Combination strategies incorporating oncolytic viruses and immune checkpoint inhibitors for advanced melanoma: what is the evidence?
Malignant melanoma is the most aggressive form of skin cancer and its incidence has increased in the last decade (1). Melanoma is considered an immunogenic tumour type based on criteria such as high tumour mutation burden, higher prevalence in immunocompromised patients and its clear response to immunotherapy. Surgical treatment alone successfully treats the vast majority of early-stage melanomas, however surgery is less effective, or even inappropriate, for advanced-stage or metastatic melanoma. In such cases, systemic treatments, such as targeted therapy, immunotherapy and, less frequently, chemotherapy are the cornerstone of patient management. Immunotherapy offers the greatest opportunity for durable tumour control in advanced melanoma (2) by using elements of the patient’s own innate and adaptive immune system to target cancer cells.
Immune checkpoint inhibitors (ICIs) have revolutionised the management of a range of cancers in recent years, with their greatest successes to date being seen in treating melanoma. ICIs are monoclonal antibodies that target immune checkpoint molecules, such as CTLA-4 and programmed death-1 (PD-1), which are co-inhibitory molecules expressed most prominently on the membrane surface lymphocytes. Since 2010, the Food and Drug Administration (FDA) has approved both anti-CLTA-4 (ipilimumab) and anti-PD-1 (nivolumab, pembrolizumab) antibodies for treating advanced melanoma. Patients can be treated with combination (ipilimumab plus nivolumab) or single-agent (anti-CTLA-4 or anti-PD-1) therapy (3-5). Pembrolizumab has shown response rates around 38% in patients with metastatic melanoma, including in those with progressive disease after treatment with ipilimumab, and an overall survival (OS) of 74% at 12 months (6). Clinical benefit of PD-1 administration has been correlated with a marked expansion/increase of tumour-infiltrating CD8+ T cells.
Even though ICIs are transformative cancer therapies and offer therapeutic benefits for patients with advanced melanoma, in a significant subset of patients the tumour will display either intrinsic or acquired resistance to these drugs, usually accompanied with a lack of sufficient T cell inflammation and resistance to ICI therapy (7). These limitations have driven the development of combination strategies between ICIs and other types of immunotherapies, including with oncolytic viruses (OVs). It is the hope that such combinations will enhance the steps of spontaneous T cell activation and immune cell infiltration into tumours.
OVs are a novel class of cancer immunotherapy (8). They can be naturally occurring or genetically-modified, but share the property of preferentially replicating in and killing tumour cells. There are several DNA and RNA viruses which have been exploited as OVs in translational cancer research: for instance, reoviruses, herpes simplex viruses (HSV) and adenoviruses (9,10). The therapeutic efficacy of OVs extends to their modulation of the tumour-immune microenvironment (TIME) to reverse the immunosuppressive milieu of tumours and, thereby, sensitise them to tumour-specific lymphocytes and ICIs (11). Importantly, a variety of genes can be inserted into the genome of OVs to enhance their therapeutic efficacy; for example, transgenes expressing cytokines or recombinant ICI antibodies. Such viral genome engineering can improve local and systemic anti-tumour immunity and reduce the emergence of treatment resistance (12).
Talimogene laherparepvec (T-VEC) has been approved by multiple regulatory agencies for the treatment of advanced melanoma. T-VEC is a genetically-modified HSV type 1 that encodes granulocyte-macrophage colony-stimulating factor (GM-CSF). The chemokine facilitates priming of tumour-specific lymphocytes and infiltration of immune cells into the tumour site. Thus, it enhances anti-tumour immunity and induces a systemic immune response (13). Additionally, T-VEC contains deletions of the infected cell proteins (ICPs) 34.5 and 47, which allow the virus selectively to infect tumour cells (14) and promote antigen presentation in infected tumour cells (15), respectively.
Based on the data from the randomised phase III OPTiM trial (NCT00769704) in patients with unresectable stage IIIB–IVM1c melanoma, intralesional administration of T-VEC was shown to improve response rate compared with subcutaneous GM-CSF alone [durable response rate (DRR) 16.3 vs. 2.1%; overall response rate 26.4% vs. 5.7%]. Although adverse events associated with T-VEC have been reported (e.g., fatigue, chills, and pyrexia), the agent is well tolerated with low levels of grade 3–5 toxicity (16). Subsequent follow-up analyses reported median OS of 23.3 and 18.9 months for T-VEC and GM-CSF, respectively. Updated overall response rates were 31.5% (T-VEC) and 6.4% (GM-CSF), while complete responses were 16.9% (50 patients from T-VEC group) and 0.7% (1 patient from GM-CSF group). The benefits of T-VEC were most pronounced in the patients with stage IIIB, IIIC, and IVM1a compared to more advanced metastatic stages (17). Despite this successful phase III trial, single-agent T-VEC therapy has achieved limited market penetration and has established little more than a niche role in the treatment of melanoma, largely because of the dominant positions assumed by ICIs and BRAF/MEK inhibitors. T-VEC, and other OVs, have been the subject of further studies in combination with ICIs in an attempt to exploit their roles as relatively non-toxic TIME-modulating therapies.
A phase Ib study (MASTERKEY-265; NCT02263508) examined 21 patients with unresectable stage IIIB–IVM1c melanoma who were treated with the combination of T-VEC and pembrolizumab. Patients received intratumoral injections of 1×106 plaque-forming units (pfu) per mL of T-VEC on day 1 of week 1 and subsequent doses of 1×108 pfu/mL were injected on day 1 of week 4 and 6, and every 2 weeks thereafter. Subsequently, a flat dose of 200 mg of pembrolizumab was infused intravenously every 2 weeks commencing on day 1 of week 6 (together with the third dose of T-VEC). The combined treatment resulted in a high overall response rate of 62% and complete response rate (CRR) of 33% in advanced melanoma patients. Additionally, the combination of T-VEC and pembrolizumab did not increase adverse events compared to historical controls for pembrolizumab alone. Most importantly, after combination treatment, post-treatment tumour biopsies were shown to have an increased density of CD8+ T cells, programmed death-ligand 1 (PD-L1) and interferon-γ (IFN-γ) expression (18).
These clinical data provided proof-of-principle for T-VEC inducing immunogenic cell death in tumour cells, causing the release of viral antigens, danger-associated molecular patterns (DAMPs), pathogen-associated molecular patterns (PAMPs) and, thereby, promoting IFN-γ signalling. Such T-VEC-induced IFN-γ signalling, together with virus-mediated local GM-CSF production leads to infiltration and activation of antigen-presenting cells (APCs) in the TIME. The APCs expressing ingested tumour antigen can then prime and activate CD8+ T cells in the tumour-draining lymph nodes. The impact of T-VEC in increasing the influx of tumour-specific lymphocytes into the TIME can alter the state of tumours from immunologically cold to hot. This phenotypic change within a tumour can, in turn, improve tumour responsiveness to ICI therapy.
Further characterisation of the ways that T-VEC was distributed within tumours and influenced the tumour microenvironment in the MASTERKEY-265 trial potentially could have revealed mechanistic insight into induction of stimulatory events in relevant cell sub-populations within the tumour microenvironment, similar to the analysis of T-VEC-treated lymphoma samples in the study by Ramelyte et al. (19). Single-cell analysis could have provided insight into which immune pathways are functionally relevant in distinct patient populations and, thus, might have informed the optimisation of combination trials.
Following the initial phase Ib MASTERKEY-265 trial, Chesney et al. (20) completed the main phase III randomised, double-blind, placebo-controlled trial. Six hundred ninety-two patients with advanced melanoma (stage IIIB–IVM1c) were randomly divided into 346 patients in each of two groups (pembrolizumab plus T-VEC vs. pembrolizumab plus placebo). In this component of the study, the schedule was altered and T-VEC and pembrolizumab were administrated concurrently from day 1 of cycle 1. As before, the initial dose level was up to 4 mL of 1×106 pfu/mL, with subsequent doses at 1×108 pfu/mL initially 2-weekly and, then, beyond cycle 5 given 3-weekly to align with the standard administration schedule of flat-dosing of 200 mg of pembrolizumab. Primary endpoints of the study were progression-free survival (PFS) and OS. Ultimately, the study reported that the T-VEC/pembrolizumab combination did not significantly improve PFS or OS compared to the placebo group. Notably, objective response rate (ORR) and DRR of T-VEC/pembrolizumab group were 48.6% and 42.2%, respectively, while those for placebo were 41.3% and 34.1%, respectively. Even though both ORR and DRR were numerically higher in the combination treatment group, the differences were not statistically significant.
Given the priority being given to combination studies of ICIs with a range of different OVs, including other herpes simplex viral agents, it is important to try to understand the reasons for MASTERKEY-265 being a negative trial following on from the positive outcomes from OPTiM. The first issue to consider relates to the distribution of disease stages between the two studies. Patients recruited to MASTERKEY-265 had more advanced disease than in OPTiM (55 vs. 39–45% with stage IVM1b/c disease). As discussed above, previous subgroup analyses have confirmed that T-VEC is more effective in earlier stage disease (IIIB to IVM1a) (17). Thus, it is possible that any beneficial effects of T-VEC were insufficient to translate to PFS and OS improvements in pembrolizumab-treated patients with more advanced disease, in whom the majority of the benefit derived from the systemic therapy that was given in both arms of the study. Review of the Forest plot data between the two arms in MASTERKEY-265 confirms that the T-VEC plus pembrolizumab combination was most effective in earlier stage disease, but the differences are not particularly striking and stage distribution, per se, is unlikely adequately to explain the overall negative result. Perhaps future research could include dedicated studies in patients with early-stage disease.
Another important consideration is the effect of T-VEC on different sized tumours. The OPTiM trial was the first to report that patients with lower tumour burden (lower than 14.5 cm2) have a higher probability of achieving complete response (17). These data are supported by a meta-analysis of 8 studies with 642 patients in stage IIIB–IVM1c melanoma treated with T-VEC. A higher efficacy of T-VEC in early metastatic melanoma was reported in that the CRR and ORR of patients in stage IIIB–IVM1a melanoma were 41% and 64%, respectively, while the CRR and ORR of patients in stage IVM1b–IVM1c were 4% and 9%, respectively (21). Furthermore, apart from the substages of disease, size and number of lesions are also associated with tumour load and considered as baseline characteristics to indicate the chance of clinical responses after treatment with T-VEC. Thus, metastatic lesions with a larger diameter were correlated with poorer responses to T-VEC (22). Similar data exist for the likelihood of patients with melanoma deriving benefit from immunotherapy (23). In keeping with these reports, the Forest plots from MASTERKEY-265 demonstrate an improved degree of benefit for the T-VEC plus pembrolizumab combination for those with a sum of lesion diameters below the median. Again, these data point towards the fact that the T-VEC plus pembrolizumab combination might have yielded a positive trial outcome had the trial been conducted exclusively in patients with lower tumour burdens. Having said that, one must recognise that that is not the area of greatest unmet need in patients with melanoma.
There is another important factor to consider when assessing the outcomes of MASTERKEY-265, and that relates to treatment schedule. It is important to note the difference in the treatment schedule between the initial phase Ib (18) and the full phase III trial (20). As previously described, T-VEC and pembrolizumab combination was administrated concomitantly to the patients from the outset in the phase III trial, while repeat-dose T-VEC was injected 6 weeks prior to the administration of first dose of pembrolizumab in the phase Ib trial. Other data (24) have suggested that the difference in administration schedule could have influenced the activities of the T-VEC/pembrolizumab combination, in that priming the TIME with OVs prior to giving ICIs improved outcomes compared to delivering the treatment concomitantly. One can hypothesise that administration of T-VEC prior to pembrolizumab would allow for reprogramming of the TIME by recruiting and activating APCs with tumour (and viral) antigens with subsequent priming, expansion and migration of tumour-specific CD8+ T cells back to the tumour. Additionally, T-VEC upregulates the expression of PD-L1 and MHC-I on the membrane of tumour cells, and increases the expression of inflammatory cytokines, e.g., IFN-γ and IL-2, which further supports infiltrating CD8+ T cells. Subsequently, as opposed to concomitant, administration of ICIs can further boost and maintain the activation of the existing tumour-specific CD8+ T cells in such an optimised environment (Figure 1).
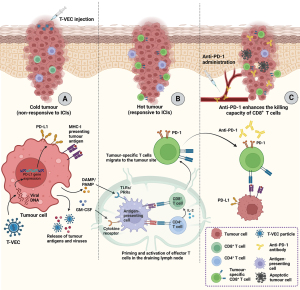
Although the trial conducted by Chesney et al. (20) failed to show significant clinical benefit of T-VEC combined with pembrolizumab, the study did demonstrate that patients with advanced melanoma can safely receive combinations of OV (HSV and, presumably, other virus therapies) with anti-PD-1 ICI. Therefore, it is worth considering the possibility that MASTERKEY-265 was a negative trial because T-VEC is an insufficiently potent therapeutic agent. Ongoing studies of next-generation HSV agents include those involving the RP viral platform (RP-1, 2, and 3). Like T-VEC, RP-1 has deletions of ICP 34.5 and 47 and encodes GM-CSF, but also encodes an additional transgene, constitutively active gibbon ape leukaemia virus fusogenic glycoprotein (GALV), which improves both its cytotoxicity and immune-activating effects. Pre-clinical data show that in a bilateral murine lymphoma tumour model where one tumour was injected with mRP1, and the other left untreated, mRP1 plus anti-PD-1 therapy enhanced anti-tumour effects in both injected and non-injected tumours, compared with either therapy alone (25). RP-2 additionally encodes an expression cassette for production of an anti-CTLA-4 antibody-like molecule within the tumour and RP-3 carries potentially increased potency through its expression of CD40-ligand and 4-1BB ligand-activating constructs. We can expect that a range of other highly engineered and potent viral agents will enter clinical studies and pick up the baton from MASTERKEY-265.
To date, combination treatment regimens of OVs and ICIs have been studied in several clinical trials. Although such regimens have been confirmed as safe and, in small, early-phase studies, have demonstrated positive clinical outcomes in advanced melanoma (and other) patient groups, we have not yet seen data from randomised clinical trials that prove that the combination of OV and ICI can be superior to ICI alone. As we look to the future, the expectation must be that progress will come through greater understanding and exploitation of the biological mechanisms that underpin favourable combinations of OV and ICIs. Most likely, this will involve novel, next-generation engineered OVs that carry multiple payload genes delivered according to biologically optimised dose-schedules alongside single- or multi-agent ICI therapy. Using such approaches, we can be optimistic that we will improve outcomes for patients with advanced melanoma and other cancer types.
Acknowledgments
Funding: The article was supported in part by
Footnote
Provenance and Peer Review: This article was commissioned by the editorial office, Annals of Translational Medicine. The article did not undergo external peer review.
Conflicts of Interest: All authors have completed the ICMJE uniform disclosure form (available at https://atm.amegroups.com/article/view/10.21037/atm-2023-5/coif). VR acknowledges research funding and consulting fees from Replimune to institution. AAM, MP, and KJH reports grants from institution by Boerhringer-Ingelheim and Replimune. KJH reports consulting fees received from Arch Oncology, AstraZeneca, Boehringer-Ingelheim, Codiak Biosciences, F-start Therapeutics, Merck-Serono, MSD, Oncolys BioPharma, Pfizer, and Replimune, and payments or honoraria from Merck-Serono, MSD, and Replimune. AW has no conflicts of interest to declare.
Ethical Statement: The authors are accountable for all aspects of the work in ensuring that questions related to the accuracy or integrity of any part of the work are appropriately investigated and resolved.
Open Access Statement: This is an Open Access article distributed in accordance with the Creative Commons Attribution-NonCommercial-NoDerivs 4.0 International License (CC BY-NC-ND 4.0), which permits the non-commercial replication and distribution of the article with the strict proviso that no changes or edits are made and the original work is properly cited (including links to both the formal publication through the relevant DOI and the license). See: https://creativecommons.org/licenses/by-nc-nd/4.0/.
References
- Jenkins RW, Fisher DE. Treatment of Advanced Melanoma in 2020 and Beyond. J Invest Dermatol 2021;141:23-31. [Crossref] [PubMed]
- Li J, Kan H, Zhao L, et al. Immune checkpoint inhibitors in advanced or metastatic mucosal melanoma: a systematic review. Ther Adv Med Oncol 2020;12:1758835920922028. [Crossref] [PubMed]
- Robert C, Schachter J, Long GV, et al. Pembrolizumab versus Ipilimumab in Advanced Melanoma. N Engl J Med 2015;372:2521-32. [Crossref] [PubMed]
- Larkin J, Chiarion-Sileni V, Gonzalez R, et al. Five-Year Survival with Combined Nivolumab and Ipilimumab in Advanced Melanoma. N Engl J Med 2019;381:1535-46. [Crossref] [PubMed]
- Wolchok JD, Chiarion-Sileni V, Gonzalez R, et al. Long-Term Outcomes With Nivolumab Plus Ipilimumab or Nivolumab Alone Versus Ipilimumab in Patients With Advanced Melanoma. J Clin Oncol 2022;40:127-37. [Crossref] [PubMed]
- Sharma P, Allison JP. Immune checkpoint targeting in cancer therapy: toward combination strategies with curative potential. Cell 2015;161:205-14. [Crossref] [PubMed]
- Davis LE, Shalin SC, Tackett AJ. Current state of melanoma diagnosis and treatment. Cancer Biol Ther 2019;20:1366-79. [Crossref] [PubMed]
- Melcher A, Harrington K, Vile R. Oncolytic virotherapy as immunotherapy. Science 2021;374:1325-6. [Crossref] [PubMed]
- Macedo N, Miller DM, Haq R, et al. Clinical landscape of oncolytic virus research in 2020. J Immunother Cancer 2020;8:e001486. [Crossref] [PubMed]
- Harrington K, Freeman DJ, Kelly B, et al. Optimizing oncolytic virotherapy in cancer treatment. Nat Rev Drug Discov 2019;18:689-706. [Crossref] [PubMed]
- Shi G, Yang Q, Zhang Y, et al. Modulating the Tumor Microenvironment via Oncolytic Viruses and CSF-1R Inhibition Synergistically Enhances Anti-PD-1 Immunotherapy. Mol Ther 2019;27:244-60. [Crossref] [PubMed]
- Kontermann RE, Ungerechts G, Nettelbeck DM. Viro-antibody therapy: engineering oncolytic viruses for genetic delivery of diverse antibody-based biotherapeutics. MAbs 2021;13:1982447. [Crossref] [PubMed]
- Kaufman HL, Ruby CE, Hughes T, et al. Current status of granulocyte-macrophage colony-stimulating factor in the immunotherapy of melanoma. J Immunother Cancer 2014;2:11. [Crossref] [PubMed]
- Liu BL, Robinson M, Han ZQ, et al. ICP34.5 deleted herpes simplex virus with enhanced oncolytic, immune stimulating, and anti-tumour properties. Gene Ther 2003;10:292-303. [Crossref] [PubMed]
- Früh K, Ahn K, Djaballah H, et al. A viral inhibitor of peptide transporters for antigen presentation. Nature 1995;375:415-8. [Crossref] [PubMed]
- Andtbacka RH, Kaufman HL, Collichio F, et al. Talimogene Laherparepvec Improves Durable Response Rate in Patients With Advanced Melanoma. J Clin Oncol 2015;33:2780-8. [Crossref] [PubMed]
- Andtbacka RHI, Collichio F, Harrington KJ, et al. Final analyses of OPTiM: a randomized phase III trial of talimogene laherparepvec versus granulocyte-macrophage colony-stimulating factor in unresectable stage III-IV melanoma. J Immunother Cancer 2019;7:145. [Crossref] [PubMed]
- Ribas A, Dummer R, Puzanov I, et al. Oncolytic Virotherapy Promotes Intratumoral T Cell Infiltration and Improves Anti-PD-1 Immunotherapy. Cell 2017;170:1109-1119.e10. [Crossref] [PubMed]
- Ramelyte E, Tastanova A, Balázs Z, et al. Oncolytic virotherapy-mediated anti-tumor response: a single-cell perspective. Cancer Cell 2021;39:394-406.e4. [Crossref] [PubMed]
- Chesney JA, Ribas A, Long GV, et al. Randomized, Double-Blind, Placebo-Controlled, Global Phase III Trial of Talimogene Laherparepvec Combined With Pembrolizumab for Advanced Melanoma. J Clin Oncol 2023;41:528-40. [Crossref] [PubMed]
- Stahlie EHA, Mulder EEAP, Reijers S, et al. Single agent Talimogene Laherparepvec for stage IIIB-IVM1c melanoma patients: A systematic review and meta-analysis. Crit Rev Oncol Hematol 2022;175:103705. [Crossref] [PubMed]
- Stahlie EHA, Franke V, Zuur CL, et al. T-VEC for stage IIIB-IVM1a melanoma achieves high rates of complete and durable responses and is associated with tumor load: a clinical prediction model. Cancer Immunol Immunother 2021;70:2291-300. [Crossref] [PubMed]
- Katsurada M, Nagano T, Tachihara M, et al. Baseline Tumor Size as a Predictive and Prognostic Factor of Immune Checkpoint Inhibitor Therapy for Non-small Cell Lung Cancer. Anticancer Res 2019;39:815-25. [Crossref] [PubMed]
- Cervera-Carrascon V, Siurala M, Santos JM, et al. TNFa and IL-2 armed adenoviruses enable complete responses by anti-PD-1 checkpoint blockade. Oncoimmunology 2018;7:e1412902. [Crossref] [PubMed]
- Thomas S, Kuncheria L, Roulstone V, et al. Development of a new fusion-enhanced oncolytic immunotherapy platform based on herpes simplex virus type 1. J Immunother Cancer 2019;7:214. [Crossref] [PubMed]