapeutic strategy against DR through various mechanisms, further investigation of the effect of IMD-0345 on microglia and Müller cells in a STZ-induced rat model of diabetic retinopathy is needed.
Introduction
The most common microvascular complication of diabetes is diabetic retinopathy (DR). It is one of the leading causes of blindness in the working age population worldwide (1). DR has been considered as a microangiopathy with major pathological manifestations, including progressive destruction of the internal blood-retinal barrier, pericytes loss, and the development of microhemangioma. It is now generally accepted that DR results in pathological changes to all retinal cell types (1). Neurodegeneration of retinal ganglion cells (RGCs) and activation of glial cells are the two most early events in the pathogenesis of DR. Thereby, RGCs and glial cells become the primary targets for vision preservation in early DR (2).
Microglia and Müller cells are the fundamental glial cells responsible for maintaining retinal homeostasis. Not only do these cells communicate closely with the BRB (blood-retina barrier) component cells to form the neurovascular unit (NVU), but also play important roles in innate immunity (3). Previous studies demonstrated that microglia and Müller cells are activated early in the course of DR and remained active throughout the whole stage of DR, thereby triggering and aggravating an inflammatory retinal environment and neurodegeneration (4,5). Additionally, microglia and Müller cells are inextricably linked and reliant on one another. The energy-intensive processes carried out by microglia are fueled by adenosine triphosphate (ATP) supplied by nearby Müller cells (6). In response to hyperglycemia, Müller cells produce MCP-1 which activates and recruits microglia (7). Activated microglia can stimulate the activation of Müller cells, which in turn secrete a number of mediators that reciprocally activate microglia (8), indicating a feedback loop that perpetuates activations of microglia and Müller cells.
Nuclear factor-κB (NF-κB) is a pivotal transcription factor associated with metabolic disorders including obesity, atherosclerosis and diabetes (9,10). NF-κB exists as an inactive form in the cytoplasm by binding to the inhibitor of κB (IκB). Various stimuli (pathogen, cytokines, stress, free radicals, etc.) can trigger the degradation of IκB through activation of IκB kinases (IKKs), allowing the release of NF-κB from IκB. Once released, free NF-κB translocates to the nucleus, where it interacts with the promoter of specific genes, initiating the inflammatory and metabolic responses (11,12). The IKK complex consists of IKK-α, IKK-β, and the regulatory subunit IKK-γ (13). N-(3,5-bis-trifluoromethyl-phenyl)-5-chloro-2-hydroxy-benzamide (IMD-0354) is a novel IKKβ inhibitor with high selectivity. It has exhibited therapeutic effects on various disease models in different organs, including the eye (14). IMD-0354 has proven to attenuate uveoretinitis, suppress corneal inflammation and angiogenesis, and restore the barrier function of human retinal endothelial cells (HRECs) (14-17).
This study aimed to investigate the potential of selective IKKβ inhibition as an anti-inflammation, anti-glial cell activation, and cytoprotective therapy in a rat model of streptozotocin (STZ)-induced DR. We present the following article in accordance with the ARRIVE reporting checklist (available at https://atm.amegroups.com/article/view/10.21037/atm-22-2204/rc).
Methods
Rat model of diabetes
The approval of animal experiments was acquired from the Animal Ethics Committee of the Eye and ENT Hospital of Fudan University (Shanghai, China) (No. 2020142). In this study, all the procedures were carried out based on the ARVO Statement for the Use of Animals in Ophthalmic and Vision Research.
Adult male Sprague Dawley (SD) rats [approximately 200 g, animal production license No. SCXK(Shanghai) 2012‑0002] were kept under a 12‑h light/12‑h dark cycle with free access to food and water. In a random manner, totally 96 rats were randomly assigned to one of the four groups: (I) normal control, (n=22), (II) normal + IMD-0354, (n=22), (III) diabetic group, (n=26), or (IV) diabetic + IMD-0354, (n=26). Rats in the diabetic group were injected STZ (Streptozocin) intraperitoneally (60 mg/kg, S0130, Sigma-Aldrich, Billerica, MA, USA). Normal control rats referred to wild-type ones devoid of any injury, which were administered with sodium citrate buffer vehicle (pH 4.6, Sigma-Aldrich, Billerica) at an equivalent volume. At 24, 48 and 72 hours following STZ provision, a glucometer (Precision PC; Medic, Cambridge, UK) was utilized to examine the blood glucose levels. Rats were deemed diabetic when the levels of blood glucose in triplicate consecutive assessments exceeded 16.7 mmol/L.
IMD-0354 injection
IMD-0354 (Tocris 2611, Bristol, UK) was dissolved in 4% dimethyl sulfoxide (DMSO) (Sigma-Aldrich) immediately before the usage. Rats in the diabetic + IMD-0354 group were administrated IMD-0354 (30 mg/kg) intraperitoneally from week 6 of STZ injection for 6 consecutive weeks through the termination of the experiment. Rats in the control + IMD-0354 group were also given IMD-0354 (30 mg/kg) for six consecutive weeks, in order to assess the drug’s safety and potential side effects (1). Normal control and one group of diabetic rats received the same volume of 4% DMSO in phosphate-buffered saline (PBS; Gibco, Grand Island, NY, USA) at each time point. Under ketamine (80 mg/kg)- and xylazine (10 mg/kg)-induced anesthesia, the rats were killed by cervical dislocation, and then ocular samples were gathered for terminal deoxynucleotidyl transferase dUTP nick end labeling (TUNEL), western blot, as well as immunofluorescence assessments.
Oxidative stress assays
Rat retinas were extracted immediately after animals were sacrificed. Saline (0.9%) was utilized to gather the samples on ice as homogenates (10%). Following a 10-minute centrifugation of homogenates at 2,000 rpm, the supernatants were gathered and diluted. Biomarkers of oxidative stress like superoxide dismutase (SOD) (Cat. No. A001-1), catalase (CAT) (Cat. No. A007-1), malonic dialdehyde (MDA) (Cat. No. A003-1), GSH (Cat. No. A061-1) and glutathione peroxidase (GSH-Px) (Cat. No. A005) were examined as per the instructions of commercial kits from Jiancheng Bioengineering Institute (Nanjing, China).
TUNEL
Eyeballs were collected and immobilized for 1 h in paraformaldehyde (PFA; 4%). The anterior segment was dissected, which included cornea, lens and iris, while the residual eyecup was immobilized overnight in PFA (4%) at 4 ℃, and subsequently dehydrated for approximately 3 hours with sucrose (30%; S1888, Sigma‑Aldrich) and embedded using optimal cutting temperature compound (Tissue Tek, Sakura, Japan). A Leica microtome (Germany) was employed to cut the serial cryosections into a 10-µm thickness, and then adhesion microscope slides (Citoglas, Taizhou, China) were utilized for their mounting. Dried sections were cryopreserved at −80 ℃ until usage. With the aid of TUNEL‑based in situ Cell Death Detection Kit (Roche, Nutley, USA), the cellular apoptosis on cryosections was assayed. A laser confocal microscope (Leica Microsystems, Bensheim, Germany) was applied to stochastically select 9 fields from every retina. For the apoptosis assessment, the ratio of TUNEL‑positive cell counts to the overall cell counts was computed.
Immunofluorescence and quantification of cells
Frozen sections were nurtured with 5% goat serum and 0.3% Triton X-100 in PBS at room temperature for 1 h and later incubated with rabbit anti-glial fibrillary acidic protein (GFAP) antibody (ab62428, 1:100, Abcam) for Müller cells at 4 ℃ overnight. Negative control used was the primary antibody-free sections. Following thrice PBS washing, the sections were subjected to a 1-h incubation using proper secondary antibodies under ambient temperature and dark conditions, and subsequently washed extensively, cover-slipped and assessed via a laser confocal microscope (Leica Microsystems). The Iba1-, Brn3a- and GFAP-positive cells were quantified on 9 fields selected stochastically from every retina. After anesthesia, rats were sequentially perfused in saline and PFA (4%) transcardially. Their right eyes were enucleated and immobilized for 1 h in PFA (4%) at 4 ℃. Next step was isolation of whole retinas, their 30-min placement in cold Triton X-100 (0.3%), and 1-h blockade using donkey serum (10%; Jackson Immuno Research Laboratories, West Grove, USA) involving Tween-20 (0.05%; Sigma-Aldrich) and bovine serum albumin (1%) at ambient temperature. Overnight incubation of the whole retinas proceeded using primary rabbit polyclonal anti-Brn3a (ab53025, 1:100, Abcam) antibody in RGCs and rabbit anti-Iba1 (ab245230, 1:100, Abcam) antibody in the case of microglia at 4 ℃. For the immunoreactive protein visualization, incubation was accomplished with the 1:1,000 dilution of goat anti-rabbit secondary antibody (Thermo Fisher Scientific, Waltham, USA). A laser scanning microscope (LSM) 510 META confocal laser-scanning microscope (Zeiss, Jena, Germany) was applied for scanning and capturing the immunofluorescent images. Activated microglia and RGCs were quantified in areas of almost identical distances of 1/6, 3/6 and 5/6 retinal radii from the optic disc in every quadrant. Four computations were made on every retinal piece at each distance under a ×200 magnification. An automated system Image-Pro Plus (Ver. 6.0, Media Cybernetics, Silver Spring, USA) was utilized for analyzing the images. Results were presented as the mean densities of microglial cells and RGCs at a specific zone of every retina.
Measurement of nuclear NF-κB-p65 levels and cytokine concentrations in the retina
Retinas dissected from rats were homogenized by ultrasound. Enzyme-linked immunosorbent assay kits (ELISA) (Novusbio, Colorado, CO or R&D Systems, Minneapolis, MN, USA) were adopted for measuring concentrations of tumor necrosis factor α (TNF-α), interleukin 1β (IL-1β), interleukin 6 (IL-6), interleukin 10 (IL-10), monocyte chemoattractant protein 1 (MCP-1) and vascular endothelial growth factor (VEGF) in the retina.
Culture of primary rat retinal microglia and Müller cells
From neonatal SD rats, we isolated the primary rat retinal microglia, as well as Müller cells. Following euthanasia, ocular enucleation and anterior segment elimination, the murine retina was harvested and digested using trypsin (0.25%; Gibco) at 37 ℃. Five min later, trypsin was deactivated using FBS (20%; Gibco)-involving DMEM/F12 (Gibco) supplemented with penicillin/streptomycin (1%; Sigma-Aldrich). A 5-min centrifugation proceeded at 1,200 rpm. Then, the cells were shifted into a sterile centrifuge tube and resuspended in the prewarmed culture media (10% FBS-involving DMEM/F12; 8–10 mL), followed by transfer into a gelatin-based solution-precoated T75 flask. We placed the flasks in a humidified 37 ℃ incubator with 5% CO2. On the 3rd day, replacement of culture media was accomplished for the non-adherent cell elimination and nutrient replenishment. Subsequently, media were replaced at 2-day intervals for the acquisition and purification of Müller cells. Upon confluency of the mixed cells, the flasks were shaken mechanically for eliminating loosely attached primary microglia. Müller glia accounted for 90% of the residual cells, which were attached firmly to the bottom. Müller cells were made purer through twice subcultivation. We did not replace the culture media until 12 days thereafter, in order to acquire and purify microglial cells. The microglia-containing supernatant was gathered and centrifuged, and then the cells were resuspended at proper density. According to our prior work (18), the isolated microglia had >93% purity.
Treatment of cells
Purified microglia and Müller cells were seeded on coverslips in 24-well plates. Cells were incubated in media containing either normal (5 mM) or high (20 mM) concentration of glucose for 24 h. Subgroups of cells were subject to treatment with or without 1 µg/mL IMD-0354 for 1 h.
Western blot
Retinal microglia and Müller cells were lysed in RIPA buffer (Beyotime Institute Biotechnology, China). Cytoplasmic and nuclear protein of microglia/Müller cells were separated by protein extraction kit (Pierce Biotechnology, Rockford, IL, USA) for the detection of cytoplasmic and nuclear NF-κB-p65. Protein concentrations were measured with a BCA (bicinchoninic acid) Protein Assay Kit (Beyotime, Shanghai, China). Equal amounts of protein were fractionated by sodium dodecyl sulfate-polyacrylamide gel electrophoresis (SDS-PAGE; Beyotime) and transferred to polyvinylidene difluoride membranes (Millipore, Billerica, MA, USA). Following a 1-h blockade of membranes using 5% skimmed milk in TBS involving Tween‑20 (Sigma-Aldrich), incubation proceeded using each 1:1,000 dilution of primary antibodies against IL‑1β (ab9722, Abcam), TNF-α (ab6671, Abcam), VEGF (ab39638, Abcam), IL-6 (ab9324, Abcam), NF-κB-p65 (ab16502, Abcam), IL-10 (ab192271, Abcam), as well as nuclear factor-κB-p65 (Santa Cruz Biotechnology). Each 1:1,000 dilution of Anti-β-actin (ab8226, Abcam) and Anti-HDAC1 (ab19845, Abcam) were adopted as the loading controls separately for cytoplasmic and nuclear proteins. After thrice washing, an extra 2-h incubation of the membranes proceeded at ambient temperature using the corresponding secondary antibodies. An enhanced chemiluminescence kit was utilized to assay the expression of proteins. With the aid of ImageQuant TL v. 7.0 (GE Healthcare, Piscataway, NJ, USA), the intensity quantification was accomplished on every band. Assaying of the entire samples was triplicated.
Measurement of cytokine concentrations in cell culture supernatants
Cell-conditioned media were collected and subjected to centrifugation to remove debris. ELISA (R&D Systems) were used to measure the concentration of TNF-α, IL-1β, IL-6, and IL-10 in the centrifuged supernatants (50 µL). During the whole stage of the experiment, the researchers (XD and ZS) were aware of the group allocation, the conduct of experiment, the measurement outcome and the data analysis.
Statistical analysis
The data were presented as means ± standard error (SE) of at least three independent experiments. Differences between groups were evaluated using unpaired one-way ANOVA and LSD post-hoc tests (SPSS software, version 21.0, Chicago, IL, USA). A P value of 0.01 or less was considered statistically significant.
Results
IMD-0354 reverses elevated levels of NF-κB-p65 in the retina of diabetic rats
Western blot-based measurements showed that levels of nuclear NF-κB-p65 were markedly increased in the retina of diabetic rats as compared to the control rats (Figure 1A). Systemic administration of IMD-0354 for 6 weeks significantly reducedNF-κB-p65 levels in the retina of diabetic rats, whereas normal rats were not affected. Data are shown as the mean ± standard deviation (SD) (Figure 1B). These data suggest that IMD-0354 treatment could effectively penetrate the blood-retinal barrier and inhibit NF-κB activity in the diabetic retina.
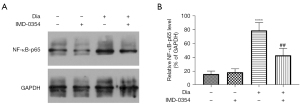
IMD-0354 inhibits activation of microglia and Müller cells in diabetic rats.
Activation of microglia and Müller cells were evaluated by immunostaining of GFAP and Iba1, respectively. As shown in Figures 2,3, both the numbers of Iba1-positive and GFAP-positive cells, as well as the levels of Iba1and GFAP expression, were significantly increased in the retina of diabetic rats when compared to the control rats. Iba1-positive cells were primarily found in the inner plexiform layer (IPL) and outer plexiform layer (OPL) in the control retinas. In contrast, Iba1-expressing cells were observed in the ganglion cell layer (GCL) in the retina of diabetic rats, suggesting that microglial cells migrate to the inner layers of the retina under diabetic conditions. After injection of IMD-0354 for 6 weeks, both Iba1- and GFAP-positive signals were greatly reduced. Although the numbers of Iba1-positive and GFAP-positive cells were markedly decreased, Iba1-positive cells are still visible in the GCL.
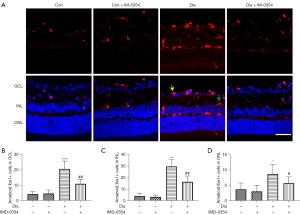
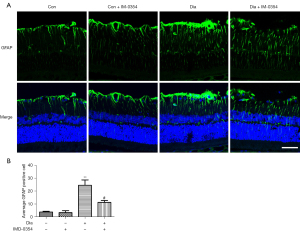
IMD-0354 attenuates oxidative injury and exerts antioxidative effects in the retina of diabetic rats
To evaluate potential antioxidant effects of IMD-0354 on the retina, we measured activities of SOD, GSH-Px and CAT. Results showed that levels of SOD, GSH-Px and CAT were remarkably lower in the diabetic group compared to the non-diabetic control group. Treatment with consecutive IMD-0354 injection for 6 weeks led to significant increases in SOD, GSH-Px and CAT levels (Figure 4A-4C). Oxidative damage in the retina was assessed by levels of MDA. The levels of MDA were significantly higher in the diabetic group compared to the non-diabetic control group. Administration of IMD-0354 in diabetic rats significantly dropped the MDA levels (Figure 4D).
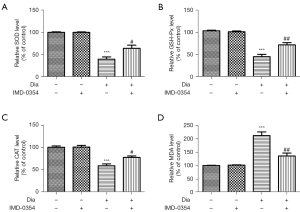
IMD-0354 protects retinal neurons from apoptosis in diabetic rats
Neuron degeneration in the retina was estimated by TUNEL assay. As shown in Figure 5A, TUNEL‑positive cells in the retina were dramatically increased in the ganglion cell layer (GCL), inner nuclear layer (INL) and outer nuclear cell (ONL) in diabetic rats, indicative of diffuse neuron apoptosis. Administration with IMD-0354 remarkably reduced, but did not completely eliminate, nerve cell apoptosis in the retina. TUNEL-positive cells were still observable in GCL, INL and ONL (Figure 5). In the diabetic rats, the density of RGCs labelled by Brn3a significantly decreased in the retina, as compared to the control animals. After consecutive injection with IMD-0354 for 6 weeks, the rates of RGCs loss in diabetic rats were significantly alleviated (Figure 6).
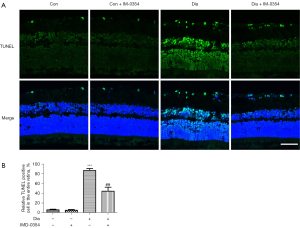
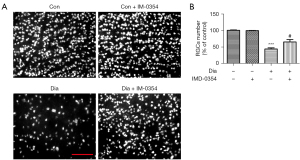
IMD-0354 decreases production of VEGF and inflammatory cytokines and increases secretion of IL-10 in the retina of diabetic rats
Effects of IMD-0354 on the expression of VEGF, inflammatory cytokines and anti-inflammatory cytokines in the retina of diabetic rats were evaluated by ELISA. Levels of IL-1β, MCP-1, IL-6, TNF-α and VEGF, in the retina were remarkably elevated in diabetic rats compared to the control animals (Figure 7A-7E). IMD-0354 significantly inhibited secretion of VEGF and inflammatory cytokines under high glucose conditions. By contrast, anti-inflammatory cytokine IL-10 was greatly reduced in diabetic rats, and IMD-0354 treatment significantly increased IL-10 in the retina (Figure 7F).
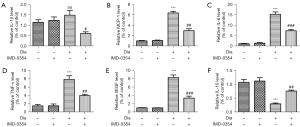
Nuclear translocation of NF-κB-p65 in retinal glial cells is induced by high glucose and reversed by IMD-0354
Effects of IMD-0354 on NF-κB activity in retinal glial cells were assessed by western blot with anti NFκ-p65 and anti-nuclear NFκ-p65 antibody. High glucose promoted NF-κB-p65 expression as well as its nuclear translocation (Figure 8, microglia; Figure 9, Müller cell). Inhibition of IKK2 by IMD-0354 downregulated the expression of NF-κB-p65 and blocked its nuclear translocation in response to high glucose, whereas the cytoplasmic levels of NF-κB-p65 were unaffected.
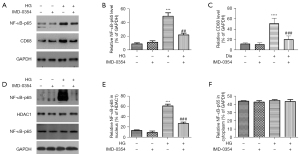
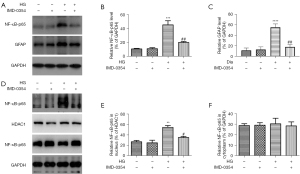
IMD-0354 diminishes inflammatory reactions and promotes anti-inflammatory reactions in retinal glial cells under high glucose conditions
ELISA analysis showed that high glucose treatment strongly upregulated expression of TNF-α, IL-1β and IL-6, and downregulated IL-10 expression in microglia and Müller cells. IMD-0354 treatment significantly decreased expression of the inflammatory cytokines (TNF-α, IL-1β, IL-6) and increased expression of anti-inflammatory IL-10 in both microglia (Figure 10A-10D) and Muller cells (Figure 11A-11D), suggesting that blockade of IKK2 by IMD-0354 treatment alleviates activation of microglia and Müller cells and the subsequent inflammatory responses.
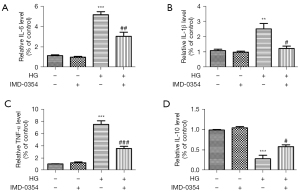
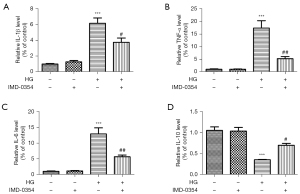
Discussion
In this study, we demonstrated that IKKβ inhibitor IMD-0354 effectively blocks nuclear translocation of NF-κB-p65 in vitro in high glucose-treated retinal glial cells and in vivo in the retina of diabetic rats. IMD-0354 exhibits therapeutic effects on oxidative injury, glial cell activation, inflammation, VEGF downregulation and neuron protection in a rat model of STZ-induced DR.
NF-κB is a ubiquitously expressed transcription factor that plays a key role in inflammation, immunity, proliferation, and cell death. Upon activation, NF-kB transports to nucleus where it binds to the promoter region of hundreds of genes and regulates gene expression. Pro-inflammatory cytokines are not only regulated by NF-κB, but also induce the activation of NF-κB itself, forming a positive feedback loop. Additionally, NF-κB signaling pathway is responsible for cell apoptosis, VEGF production, glial cell activation and oxidative stress tolerance (19-23). In our study, the total amount of NF-κB-p65 was markedly elevated in high glucose-treated microglia and Müller cells in the retina of diabetic rats compared to the control rats. In addition, we found that it is the increased nuclear NF-κB-p65expressionmainly contributes to the enhancement of total NF-κB-p65 expression, since the expression of cytoplasmic NF-κB-p65 remains unaffected. These findings suggest that NF-κB activation and nuclear translocation are important pathophysiological events under hyperglycemic condition and are major contributors in the inflammatory responses of DR. Thus, disruption of NF-κB signaling may suggest a potential strategy against DR (24).
IMD-0354 was originally developed to prevent the nuclear translocation of NF-κB by competitive interfering with the access of ATP to its docking site on IKKβ. Unlike other NF-κB inhibitors, IMD-0354 functions independent of comprehensive disruption of the NF-κB pathway, as IKK-α remains capable of activating NF-κB. Therefore, IMD-0354 may be a more multifaceted and safer suppressor of inflammatory disorder in the eye. Although previous studies reported that IMD-0354 may induce cell apoptosis and reduce proliferation of cancer cells (25,26), a recent study showed that IMD-0354 has no significant adverse effects in a variety of ocular disease models, both in vivo and in vitro.
Microglia and Müller cells play important roles in the regulation of retinal innate immunity (5). Previous studies have demonstrated that microglia and Müller cells are activated early in DR, triggering and aggravating inflammatory responses and neurodegeneration (27-29). In our study, we demonstrated that NF-κB activation is a key pathway involved inactivation of microglia and Müller cells. IMD-0354 reversed nuclear translocation of NF-κB-p65 in high glucose-treated glial cells by blocking NF-κB signaling, inhibiting activation and inflammatory responses of microglia and Müller cells. Importantly, IMD-0354 downregulated the level of nuclear NF-κb-p65, attenuated oxidative injury, decreased expression of inflammatory cytokines (TNF-α, IL-1β, IL-6), promoted production of anti-inflammatory cytokine IL-10, and protected neurons (RGC and photoreceptors) from apoptosis in the retina of diabetic rats. IMD-0354 also significantly decreased VEGF overexpression in the retina of diabetic rats. Consistent with our findings, a previous study demonstrated that systemic administration of IMD-0354 was effective in preventing DR progression while preserving the vascular integrity (30).
However, further research is needed to elucidate the mechanism by which IMD-0345 affects glial activity and cytokine and growth factor release. Additionally, the crosstalk between microglial and Müller cells enables the diversity and plasticity of glia to exhibit diverse functional phenotypes in response to their microenvironment. Further investigation of the effect of IMD-0345 on microglia and Müller cells in a STZ-induced rat model of DR is warranted.
Conclusions
In summary, NF-κB activation is a key step in the development of DR. Prevention of NF-κB nuclear translocation through inhibition of IKKβattenuates oxidative injury, glial cell activation, VEGF secretion and inflammatory responses in the retina of diabetic rats, and protects neurons from apoptosis. Thus, suppression of NF-κB signaling by IMD-0354 may represent a promising therapeutic strategy against DR through various mechanisms.
Acknowledgments
Funding: This work was supported by the Youth Project of the National Natural Science Fund (Nos. 82101150, 82101149), Shanghai Clinical Three-Year Action Plan-Major Clinical Research Project (No. SHDC2020CR2041B to GX), Shanghai Municipal Health Committee (No. 20204Y0056 to XD), Shanghai Sailing Program (No. 22YF1406000).
Footnote
Reporting Checklist: The authors have completed the ARRIVE reporting checklist. Available at https://atm.amegroups.com/article/view/10.21037/atm-22-2204/rc
Data Sharing Statement: Available at https://atm.amegroups.com/article/view/10.21037/atm-22-2204/dss
Peer Review File: Available at https://atm.amegroups.com/article/view/10.21037/atm-22-2204/prf
Conflicts of Interest: All authors have completed the ICMJE uniform disclosure form (available at https://atm.amegroups.com/article/view/10.21037/atm-22-2204/coif). The authors have no conflicts of interest to declare.
Ethical Statement: The authors are accountable for all aspects of the work in ensuring that questions related to the accuracy or integrity of any part of the work are appropriately investigated and resolved. Animal experiments were approved by the Animal Ethics Committee of the Eye and ENT Hospital of Fudan University (Shanghai, China) (No. 2020142). All procedures were conducted in accordance with the ARVO Statement for the Use of Animals in Ophthalmic and Vision Research.
Open Access Statement: This is an Open Access article distributed in accordance with the Creative Commons Attribution-NonCommercial-NoDerivs 4.0 International License (CC BY-NC-ND 4.0), which permits the non-commercial replication and distribution of the article with the strict proviso that no changes or edits are made and the original work is properly cited (including links to both the formal publication through the relevant DOI and the license). See: https://creativecommons.org/licenses/by-nc-nd/4.0/.
References
- Ren J, Zhang S, Pan Y, et al. Diabetic retinopathy: Involved cells, biomarkers, and treatments. Front Pharmacol 2022;13:953691. [Crossref] [PubMed]
- Rolev KD, Shu XS, Ying Y. Targeted pharmacotherapy against neurodegeneration and neuroinflammation in early diabetic retinopathy. Neuropharmacology 2021;187:108498. [Crossref] [PubMed]
- Pan WW, Lin F, Fort PE. The innate immune system in diabetic retinopathy. Prog Retin Eye Res 2021;84:100940. [Crossref] [PubMed]
- Carpi-Santos R, de Melo Reis RA, Gomes FCA, et al. Contribution of Müller Cells in the Diabetic Retinopathy Development: Focus on Oxidative Stress and Inflammation. Antioxidants (Basel) 2022;11:617. [Crossref] [PubMed]
- Wu H, Wang M, Li X, et al. The Metaflammatory and Immunometabolic Role of Macrophages and Microglia in Diabetic Retinopathy. Hum Cell 2021;34:1617-28. [Crossref] [PubMed]
- Wang M, Wong WT. Microglia-Müller cell interactions in the retina. Adv Exp Med Biol 2014;801:333-8. [Crossref] [PubMed]
- Davies MH, Stempel AJ, Powers MR. MCP-1 deficiency delays regression of pathologic retinal neovascularization in a model of ischemic retinopathy. Invest Ophthalmol Vis Sci 2008;49:4195-202. [Crossref] [PubMed]
- Wang M, Ma W, Zhao L, et al. Adaptive Müller cell responses to microglial activation mediate neuroprotection and coordinate inflammation in the retina. J Neuroinflammation 2011;8:173. [Crossref] [PubMed]
- Capece D, Verzella D, Flati I, et al. NF-κB: blending metabolism, immunity, and inflammation. Trends Immunol 2022;43:757-75. [Crossref] [PubMed]
- Sahukari R, Punabaka J, Bhasha S, et al. Plant Compounds for the Treatment of Diabetes, a Metabolic Disorder: NF-κB as a Therapeutic Target. Curr Pharm Des 2020;26:4955-69. [Crossref] [PubMed]
- Songkiatisak P, Rahman SMT, Aqdas M, et al. NF-κB, a culprit of both inflamm-ageing and declining immunity? Immun Ageing 2022;19:20. [Crossref] [PubMed]
- Roberti A, Chaffey LE, Greaves DR. NF-κB Signaling and Inflammation-Drug Repurposing to Treat Inflammatory Disorders? Biology (Basel) 2022;11:372. [Crossref] [PubMed]
- Israël A. The IKK complex, a central regulator of NF-kappaB activation. Cold Spring Harb Perspect Biol 2010;2:a000158. [Crossref] [PubMed]
- Liu Y, Kitaichi N, Wu D, et al. Attenuation of experimental autoimmune uveoretinitis in mice by IKKβ inhibitor IMD-0354. Biochem Biophys Res Commun 2020;525:589-94. [Crossref] [PubMed]
- Lennikov A, Mirabelli P, Mukwaya A, et al. Selective IKK2 inhibitor IMD0354 disrupts NF-κB signaling to suppress corneal inflammation and angiogenesis. Angiogenesis 2018;21:267-85. [Crossref] [PubMed]
- Lennikov A, Kitaichi N, Noda K, et al. Amelioration of endotoxin-induced uveitis treated with an IκB kinase β inhibitor in rats. Mol Vis 2012;18:2586-97. [PubMed]
- Lennikov A, Mukwaya A, Fan L, et al. Synergistic interactions of PlGF and VEGF contribute to blood-retinal barrier breakdown through canonical NFκB activation. Exp Cell Res 2020;397:112347. [Crossref] [PubMed]
- Ding X, Gu R, Zhang M, et al. Microglia enhanced the angiogenesis, migration and proliferation of co-cultured RMECs. BMC Ophthalmol 2018;18:249. [Crossref] [PubMed]
- Lv F, Yang L, Wang J, et al. Inhibition of TNFR1 Attenuates LPS Induced Apoptosis and Inflammation in Human Nucleus Pulposus Cells by Regulating the NF-KB and MAPK Signalling Pathway. Neurochem Res 2021;46:1390-9. [Crossref] [PubMed]
- Novotny NM, Markel TA, Crisostomo PR, et al. Differential IL-6 and VEGF secretion in adult and neonatal mesenchymal stem cells: role of NFkB. Cytokine 2008;43:215-9. [Crossref] [PubMed]
- Feng N, Jia Y, Huang X. Exosomes from adipose-derived stem cells alleviate neural injury caused by microglia activation via suppressing NF-kB and MAPK pathway. J Neuroimmunol 2019;334:576996. [Crossref] [PubMed]
- Ontko CD, Capozzi ME, Kim MJ, et al. Cytochrome P450-epoxygenated fatty acids inhibit Müller glial inflammation. Sci Rep 2021;11:9677. Erratum in: Sci Rep 2021;11:18816. [Crossref] [PubMed]
- Ding S, Wang C, Wang W, et al. Autocrine S100B in astrocytes promotes VEGF-dependent inflammation and oxidative stress and causes impaired neuroprotection. Cell Biol Toxicol 2021; Epub ahead of print. [Crossref] [PubMed]
- Yin Y, Chen F, Wang W, et al. Resolvin D1 inhibits inflammatory response in STZ-induced diabetic retinopathy rats: Possible involvement of NLRP3 inflammasome and NF-κB signaling pathway. Mol Vis 2017;23:242-50. [PubMed]
- Tanaka A, Konno M, Muto S, et al. A novel NF-kappaB inhibitor, IMD-0354, suppresses neoplastic proliferation of human mast cells with constitutively activated c-kit receptors. Blood 2005;105:2324-31. [Crossref] [PubMed]
- Feng Y, Pathria G, Heynen-Genel S, et al. Identification and Characterization of IMD-0354 as a Glutamine Carrier Protein Inhibitor in Melanoma. Mol Cancer Ther 2021;20:816-32. [Crossref] [PubMed]
- Mills SA, Jobling AI, Dixon MA, et al. Fractalkine-induced microglial vasoregulation occurs within the retina and is altered early in diabetic retinopathy. Proc Natl Acad Sci U S A 2021;118:e2112561118. [Crossref] [PubMed]
- Ji L, Tian H, Webster KA, et al. Neurovascular regulation in diabetic retinopathy and emerging therapies. Cell Mol Life Sci 2021;78:5977-85. [Crossref] [PubMed]
- Le YZ, Xu B, Chucair-Elliott AJ, et al. VEGF Mediates Retinal Müller Cell Viability and Neuroprotection through BDNF in Diabetes. Biomolecules 2021;11:712. [Crossref] [PubMed]
- Lennikov A, Hiraoka M, Abe A, et al. IκB kinase-β inhibitor IMD-0354 beneficially suppresses retinal vascular permeability in streptozotocin-induced diabetic mice. Invest Ophthalmol Vis Sci 2014;55:6365-73. [Crossref] [PubMed]