A new era of gene and cell therapy for cancer: a narrative review
Introduction
Drugs can be generally classified into two categories: chemical small molecule drugs and biological macromolecular drugs. With the development of science and technology, cell drugs have shifted from research and development to application. In addition, artificial organ cloning is also expected to be possible in the future. As drugs became increasingly complex in structure and diverse in function, their development has also become more challenging.
In 2017, the use of genetically modified T cells, known as chimeric antigen receptor (CAR) T-cell immunotherapy, was approved by the US Food and Drug Administration (FDA), revolutionizing tumor therapy (1). CAR-T cell therapy, which includes a combination of gene and cell technology, has marked the beginning of the “new era of gene and cell therapy” (1,2). Thus far, CAR-T cell therapy has shown good application prospects in the treatment of various hematologic malignancies, such as acute lymphoblastic leukemia (ALL) (3), lymphoma (4), acute myeloid leukemia (5), and multiple myeloma (6). However, CAR-T cell therapy has been found to be less effective in the treatment of solid tumors due to the complexity of the microenvironment, heterogeneity of solid tumor antigens, diversity of immune evasion mechanisms, and difficulties in migration and permeation to solid tumors (7,8). On the other hand, genetically engineered mesenchymal stem cells (EMSCs) have shown a promising effect when treating solid malignant tumors (9-11).
In the historical development of drugs, if the development of chemical drugs can be called the 1.0 era and the development of biological drugs is called the 2.0 era, then the development of gene and cell therapy drugs can be considered the 3.0 era, while tissue and organ cloning will be considered the 4.0 era (Figure 1). This article reviews the development of gene and cell therapy and the advent of a new era of therapy. We present this article in accordance with the Narrative Review reporting checklist (available at https://atm.amegroups.com/article/view/10.21037/atm-22-3882/rc).
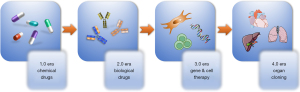
Methods
A literature search of PubMed, SpringerLink, Wiley, Web of Science, and Wanfang database was conducted on articles on gene and cell therapy published until August 2022. The key search words and terms were as follows: “gene therapy”, “cell therapy”, “chimeric antigen receptor T (CART) cell immunotherapy”, AND “tumor” OR “cancer”, “human umbilical cord-derived mesenchymal stem cells”, “engineered mesenchymal stem cells”, “mesenchymal stem cells” AND “trial” AND “tumor” OR “cancer” (Table 1).
Table 1
Items | Specification |
---|---|
Date of search | From March 10, 2022, to August 5, 2022 |
Databases and other sources searched | English databases (PubMed, SpringerLink, Wiley and Web of Science) and Chinese database (Wanfang database) |
Search terms used | “Gene therapy”, “cell therapy”, “chimeric antigen receptor T (CART) cell immunotherapy” AND “tumor” OR “cancer”, “human umbilical cord-derived mesenchymal stem cells”, “engineered mesenchymal stem cells”, “mesenchymal stem cells” AND “trial” AND “tumor” OR “cancer” |
Timeframe | March 3, 1972–August 5, 2022 |
Inclusion and exclusion criteria | The inclusion criteria were as follows: (I) articles written in English and Chinese; (II) articles about gene therapy and cell therapy; (III) articles related to CAR-T cell immunotherapy; (IV) articles about mesenchymal stem cells and engineered mesenchymal stem cells; and (V) articles that involved mesenchymal stem cells and clinical trials |
The exclusion criteria were as follows: (I) articles written languages other than Chinese and English; and (II) meeting abstracts, conference summaries, case reports, and letters | |
Selection process | Articles were independently evaluated by 3 reviewers using the same standard as above |
Discussion
The 21st century is the era of gene and cell therapy
The rise of gene therapy
According to the FDA, gene therapy is defined as products “that mediate their effects by transcription and/or translation of transferred genetic material and/or by integrating into the host genome and that are administered as nucleic acids, viruses, or genetically engineered microorganisms” (12). Gene therapy can be divided into in vivo and ex vivo therapy based on whether the engineering and modification of cells for therapeutic use are performed in vivo or ex vivo before being administered to the recipient (12).
Using gene therapy to treat human genetic diseases was proposed more than 50 years ago (13). Although the road from theory to clinical application has been long and challenging, gene therapy has provided new choices in therapy for multiple diseases. Successful gene therapies have been developed from the administration of viral vectors in vivo directly to the adoptive transfer of genetically engineered cells and genome editing (14). The first human gene therapy experiment was performed in 1970 by Stanfield Rogers, who tried to treat 2 children with hyperargininemia by injecting arginase-containing papillomavirus but failed to achieve a successful outcome (15). In 1999, Jesse Gelsinger volunteered for a gene therapy trial conducted at the University of Pennsylvania in Philadelphia for the rare genetic disease ornithine transcarbamylase deficiency, but he died shortly after the trial (16). The first successful clinical results for a gene therapy trial were published in 2000 and involved severe combined immunodeficiency (SCID)-X1, paving the way for the gene therapy for other diseases (17,18). Over the past decade, messenger RNA (mRNA)-based therapeutics have emerged as highly attractive novel treatments, especially for cancers, with tremendous progress being made in technologies regarding lipid nanoparticles (LNPs) within the design of nonviral vectors for gene delivery (19,20). In 2012, Glybera was approved as the first-ever gene therapy drug for treating a rare disease of lipoprotein lipase deficiency caused by a congenital gene defect (21). The success of Glybera indicated that human genetic diseases caused by congenital gene defects could be repaired with drugs. In the same year, American scientist Jennifer A. Doudna and French scientist Emmanuelle Charpentier developed the clustered regularly interspaced short palindromic repeats (CRISPR)/CRISPR-associated protein 9 (CAS9) gene-editing technology that revolutionized the field of gene therapy (22). In 2017, the FDA approved the listing of CAR-T cells (1), which was of epoch-making significance in the fight against cancer. The approval further increased the enthusiasm for capital investment in the gene therapy industry and advanced the development of the field. Remarkably, two LNP-based mRNA vaccines against severe acute respiratory syndrome coronavirus 2 (SARS-CoV-2) were granted the first historic emergency use authorization from the FDA in 2020, which was a milestone for the use of mRNA-based gene therapy and attracted extensive attention worldwide (23,24).
The advent of the era of cell therapy
The 3.0 era of gene and cell therapy drugs began with the development of gene and cell biology technologies. From the perspective of life development, diseases in a living body usually indicate problems in basic unit of life—cells. Therefore, gene or cell therapy aims to repair damaged cells or tissues rather than the disease itself. For example, to treat infectious diseases, it is necessary to eliminate pathogenic microorganisms and repair and regenerate tissues and organs damaged by disease. For the management of cancer, cancer immunotherapy is the latest treatment method (25). Although an excessive immune response kills cancer cells, it also affects the surrounding healthy tissues and organs. Thus, finding a therapy that can mediate immune responses and repair and regenerate damaged tissues is essential. Accordingly, cell therapy has become a novel strategy for treating such diseases (26).
Currently, the main focus of research and development of cell drugs includes immune and stem cells. Immune cells refer to cells that participate or assist in the process of an organism’s immune response. Lymphocytes have a key role in the immune process. During the immune response, various lymphocyte groups not only restrict each other but also cooperate to collectively exert immune function. CAR-T cells, a new type of therapeutic T cell (1,27), are exemplary immune cells used for cell therapy; they express chimeric receptors that can recognize specific tumor antigens on the surface of T cells through genetic engineering (28). The expanded CAR-T cells can be reinfused into the patients to kill tumor cells (29). Stem cells, which can self-proliferate and differentiate, are another popular product for cell therapy. A large amount of quiescent stem cells are stored in tissues and organs, forming the basis for organ development and regeneration (30). The clinical application of stem cells has spawned the development of regenerative medicine, bringing hope for treating various diseases (31). One of the most representative stem cell types is mesenchymal stem cells (MSCs), a heterogeneous subset of stromal stem cells that exists in many fetal and adult tissues and can differentiate into cells of the mesodermal and other embryonic lineages (32,33). MSCs are a type of multipotent stem cell with the potential for self-renewal and multidirectional differentiation (34) as well the exertion of multidirectional anti-inflammatory (35), immunomodulation (36), tissue repair, and targeted chemotaxis functions (37), representing a wide range of clinical applications (26).
The current status of the development of stem cell drugs in China
In recent years, the Chinese government has actively promoted and regulated the development of the stem cell industry. At the time of writing, stem cell products are still in the developmental stage in China. As of June 2022, stem cell research and development enterprises have submitted 44 investigational new drug (INDs) applications for MSC-related drugs to the National Center for Drug Evaluation (CDE) in China, 34 of which have been approved thus far (Table 2). The MSC products approved for registered clinical trials constitute China’s first echelon of stem cell drug development. MSC products that have entered the phase II clinical trial stage represent the vanguard of China’s emerging stem cell drug industry and are expected to achieve future breakthroughs in new stem cell drug innovation in China.
Table 2
Auto-/allo- | Cell type | Source of MSCs |
---|---|---|
Auto- [3] | Autotransfusion of cells [2] | Bronchial basal layer cells [2] |
Mesenchymal progenitor cells [1] | Adipose tissue [1] | |
Allo- [31] | Mesenchymal stem cells [25] | Umbilical cord [18] |
Bone marrow [3] | ||
Adipose tissue [1] | ||
Dental pulp [1] | ||
Placenta [1] | ||
Uterine blood [1] | ||
Precursor cells [3] | Human embryogenic mesenchymal precursor cells [3] | |
Progenitor cells [2] | Adipose mesenchymal progenitor cells [1] | |
Vascular endothelial progenitor cells [1] | ||
Human embryogenic cells [1] | The ectoderm of the blastocyst stage on day 8–9 of embryonic development [1] |
The numbers in square brackets represent the number of clinical trials for MSCs approved by the CDE in China. CDE, Center for Drug Evaluation; MSC, mesenchymal stem cell; auto-, autologous; allo-, allogeneic.
MSCs were originally isolated from adult bone marrow, and their other main sources include the umbilical cord, adipose tissue, muscle, and placenta (38). There are records of the umbilical cord being used as medicine in China since ancient times, which can be traced to the Tang dynasty. Recent studies have confirmed that umbilical cord tissue is rich in MSCs (39). The biological properties and functions of umbilical cord-derived MSCs (UC-MSCs) are similar to those of bone marrow-derived MSCs (BM-MSCs) and were found to have good effects in preclinical and clinical studies in the treatment of various diseases (40). The research and development of UC-MSCs in China began early; therefore, the processes of industrialization are relatively complete.
Moreover, many clinical studies related to the human UC-MSCs (hUC-MSCs) are underway (41-44). Compared with MSCs from other sources, hUC-MSCs have stronger immune regulation and regeneration function and are currently recognized as a stem cell type of “clinical utility”. They have many major advantages (45). First, the umbilical cord is their most abundant source (46). They can be easily collected with a low risk of contamination of cells and pathogens from the maternal source (47,48). Additionally, hUC-MSCs are suitable for large-scale commercial production at a lower cost. Second, since the umbilical cord is regarded as medical waste, its use is associated with fewer ethical and moral restrictions (46). Third, hUC-MSCs have stable cells and strong biological functions. They are more primitive than BM-MSCs and have a stronger ability to proliferate and differentiate (49). Fourth, due to low immunogenicity (50), they can be used for allotransplantation. To summarize, hUC-MSCs have the characteristics of drugs, including “industrialization, scalization, and standardization” (51), and currently represent the most promising MSC drug.
hUC-MSC-related clinical trials account for more than 50% (18/34) of the MSC-related clinical trials in China and have a wide range of applications, including knee osteoarthritis, acute-on-chronic liver failure, rheumatoid arthritis, inflammatory bowel disease, graft-versus-host disease, chronic periodontitis, ischemic stroke, diabetic foot, idiopathic pulmonary fibrosis, and chronic plaque psoriasis (Figure 2).
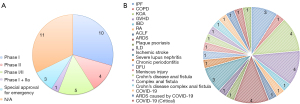
In the United States, the development of drugs based on MSCs mainly derives from bone marrow and adipose sources, and there is less drug development for hUC-MSCs. China will likely be the first to approve hUC-MSCs as drugs for clinical use. In addition, the application of genetic EMSCs against cancer has also received extensive attention.
The 3.0 era of gene and cell drugs in cancer therapy
The development of CAR-T cells
The successful development of CAR-T cells heralds the arrival of the era of gene and cell drugs and tumor cures (52,53). CAR-T cell therapy has produced noteworthy results in treating certain hematologic malignancies (54). The first patients with relapsed or refractory B-cell leukemia treated with CD19-targeted CAR-T cells showed favorable responses (3,55,56). Thus, CAR-T cell therapy is a cause for optimism in some patients with relapsed and refractory hematologic malignancies. CAR-T cell therapy was approved by the FDA in 2017 for the treatment of ALL (56-59) and to treat diffuse large B-cell lymphoma in the United States (56,60). FDA approvals were also obtained for the use of CAR-T cell therapy to treat mantle cell lymphoma in 2020 (61) and multiple myeloma in 2021 (62,63). However, most patients with B-cell malignancies were found to relapse within 1 to 2 years after CAR-T cell treatment (56). For example, even though the initial complete response rates of patients with ALL were greater than 80% after treatment with CAR-T19 cell therapy, nearly 50% of patients were found to relapse within the first year (56,59,64). The common mechanisms associated with relapse after CAR-T cell therapy include loss of the tumor antigen, T-cell exhaustion, and immunosuppression of the tumor microenvironment (TME) (52). Moreover, many obstacles prevent the use of CAR-T cell immunotherapy in the treatment of solid tumors (7).
The microenvironment of solid tumors is a decisive factor in the proliferation, metastasis, chemotherapy resistance, and immune escape of tumor cells (65,66). There are a large number of matrix and immunosuppressive components that have important roles in this process (67). A physical barrier prevents the infiltration of CAR-T cells (68). Immunosuppressive cells, such as myeloid-derived suppressor cells, regulatory T cells (Tregs), and tumor-associated macrophages (68,69) as well as their secreted inhibitory factors, such as interleukin (IL)-10 and IL-4, inhibit the connection, dampening the effect of CAR-T cells on tumor cells through a complex signaling network (70). Furthermore, solid tumors are often accompanied by aberrant vasculature and dense fibrogenic extracellular matrix, forming a hypoxic, acidic microenvironment that lowers the survival and activation of infiltrating T cells (71-73). Due to the heterogeneity between various malignant tumor tissues and patients, CAR-T cell therapy is only effective on one part of tumor cells and can sometimes increase the risk of tumor recurrence and metastasis (74,75). Therefore, research for more effective gene and cell drugs for cancer therapy, particularly for solid tumors, is urgently required, and MSCs may be an ideal choice.
The development of EMSCs
Treating tumors with naïve MSCs is a double-edged sword (76). Naïve MSCs are recruited to tumor sites by tumor cells where they can inhibit or promote tumor proliferation and development, depending on the different cytokine profiles released. Thus, MSCs can both suppress and promote cancer cells (10,77,78). Cancer cells can trigger an inflammatory response within the tumor, thereby establishing a tumor-promoting microenvironment (79).
Inflammation is a common feature of the TME, and infiltration of immune cells into tumors has a dual role: it can lead to antitumor responses or suppress immune responses to promote malignant progression (79-81). Furthermore, given the repeated alternation of tissue damage and regeneration due to the constant inflammation in tumors (65), tumors are considered “wounds that do not heal” (82).
Clinically, about 90% of patients with cancer die of tumor metastasis. Thus, the prevention of metastasis is essential for prolonging the survival of patients (83). Preventing tumor occurrence and metastasis is the most economical and effective strategy. Inflammation has an important role in the different stages of tumor development, including the initiation stage, development stage, tumor transformation stage, and infiltration and metastasis stage of tumorigenesis (84). Additionally, inflammation affects immune surveillance and response to tumor therapy (85). The anti-inflammatory effect of MSCs suggests their potential to prevent tumorigenesis and metastasis (86,87). hUC-MSCs have been reported to inhibit tumorigenesis by reducing the proportion of macrophages in mice with colon cancer (88). MSCs injected intravenously can be detected in the tumor tissue, revealing inhibited lung metastasis formation in mice, and the possible mechanism may be direct interaction between MSCs and cancer cells through soluble factors (89). However, for patients with some advanced tumors, naïve MSCs cannot achieve meaningful therapeutic effect, and the immunosuppression effects of MSCs may even have a role in promoting tumor development (86).
Since MSCs can enter the sites of tumors and metastasis, they can be used as “Trojan horses of cells” to transport antitumor factors into the TME at sites of tumors and metastasis (Figure 3) (90). Furthermore, MSCs can be genetically modified, and components expressed by EMSCs can exert effects of antiproliferation, proapoptosis, and antiangiogenesis (90,91). Besides these effects, MSCs can be modified to express the cytokines (i.e., tumor necrosis factor superfamily, interferons, and, interleukins) that can increase endogenous tumor immunity and effectively inhibit tumor growth, development, and metastasis (92-94). In addition, by coating or via genetic engineering antibodies, MSCs can stably and constantly express antibodies against tumor-specific ligands to tumor loci, which can inhibit tumor growth and kill tumor cells (95,96). Moreover, MSCs can also be engineered to carry suicide genes to produce toxic substances that specifically inhibit tumor growth while sparing normal tissue surrounding the tumor (97). Furthermore, MSCs can deliver therapeutic antitumor microRNA (miRNA) to inhibit tumor angiogenesis, growth, migration, and chemotherapy resistance (98-100). Additionally, MSCs can be used as delivery vehicles for conventional chemotherapy drugs with antitumor effects (101) and oncolytic viruses that can selectively kill cancer cells (102). More importantly, MSCs can be converted into potent antigen-presenting cells (APCs) by either genetically engineering them to express the immunoproteasome complex (103,104) or by pharmacological means using UM171A (105) or tranylcypromine, which can inhibit the lysine-specific demethylase 1 (LSD1) in MSCs (106). These factors promote APC-like properties that may be conducive to designing universal MSC-based anticancer vaccines (103).
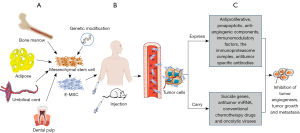
Moreover, MSCs can promote tissue and organ repair (107), improve the local microenvironment of the tumor (108), and prevent tumor recurrence (109), which can overcome some obstacles of CAR-T cell therapy in the treatment of solid tumors. Consequently, EMSCs have great potential for clinical applications in treating cancer.
Clinical studies of EMSCs
Thus far, few clinical trials on EMSCs modified by viruses or nonviruses have been conducted for different diseases. The first clinical study on the use of EMSCs in treating solid tumors, reported in 2010, used the systemic oncolytic virus provided by autologous bone marrow MSCs to treat metastatic neuroblastoma. One-quarter of patients achieved a complete response more than 4 years after diagnosis (36 months after the first treatment), which was the first time a patient with stage IV neuroblastoma achieved a complete response in the investigator’s 20 years of experience with neuroblastoma (110). In 2019, another clinical trial, the phase I–II treatment of advanced tumors with mesenchymal cells 1 (TREAT-ME-1) trial, which was focused on herpes simplex virus thymidine kinase (HSV-TK)-modified MSCs against advanced gastrointestinal tumors with suicide gene therapy system, evaluated the safety and tolerability of transgenic autologous MSCs combined with ganciclovir against advanced gastrointestinal adenocarcinoma and showed preliminary efficacy of the therapy in clinical stabilization of the disease (111). In the latest clinical trial, published in 2020, autologous MSCs carrying the oncolytic virus Icovir-5 called Celyvir, an advanced therapy medicine for patients with advanced tumors, were used to treat malignant diseases. The study found no grade 2 to 5 toxicities, which suggested the combination of MSCs and oncolytic adenovirus was safe. Furthermore, the disease was stabilized in 2 patients with neuroblastoma (112). Although further phases of clinical trials are needed, EMSCs provide a valuable option for some incurable diseases in clinical practice.
Conclusions
Gene and cell therapies have broad application prospects in treating human diseases, especially autoimmune diseases and malignant tumors. These may further promote the development of precision medicine and bring changes to traditional medicine. According to patients’ genes, environment, and lifestyle, an individualized treatment plan can be systematically formulated by considering the optimal treatment effect and the optimal medical cost-benefit ratio. Among the different cell types, MSCs are emerging as a potential and valuable cellular drug that can be successfully applied in cancer, autoimmune diseases and various tissue or organ abnormalities (e.g., neurodegenerative diseases, cardiovascular diseases and bone tissue fractures) in the future (9,76,113-117). Although further clinical practice is required, we believe that naïve MSCs and EMSCs will eventually open a new era of human disease prevention and treatment in the near future.
Acknowledgments
We would like to thank Ivana Stojcic (Shanghai Meisi Medical Technology Co., Ltd.) for editing the language of this paper.
Funding: This work was supported by grants from Beijing Municipal Science and Technology Project (No. Z221100007922028).
Footnote
Reporting Checklist: The authors have completed the Narrative Review reporting checklist. Available at https://atm.amegroups.com/article/view/10.21037/atm-22-3882/rc
Conflicts of Interest: All authors have completed the ICMJE uniform disclosure form (available at https://atm.amegroups.com/article/view/10.21037/atm-22-3882/coif). The authors have no conflicts of interest to declare.
Ethical Statement: The authors are accountable for all aspects of the work in ensuring that questions related to the accuracy or integrity of any part of the work are appropriately investigated and resolved.
Open Access Statement: This is an Open Access article distributed in accordance with the Creative Commons Attribution-NonCommercial-NoDerivs 4.0 International License (CC BY-NC-ND 4.0), which permits the non-commercial replication and distribution of the article with the strict proviso that no changes or edits are made and the original work is properly cited (including links to both the formal publication through the relevant DOI and the license). See: https://creativecommons.org/licenses/by-nc-nd/4.0/.
References
- June CH, O'Connor RS, Kawalekar OU, et al. CAR T cell immunotherapy for human cancer. Science 2018;359:1361-5. [Crossref] [PubMed]
- Atilla E, Kilic P, Gurman G. Cellular therapies: Day by day, all the way. Transfus Apher Sci 2018;57:187-96. [Crossref] [PubMed]
- Brentjens RJ, Rivière I, Park JH, et al. Safety and persistence of adoptively transferred autologous CD19-targeted T cells in patients with relapsed or chemotherapy refractory B-cell leukemias. Blood. 2011;118:4817-28. [Crossref] [PubMed]
- Brudno JN, Lam N, Vanasse D, et al. Safety and feasibility of anti-CD19 CAR T cells with fully human binding domains in patients with B-cell lymphoma. Nat Med 2020;26:270-80. [Crossref] [PubMed]
- Marvin-Peek J, Savani BN, Olalekan OO, et al. Challenges and Advances in Chimeric Antigen Receptor Therapy for Acute Myeloid Leukemia. Cancers (Basel) 2022;14:497. [Crossref] [PubMed]
- Schmidts A, Ormhøj M, Choi BD, et al. Rational design of a trimeric APRIL-based CAR-binding domain enables efficient targeting of multiple myeloma. Blood Adv 2019;3:3248-60. [Crossref] [PubMed]
- Zhang BL, Qin DY, Mo ZM, et al. Hurdles of CAR-T cell-based cancer immunotherapy directed against solid tumors. Sci China Life Sci 2016;59:340-8. [Crossref] [PubMed]
- Tahmasebi S, Elahi R, Esmaeilzadeh A. Solid Tumors Challenges and New Insights of CAR T Cell Engineering. Stem Cell Rev Rep 2019;15:619-36. [Crossref] [PubMed]
- Shah K. Mesenchymal stem cells engineered for cancer therapy. Adv Drug Deliv Rev 2012;64:739-48. [Crossref] [PubMed]
- Feng B, Chen L. Review of mesenchymal stem cells and tumors: executioner or coconspirator? Cancer Biother Radiopharm 2009;24:717-21. [Crossref] [PubMed]
- Nakamizo A, Marini F, Amano T, et al. Human bone marrow-derived mesenchymal stem cells in the treatment of gliomas. Cancer Res 2005;65:3307-18. [Crossref] [PubMed]
- Wirth T, Parker N, Ylä-Herttuala S. History of gene therapy. Gene 2013;525:162-9. [Crossref] [PubMed]
- Friedmann T, Roblin R. Gene therapy for human genetic disease? Science 1972;175:949-55. [Crossref] [PubMed]
- Dunbar CE, High KA, Joung JK, et al. Gene therapy comes of age. Science 2018;359:eaan4672. [Crossref] [PubMed]
- Terheggen HG, Lowenthal A, Lavinha F, et al. Unsuccessful trial of gene replacement in arginase deficiency. Z Kinderheilkd 1975;119:1-3. [PubMed]
- Smith L, Byers JF. Gene therapy in the post-Gelsinger era. JONAS Healthc Law Ethics Regul 2002;4:104-10. [Crossref] [PubMed]
- Cavazzana-Calvo M, Hacein-Bey S, de Saint Basile G, et al. Gene therapy of human severe combined immunodeficiency (SCID)-X1 disease. Science 2000;288:669-72. [Crossref] [PubMed]
- Anderson WF. Gene therapy. The best of times, the worst of times. Science 2000;288:627-9. [Crossref] [PubMed]
- Guevara ML, Persano F, Persano S. Advances in Lipid Nanoparticles for mRNA-Based Cancer Immunotherapy. Front Chem 2020;8:589959. [Crossref] [PubMed]
- Guan S, Rosenecker J. Nanotechnologies in delivery of mRNA therapeutics using nonviral vector-based delivery systems. Gene Ther 2017;24:133-43. [Crossref] [PubMed]
- Ylä-Herttuala S. Endgame: glybera finally recommended for approval as the first gene therapy drug in the European union. Mol Ther 2012;20:1831-2. [Crossref] [PubMed]
- Jinek M, Chylinski K, Fonfara I, et al. A programmable dual-RNA-guided DNA endonuclease in adaptive bacterial immunity. Science 2012;337:816-21. [Crossref] [PubMed]
- Qiu M, Li Y, Bloomer H, et al. Developing Biodegradable Lipid Nanoparticles for Intracellular mRNA Delivery and Genome Editing. Acc Chem Res 2021;54:4001-11. [Crossref] [PubMed]
- Verbeke R, Lentacker I, De Smedt SC, et al. The dawn of mRNA vaccines: The COVID-19 case. J Control Release 2021;333:511-20. [Crossref] [PubMed]
- Yang Y. Cancer immunotherapy: harnessing the immune system to battle cancer. J Clin Invest 2015;125:3335-7. [Crossref] [PubMed]
- Rodríguez-Fuentes DE, Fernández-Garza LE, Samia-Meza JA, et al. Mesenchymal Stem Cells Current Clinical Applications: A Systematic Review. Arch Med Res 2021;52:93-101. [Crossref] [PubMed]
- Sadelain M, Rivière I, Brentjens R. Targeting tumours with genetically enhanced T lymphocytes. Nat Rev Cancer 2003;3:35-45. [Crossref] [PubMed]
- Sadelain M, Brentjens R, Rivière I. The basic principles of chimeric antigen receptor design. Cancer Discov 2013;3:388-98. [Crossref] [PubMed]
- Sadelain M, Rivière I, Riddell S. Therapeutic T cell engineering. Nature 2017;545:423-31. [Crossref] [PubMed]
- Zakrzewski W, Dobrzyński M, Szymonowicz M, et al. Stem cells: past, present, and future. Stem Cell Res Ther 2019;10:68. [Crossref] [PubMed]
- Jin J. Stem Cell Treatments. JAMA 2017;317:330. [Crossref] [PubMed]
- Liu G, Wang L, Pang T, et al. Umbilical cord-derived mesenchymal stem cells regulate thymic epithelial cell development and function in Foxn1(-/-) mice. Cell Mol Immunol 2014;11:275-84. [Crossref] [PubMed]
- Uccelli A, Moretta L, Pistoia V. Mesenchymal stem cells in health and disease. Nat Rev Immunol 2008;8:726-36. [Crossref] [PubMed]
- Caplan AI. Adult mesenchymal stem cells for tissue engineering versus regenerative medicine. J Cell Physiol 2007;213:341-7. [Crossref] [PubMed]
- Gugjoo MB, Hussain S. Mesenchymal Stem Cell-Mediated Immuno-Modulatory and Anti- Inflammatory Mechanisms in Immune and Allergic Disorders. Recent Pat Inflamm Allergy Drug Discov 2020;14:3-14. [Crossref] [PubMed]
- Song N, Scholtemeijer M, Shah K. Mesenchymal Stem Cell Immunomodulation: Mechanisms and Therapeutic Potential. Trends Pharmacol Sci 2020;41:653-64. [Crossref] [PubMed]
- Fu X, Liu G, Halim A, et al. Mesenchymal Stem Cell Migration and Tissue Repair. Cells 2019;8:784. [Crossref] [PubMed]
- Hmadcha A, Martin-Montalvo A, Gauthier BR, et al. Therapeutic Potential of Mesenchymal Stem Cells for Cancer Therapy. Front Bioeng Biotechnol 2020;8:43. [Crossref] [PubMed]
- Alatyyat SM, Alasmari HM, Aleid OA, et al. Umbilical cord stem cells: Background, processing and applications. Tissue Cell 2020;65:101351. [Crossref] [PubMed]
- Ding DC, Chang YH, Shyu WC, et al. Human umbilical cord mesenchymal stem cells: a new era for stem cell therapy. Cell Transplant 2015;24:339-47. [Crossref] [PubMed]
- Meng F, Xu R, Wang S, et al. Human umbilical cord-derived mesenchymal stem cell therapy in patients with COVID-19: a phase 1 clinical trial. Signal Transduct Target Ther 2020;5:172. [Crossref] [PubMed]
- Cao Y, Sun H, Zhu H, et al. Allogeneic cell therapy using umbilical cord MSCs on collagen scaffolds for patients with recurrent uterine adhesion: a phase I clinical trial. Stem Cell Res Ther 2018;9:192. [Crossref] [PubMed]
- Shi M, Li YY, Xu RN, et al. Mesenchymal stem cell therapy in decompensated liver cirrhosis: a long-term follow-up analysis of the randomized controlled clinical trial. Hepatol Int 2021;15:1431-41. [Crossref] [PubMed]
- Lanzoni G, Linetsky E, Correa D, et al. Umbilical cord mesenchymal stem cells for COVID-19 acute respiratory distress syndrome: A double-blind, phase 1/2a, randomized controlled trial. Stem Cells Transl Med 2021;10:660-73. [Crossref] [PubMed]
- Shaikh MS, Shahzad Z, Tash EA, et al. Human Umbilical Cord Mesenchymal Stem Cells: Current Literature and Role in Periodontal Regeneration. Cells 2022;11:1168. [Crossref] [PubMed]
- Beeravolu N, McKee C, Alamri A, et al. Isolation and Characterization of Mesenchymal Stromal Cells from Human Umbilical Cord and Fetal Placenta. J Vis Exp 2017;55224. [PubMed]
- Denys M, Léon A, Robert C, et al. Biosafety Evaluation of Equine Umbilical Cord-Derived Mesenchymal Stromal Cells by Systematic Pathogen Screening in Peripheral Maternal Blood and Paired UC-MSCs. Biopreserv Biobank 2020;18:73-81. [Crossref] [PubMed]
- Vackova I, Czernekova V, Tomanek M, et al. Absence of maternal cell contamination in mesenchymal stromal cell cultures derived from equine umbilical cord tissue. Placenta 2014;35:655-7. [Crossref] [PubMed]
- Baksh D, Yao R, Tuan RS. Comparison of proliferative and multilineage differentiation potential of human mesenchymal stem cells derived from umbilical cord and bone marrow. Stem Cells 2007;25:1384-92. [Crossref] [PubMed]
- Chen PM, Yen ML, Liu KJ, et al. Immunomodulatory properties of human adult and fetal multipotent mesenchymal stem cells. J Biomed Sci 2011;18:49. [Crossref] [PubMed]
- Zhang Y, Na T, Zhang K, et al. GMP-grade microcarrier and automated closed industrial scale cell production platform for culture of MSCs. J Tissue Eng Regen Med 2022;16:934-44. [Crossref] [PubMed]
- Larson RC, Maus MV. Recent advances and discoveries in the mechanisms and functions of CAR T cells. Nat Rev Cancer 2021;21:145-61. [Crossref] [PubMed]
- Titov A, Zmievskaya E, Ganeeva I, et al. Adoptive Immunotherapy beyond CAR T-Cells. Cancers (Basel) 2021;13:743. [Crossref] [PubMed]
- Bourbon E, Ghesquières H, Bachy E. CAR-T cells, from principle to clinical applications. Bull Cancer 2021;108:S4-S17. [Crossref] [PubMed]
- Kalos M, Levine BL, Porter DL, et al. T cells with chimeric antigen receptors have potent antitumor effects and can establish memory in patients with advanced leukemia. Sci Transl Med 2011;3:95ra73. [Crossref] [PubMed]
- Sakemura R, Cox MJ, Hefazi M, et al. Resistance to CART cell therapy: lessons learned from the treatment of hematological malignancies. Leuk Lymphoma 2021;62:2052-63. [Crossref] [PubMed]
- Brentjens RJ, Davila ML, Riviere I, et al. CD19-targeted T cells rapidly induce molecular remissions in adults with chemotherapy-refractory acute lymphoblastic leukemia. Sci Transl Med 2013;5:177ra38. [Crossref] [PubMed]
- Mullard A. FDA approves first CAR T therapy. Nat Rev Drug Discov 2017;16:669. [PubMed]
- Maude SL, Laetsch TW, Buechner J, et al. Tisagenlecleucel in Children and Young Adults with B-Cell Lymphoblastic Leukemia. N Engl J Med 2018;378:439-48. [Crossref] [PubMed]
- FDA Approves Second CAR T-cell Therapy. Cancer Discov 2018;8:5-6. [Crossref] [PubMed]
- Voelker R. CAR-T Therapy Is Approved for Mantle Cell Lymphoma. JAMA 2020;324:832. [Crossref] [PubMed]
- Mullard A. FDA approves first BCMA-targeted CAR-T cell therapy. Nat Rev Drug Discov 2021;20:332. [Crossref] [PubMed]
- Mullard A. FDA approves second BCMA-targeted CAR-T cell therapy. Nat Rev Drug Discov 2022;21:249. [Crossref] [PubMed]
- Park JH, Rivière I, Gonen M, et al. Long-Term Follow-up of CD19 CAR Therapy in Acute Lymphoblastic Leukemia. N Engl J Med 2018;378:449-59. [Crossref] [PubMed]
- Hinshaw DC, Shevde LA. The Tumor Microenvironment Innately Modulates Cancer Progression. Cancer Res 2019;79:4557-66. [Crossref] [PubMed]
- Khalaf K, Hana D, Chou JT, et al. Aspects of the Tumor Microenvironment Involved in Immune Resistance and Drug Resistance. Front Immunol 2021;12:656364. [Crossref] [PubMed]
- Stromnes IM, Schmitt TM, Hulbert A, et al. T Cells Engineered against a Native Antigen Can Surmount Immunologic and Physical Barriers to Treat Pancreatic Ductal Adenocarcinoma. Cancer Cell 2015;28:638-52. [Crossref] [PubMed]
- Miao L, Zhang Z, Ren Z, et al. Obstacles and Coping Strategies of CAR-T Cell Immunotherapy in Solid Tumors. Front Immunol 2021;12:687822. [Crossref] [PubMed]
- Quail DF, Joyce JA. Microenvironmental regulation of tumor progression and metastasis. Nat Med 2013;19:1423-37. [Crossref] [PubMed]
- Jin C, Yu D, Essand M. Prospects to improve chimeric antigen receptor T-cell therapy for solid tumors. Immunotherapy 2016;8:1355-61. [Crossref] [PubMed]
- Moon EK, Wang LC, Dolfi DV, et al. Multifactorial T-cell hypofunction that is reversible can limit the efficacy of chimeric antigen receptor-transduced human T cells in solid tumors. Clin Cancer Res 2014;20:4262-73. [Crossref] [PubMed]
- Berahovich R, Liu X, Zhou H, et al. Hypoxia Selectively Impairs CAR-T Cells In Vitro. Cancers (Basel) 2019;11:602. [Crossref] [PubMed]
- Kankeu Fonkoua LA, Sirpilla O, Sakemura R, et al. CAR T cell therapy and the tumor microenvironment: Current challenges and opportunities. Mol Ther Oncolytics 2022;25:69-77. [Crossref] [PubMed]
- Roessler S, Budhu A, Wang XW. Deciphering cancer heterogeneity: the biological space. Front Cell Dev Biol 2014;2:12. [Crossref] [PubMed]
- Zhang H, Ye ZL, Yuan ZG, et al. New Strategies for the Treatment of Solid Tumors with CAR-T Cells. Int J Biol Sci 2016;12:718-29. [Crossref] [PubMed]
- Marofi F, Vahedi G, Biglari A, et al. Mesenchymal Stromal/Stem Cells: A New Era in the Cell-Based Targeted Gene Therapy of Cancer. Front Immunol 2017;8:1770. [Crossref] [PubMed]
- Zhang L, Xiang J, Li G. The uncertain role of unmodified mesenchymal stem cells in tumor progression: what master switch? Stem Cell Res Ther 2013;4:22. [Crossref] [PubMed]
- Spaeth E, Klopp A, Dembinski J, et al. Inflammation and tumor microenvironments: defining the migratory itinerary of mesenchymal stem cells. Gene Ther 2008;15:730-8. [Crossref] [PubMed]
- Singh N, Baby D, Rajguru JP, et al. Inflammation and cancer. Ann Afr Med 2019;18:121-6. [Crossref] [PubMed]
- Karin M. Nuclear factor-kappaB in cancer development and progression. Nature 2006;441:431-6. [Crossref] [PubMed]
- de Visser KE, Coussens LM. The interplay between innate and adaptive immunity regulates cancer development. Cancer Immunol Immunother 2005;54:1143-52. [Crossref] [PubMed]
- Dvorak HF. Tumors: wounds that do not heal. Similarities between tumor stroma generation and wound healing. N Engl J Med 1986;315:1650-9. [Crossref] [PubMed]
- Ganesh K, Massagué J. Targeting metastatic cancer. Nat Med 2021;27:34-44. [Crossref] [PubMed]
- Hibino S, Kawazoe T, Kasahara H, et al. Inflammation-Induced Tumorigenesis and Metastasis. Int J Mol Sci 2021;22:5421. [Crossref] [PubMed]
- Grivennikov SI, Greten FR, Karin M. Immunity, inflammation, and cancer. Cell 2010;140:883-99. [Crossref] [PubMed]
- Liang W, Chen X, Zhang S, et al. Mesenchymal stem cells as a double-edged sword in tumor growth: focusing on MSC-derived cytokines. Cell Mol Biol Lett 2021;26:3. [Crossref] [PubMed]
- Todoric J, Antonucci L, Karin M. Targeting Inflammation in Cancer Prevention and Therapy. Cancer Prev Res (Phila) 2016;9:895-905. [Crossref] [PubMed]
- Fu Y, Li J, Li M, et al. Umbilical Cord Mesenchymal Stem Cells Ameliorate Inflammation-Related Tumorigenesis via Modulating Macrophages. Stem Cells Int 2022;2022:1617229. [Crossref] [PubMed]
- Meleshina AV, Cherkasova EI, Shirmanova MV, et al. Influence of mesenchymal stem cells on metastasis development in mice in vivo. Stem Cell Res Ther 2015;6:15. [Crossref] [PubMed]
- Nowakowski A, Drela K, Rozycka J, et al. Engineered Mesenchymal Stem Cells as an Anti-Cancer Trojan Horse. Stem Cells Dev 2016;25:1513-31. [Crossref] [PubMed]
- Keung EZ, Nelson PJ, Conrad C. Concise review: genetically engineered stem cell therapy targeting angiogenesis and tumor stroma in gastrointestinal malignancy. Stem Cells 2013;31:227-35. [Crossref] [PubMed]
- Quiroz-Reyes AG, González-Villarreal CA, Martínez-Rodriguez H, et al. A combined antitumor strategy of separately transduced mesenchymal stem cells with soluble TRAIL and IFNβ produces a synergistic activity in the reduction of lymphoma and mice survival enlargement. Mol Med Rep 2022;25:206. [Crossref] [PubMed]
- Kułach N, Pilny E, Cichoń T, et al. Mesenchymal stromal cells as carriers of IL-12 reduce primary and metastatic tumors of murine melanoma. Sci Rep 2021;11:18335. [Crossref] [PubMed]
- Chulpanova DS, Gilazieva ZE, Akhmetzyanova ER, et al. Cytochalasin B-induced membrane vesicles from human mesenchymal stem cells overexpressing TRAIL, PTEN and IFN-β1 can kill carcinoma cancer cells. Tissue Cell 2021;73:101664. [Crossref] [PubMed]
- Zhang J, Yuan Z, Zhong W, et al. Stem Cell as Vehicles of Antibody in Treatment of Lymphoma: a Novel and Potential Targeted Therapy. Stem Cell Rev Rep 2021;17:829-41. [Crossref] [PubMed]
- Yan C, Li S, Li Z, et al. Human umbilical cord mesenchymal stem cells as vehicles of CD20-specific TRAIL fusion protein delivery: a double-target therapy against non-Hodgkin's lymphoma. Mol Pharm 2013;10:142-51. [Crossref] [PubMed]
- Amara I, Touati W, Beaune P, et al. Mesenchymal stem cells as cellular vehicles for prodrug gene therapy against tumors. Biochimie 2014;105:4-11. [Crossref] [PubMed]
- Lee JK, Park SR, Jung BK, et al. Exosomes derived from mesenchymal stem cells suppress angiogenesis by down-regulating VEGF expression in breast cancer cells. PLoS One 2013;8:e84256. [Crossref] [PubMed]
- Yu S, Zhou Y, Niu L, et al. Mesenchymal stem cell-derived exosome mir-342-3p inhibits metastasis and chemo-resistance of breast cancer through regulating ID4. Genes Genomics 2022;44:539-50. [Crossref] [PubMed]
- Zhang F, Lu Y, Wang M, et al. Exosomes derived from human bone marrow mesenchymal stem cells transfer miR-222-3p to suppress acute myeloid leukemia cell proliferation by targeting IRF2/INPP4B. Mol Cell Probes 2020;51:101513. [Crossref] [PubMed]
- Takayama Y, Kusamori K, Nishikawa M. Mesenchymal stem/stromal cells as next-generation drug delivery vehicles for cancer therapeutics. Expert Opin Drug Deliv 2021;18:1627-42. [Crossref] [PubMed]
- Yoon AR, Rivera-Cruz C, Gimble JM, et al. Immunotherapy by mesenchymal stromal cell delivery of oncolytic viruses for treating metastatic tumors. Mol Ther Oncolytics 2022;25:78-97. [Crossref] [PubMed]
- Abusarah J, Khodayarian F, El-Hachem N, et al. Engineering immunoproteasome-expressing mesenchymal stromal cells: A potent cellular vaccine for lymphoma and melanoma in mice. Cell Rep Med 2021;2:100455. [Crossref] [PubMed]
- Bikorimana JP, El-Hachem N, El-Kadiry AE, et al. Thymoproteasome-Expressing Mesenchymal Stromal Cells Confer Protective Anti-Tumor Immunity via Cross-Priming of Endogenous Dendritic Cells. Front Immunol 2020;11:596303. [Crossref] [PubMed]
- Salame N, Bikorimana JP, El-Hachem N, et al. UM171A-induced ROS promote antigen cross-presentation of immunogenic peptides by bone marrow-derived mesenchymal stromal cells. Stem Cell Res Ther 2022;13:16. [Crossref] [PubMed]
- Mardani F, Saad W, El-Hachem N, et al. LSD1 Inhibition Enhances the Immunogenicity of Mesenchymal Stromal Cells by Eliciting a dsRNA Stress Response. Cells 2022;11:1816. [Crossref] [PubMed]
- Zipori D. Mesenchymal stem cells: harnessing cell plasticity to tissue and organ repair. Blood Cells Mol Dis 2004;33:211-5. [Crossref] [PubMed]
- Harrell CR, Volarevic A, Djonov VG, et al. Mesenchymal Stem Cell: A Friend or Foe in Anti-Tumor Immunity. Int J Mol Sci 2021;22:12429. [Crossref] [PubMed]
- Bartosh TJ, Ullah M, Zeitouni S, et al. Cancer cells enter dormancy after cannibalizing mesenchymal stem/stromal cells (MSCs). Proc Natl Acad Sci U S A 2016;113:E6447-56. [Crossref] [PubMed]
- García-Castro J, Alemany R, Cascalló M, et al. Treatment of metastatic neuroblastoma with systemic oncolytic virotherapy delivered by autologous mesenchymal stem cells: an exploratory study. Cancer Gene Ther 2010;17:476-83. [Crossref] [PubMed]
- von Einem JC, Guenther C, Volk HD, et al. Treatment of advanced gastrointestinal cancer with genetically modified autologous mesenchymal stem cells: Results from the phase 1/2 TREAT-ME-1 trial. Int J Cancer 2019;145:1538-46. [Crossref] [PubMed]
- Ruano D, López-Martín JA, Moreno L, et al. First-in-Human, First-in-Child Trial of Autologous MSCs Carrying the Oncolytic Virus Icovir-5 in Patients with Advanced Tumors. Mol Ther 2020;28:1033-42. [Crossref] [PubMed]
- Squillaro T, Peluso G, Galderisi U. Clinical Trials With Mesenchymal Stem Cells: An Update. Cell Transplant 2016;25:829-48. [Crossref] [PubMed]
- Scopetti M, Santurro A, Gatto V, et al. Mesenchymal stem cells in neurodegenerative diseases: Opinion review on ethical dilemmas. World J Stem Cells 2020;12:168-77. [Crossref] [PubMed]
- Chen Y, Yu Q, Hu Y, et al. Current Research and Use of Mesenchymal Stem Cells in the Therapy of Autoimmune Diseases. Curr Stem Cell Res Ther 2019;14:579-82. [Crossref] [PubMed]
- Chen C, Lou Y, Li XY, et al. Mapping current research and identifying hotspots on mesenchymal stem cells in cardiovascular disease. Stem Cell Res Ther 2020;11:498. [Crossref] [PubMed]
- Chen R, Hao Z, Wang Y, et al. Mesenchymal Stem Cell-Immune Cell Interaction and Related Modulations for Bone Tissue Engineering. Stem Cells Int 2022;2022:7153584. [Crossref] [PubMed]
(English Language Editors: C. Mullens and J. Gray)