Use of thoracic ultrasound in acute respiratory distress syndrome
Introduction
Acute respiratory distress syndrome (ARDS) is defined as noncardiogenic pulmonary edema that leads to alveolar shunt and gas-exchange impairment (1). According to recent findings, ARDS may represent up to 10% of intensive care unit (ICU) admissions, but it is still underrecognized (2). Lung protective mechanical ventilation, positive end-expiratory pressure (PEEP), prone positioning, and restrictive fluid therapy have become cornerstones of ARDS clinical management (3-6). However, the response to these therapeutic strategies may vary, and it is poorly predictable.
According to the 2012 Berlin definition, the diagnosis of ARDS is based on the onset of hypoxemia and bilateral chest opacities within 1 week of a known clinical insult, which is not fully explained by cardiac failure or fluid overload (1). Bilateral infiltrates remain one of the hallmarks of ARDS diagnosis and may be detected by computed tomography (CT) and X-ray. However, these imaging techniques have several limitations. Chest X-ray have only moderate inter-observer reliability (7). While CT scan has proven to be more accurate than chest radiography, the risk of transporting severely ill patients from and to the ICU must be considered. Furthermore, limited accessibility to these techniques in resource-constrained settings can hinder the identification of ARDS (8).
Alternative imaging techniques have the potential to be useful tools for the diagnosis and therapeutic management of patients with ARDS. Among these techniques, ultrasound evaluation of the heart and lung has been widely implemented in the critical care setting (9). Bedside lung ultrasound (LUS) offers several advantages, including avoiding the need for transportation and radiation, as well as superior detection of lung pathologic abnormalities associated with ARDS when compared to auscultation and chest radiography (10). Since its first description in 1968 (11), the clinical utility of LUS has been questioned for many years because the impedance difference between tissue and air was considered a limiting factor for ultrasound examination. In recent decades, it has become an indispensable bedside tool for managing critically ill patients. International guidelines and consensus recommend the use of LUS in ICUs and emergency departments (11-13). Echocardiography has also become an essential tool for diagnosis, monitoring and guiding clinical decisions in the critical care setting (9). It allows assessment of left and right ventricular systolic function, filling pressures, preload responsiveness and pulmonary hypertension. A combination of LUS and echocardiography provide valuable information for patients with ARDS at the bedside. Recently, ultrasound has been used to evaluate respiratory muscle function in specific clinical scenarios, such as difficult weaning.
In this article, we review the use of thoracic ultrasound, including lung and diaphragm examination, for the clinical assessment of patients with ARDS, and we discuss its limitations and future perspectives.
Basic ultrasound examination
Probe selection
Different probes may be used for LUS. The choice depends on the patient’s anatomy and the suspected abnormality to be assessed (14). Linear high-frequency probes are suitable for pleural examinations, particularly in patients with thin chest walls and during the examination of the anterior part of the chest. Phased-array and convex low-frequency probes are more convenient for exploring lateral and posterior regions and can be helpful in patients with a thick chest wall for assessing abnormalities that occur in deeper areas of the lung.
Ultrasound examination
Ideally, LUS examination should be conducted according to a systematic protocol. Several approaches have been proposed to explore the lung. First, the Bedside Lung Ultrasound in Emergency (BLUE) protocol was developed for the diagnosis of acute respiratory failure (ARF) in the Emergency setting (15). It defines 3 points of interest per lung (BLUE points): upper and lower BLUE points in the anterior wall, and the posterolateral alveolar and/or pleural syndrome (PLAPS), i.e., “PLAPS point”. A twelve-region examination allows for a quantitative approach of lung aeration and is generally used in ICUs (16-20). Each chest wall can be divided into three areas using anterior and posterior-axillary lines as anatomical landmarks (anterior, lateral, and posterior), wherein each area is divided in two regions, namely, superior and inferior regions. In each region of interest, the lung surface of all adjacent intercostal spaces must be explored by moving the probe transversally (19). Dorsal lung segments of the upper lobes, located behind the scapula, are the only regions that cannot be explored by LUS.
LUS findings
A normal ultrasound pattern is defined by lung sliding and regularly spaced horizontal lines (A-lines) (Figure 1). A-lines correspond to reverberation artifacts of the pleural line and indicate the presence of a high gas-volume ratio. Thus, they can be seen in normally aerated lungs, as well as in hyperinflated lungs and pneumothorax.
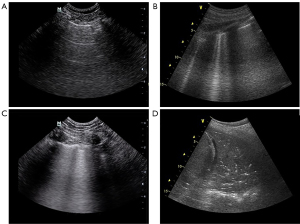
As the lung loses aeration, the LUS pattern varies from A-lines to B-lines. B-lines result from the widening of the pulmonary interlobular septa by either fluid accumulation (from increased hydrostatic pressure or altered capillary permeability), inflammation, or fibrosis. They have been described as a sign of alveolar-interstitial syndrome (20). The number of B-lines depends on the degree of aeration loss. More than two B-lines per scan make a B-pattern and correlate with thickened interlobular septa, whereas five or more correlate with ground-glass areas (18,21). Coalescent B-lines correspond to severe lung aeration loss resulting from partial filling of alveolar spaces.
Lung consolidation results from massive aeration loss. It appears as a tissue-like echotexture, with a superficial boundary at the pleural line and a deep irregular boundary with the aerated lung called the “shred sign” (22). When the hole lobe is involved, this boundary is regular.
Diaphragm ultrasound
There are two methods for evaluating diaphragm function (23) (Figure 2). First, the intercostal approach allows for assessing diaphragm thickness and thickened fraction. (23). Thickening fraction (TFdi) is calculated as the percentage inspiratory increase in diaphragm thickness relative to end-expiratory thickness [TFdi = (end-inspiratory thickness − end-expiratory thickness)/end-expiratory thickness × 100%]. It reflects the contractile activity of the diaphragm (24). However, recent consensus statement remarks the controversy existing regarding cut-off value for diaphragm dysfunction assessment and extubation failure prediction (25).
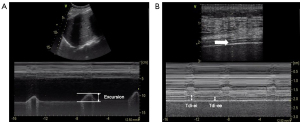
The diaphragmatic excursion is measured using a subcostal approach (24). The excursion is quantified in M-mode, with the patient in a semi-seated position, and should only be measured during unassisted breathing, as active contraction of the diaphragm cannot be distinguished from passive displacement due to ventilator inspiratory pressures (26).
Ultrasound assessment of accessory inspiratory (parasternal intercostal muscle) and expiratory (rectus abdominis, external oblique, internal oblique, and transversus abdominis) muscles could add useful information regarding the patient’s inspiratory effort, patient-ventilator interaction, and expiratory muscle effort (23,27). The parasternal intercostal thickening fraction and its ratio to the diaphragm thickening have been shown to be good predictors of the need for reintubation (28).
Echocardiography
Echocardiography is frequently used in the critical care field. Echocardiographic examination technic description is beyond the aim of this review due to the complexity and the amount of information that can be obtained. Briefly, critical care echocardiography (CCE) can be divided into basic and advanced skill sets, and it can be performed using either a transthoracic (TTE) or a transesophageal (TEE) approach (29). CCE allows the intensivist to define the pathophysiology of respiratory failure and rule out the involvement of any cardiogenic mechanism. It also provides a comprehensive assessment of hemodynamics when respiratory and circulatory failure co-exist and could be used to guide fluid resuscitation (30) and modify ventilator settings.
Initial approach in ARDS patients
ARDS diagnosis
ARDS is not a specific disease, and its current definition is based on clinical criteria (1). Its diagnosis requires a new or worsening respiratory distress within seven days from a clinical insult, impaired oxygenation, and bilateral chest radiographical abnormalities. These radiographical abnormalities may be identified by chest-X-ray or CT scan.
In ARDS patients, lung CT is considered to be the gold standard imaging technique for evaluating lung morphology and performing a quantitative analysis of lung tissue aeration (31). Moreover, it can identify other abnormalities, such as pleural effusion, atelectasis, and nodules (32), and can detect respiratory complications. Nevertheless, CT has significant limitations in the critical care setting, as it employs ionizing radiation and requires patient transfer. It is a costly resource that may not be readily available at all hospitals (33).
LUS has emerged as a promising alternative imaging technique that could potentially aid in identifying the underlying cause in patients with ARF. It has shown high sensitivity and specificity in detecting various lung and pleural diseases, including pleural effusion, pneumothorax, consolidation, and alveolar-interstitial syndrome (15,22,34-40).
Several studies have addressed the ability of LUS to differentiate ARDS from acute pulmonary edema in patients with alveolar-interstitial syndrome (41). When applied to particular clinical settings, LUS findings may help rapidly rule out the most frequent causes of ARF. In the BLUE protocol (15), for instance, LUS signs are used to build up different profiles, which may lead to the cause of ARF in patients presenting to the emergency department with dyspnea. ARDS patients typically show multiple non-homogeneous B lines with a non-gravity-dependent distribution that may coexist with spared areas, pleural thickening with decreased or abolished lung sliding, and subpleural or translobar consolidations (42). Demonstration of this dyshomogeneous sonographic pattern has been reported to strongly predict non-cardiogenic pulmonary edema in its early stages (41). Recently, an ultrasound-based definition of ARDS has been proposed for its diagnosis in a resource-constrained setting (8). The Kigali modification considers LUS findings, rather than chest X-ray or CT. However, it should be noted that ultrasound signs are not specific to ARDS. Patients with diffuse parenchymal lung diseases may also show a B-line pattern with thickened pleural line and reduced or abolished lung sliding (43). Ultrasound examination should, therefore, be integrated with a clinical evaluation to optimize the diagnostic accuracy (44).
LUS could also become a reliable tool for early detection of the most common complications of mechanical ventilation (20). It shows high specificity and sensitivity for the diagnosis of pleural effusion, pneumothorax (36), ventilator-associated pneumonia (45), and atelectasis (46).
During the coronavirus disease (COVID-19) pandemic, LUS emerged as a valuable diagnostic tool, aiding in early diagnosis, therapeutic decision-making, and follow-up monitoring of patients with COVID-19 pneumonia (47), thereby potentially reducing the use of conventional diagnostic imaging resources (CT scan and chest X-ray). Notable LUS findings in COVID-19 patients include various forms of B-lines, an irregular or fragmented pleural line, consolidations, pleural effusions, and absence of lung sliding (48-50).
Evaluation of lung aeration
LUS provides a noninvasive and reproducible imaging method of assessing lung aeration at the bedside. The different degrees of aeration may be semiquantitatively evaluated according to the LUS findings (Figure 1). Alternative rating systems have been proposed. Bouhemad et al. (20) described a score based on four steps in six regions per hemithorax: (I) A-lines correspond to the normal aeration of the lung parenchyma, (II) multiple and well-separated B-lines correspond to a moderate decrease in lung aeration resulting from interstitial syndrome, (III) coalescent B-lines correspond to a more severe decrease in aeration resulting from alveolar involvement, and (IV) consolidation corresponds to complete loss of aeration. The global LUS score corresponds to the sum of six regions’ score in each hemithorax and ranges from 0 to 36. Variations of these patterns may be used to express both loss of aeration and re-aeration in different clinical settings (16,20,51).
A correlation between LUS score and extravascular lung water assessed by transpulmonary thermodilution (52), as well as overall lung tissue density assessed by quantitative CT imaging (53), has been observed in ARDS patients. However, recent studies reported only a moderate correlation with transpulmonary thermodilution techniques in an unselected population of critically ill patients. The heterogeneity of the findings may be related to the varying distribution pattern of lung water for different underlying pathologies, which may be influenced by different factors, such as hemodynamics, capillary integrity, and renal function (54). Notably, determining and interpretating B-lines at the bedside can be difficult and highly variable (49).
The application of LUS for assessing lung aeration in ARDS patients has some limitations (55). First, the width of the intercostal space limits pleura visualization and may vary among patients when performing the study with a longitudinal orientation. Second, coalescent B lines indicate severe loss of aeration and have been associated with increased lung density in homogeneous diseases (51). However, non-homogenous diseases, such as ARDS, may also present focal coalescent B lines and subpleural consolidations. Additionally, complete loss of aeration is attributed to a lung region whenever a tissue-like pattern is observed, independently of its size, which may result in an overestimation of loss of aeration when this pattern involves only a small portion of the visualized lung region (17).
Recently, Mongodi et al. proposed a modified score to further improve the accuracy of ultrasound assessment of lung aeration (55). This score considers the percentage of involved pleura to establish the severity of loss of aeration. The use of a high-frequency linear probe in a traverse orientation aligned with the intercostal space enables visualization of a longer pleura length. Furthermore, the visualization of LUS signs, such as subpleural consolidation, may improve diagnostic accuracy. This score seems to perform better than the traditional one, but further research is needed to confirm its efficacy.
The use of LUS monitoring may be of special interest in severe ARDS patients on extracorporeal membrane oxygenation, in which a chest X-ray may be poorly informative (56). LUS score monitoring can provide valuable information regarding potential lung recovery, with a persistent high score indicating a negative outcome.
Additionally, LUS can not only quantify, but can also assess the distribution of aeration loss, which can guide mechanical ventilation settings and other possible strategies. In patients with focal ARDS, LUS shows a heterogeneous pattern with consolidation or B-lines, mainly in lower posterior regions, while anterior lung regions remain more aerated (14). In such cases, high PEEP levels may lead to overdistension of normal parenchyma (57), but prone positioning may yield adequate responses (58). Conversely, in patients with diffuse ARDS, aeration loss involves all regions homogeneously, and increasing levels of PEEP may lead to improvements. LUS can identify changes in aeration patterns induced by increases in PEEP (20).
Circulatory failure and resuscitation
Patients with ARDS may benefit from conservative fluid management (6), as fluid overload can contribute to worse outcomes (59). However, most ARDS cases are associated with hemodynamic instability, making appropriate resuscitation of patients with shock is a crucial factor in determining outcomes (60), especially in patients with both ARDS and circulatory failure.
CCE is a valuable bedside tool for hemodynamic monitoring. It is used in shock patients to evaluate cardiac function and guide fluid resuscitation, and it can be used to obtain repeated measurements to assess changes of different therapies on cardiac output (CO) (61). Briefly, using the Doppler technique, stroke volume is estimated by multiplying the velocity of time integral (VTI) of the blood flow in the left ventricle outflow track (LVOT) by the cross-sectional area of the aortic valve [0.785× (diameter)2]. Since the aortic diameter does not change, VTILVOT is a reliable parameter and can easily be used to track changes in stroke volume (62).
Fluid responsiveness may be assessed by CCE using dynamic parameters based on heart-lung interactions in mechanically ventilated patients (63). The magnitude of the effect depends mainly on the transmission of airway pressure variations to the heart. Doppler measurement of ascending aortic flow and VTILVOT may be obtained in the apical five-chamber view (TTE) and the deep gastric view (TEE). The variation of VTILVOT(DVTI) can be calculated as follows: DVTI (%) = (VTImax – VTImin)/((VTImax + VTImin)/2) × 100%, where VTImax and VTImin represent the maximum and minimum VTI in 10 cardiac cycles. Variations in aortic flow velocity greater than 12% throughout the respiratory cycle accurately predict fluid responsiveness (64). Similarly, variations in VTI also predict responsiveness (65).
TEE and TTE also allow measures of inferior and superior vena cava diameter and variation, and cardiac output changes in response to passive leg raising.
Clinicians with advanced skills in transthoracic echocardiography can also obtain a Doppler measurement of the maximal ascending aortic flow velocity (just distal to the aortic valve) immediately before and after performing a PLR.
How LUS can be used to personalize MV settings in the acute phase of ARDS?
Changes in lung aeration
Computed tomography is considered the gold standard for assessing lung morphology and recruitment in ARDS patients; however, it has several limitations, including high irradiation exposure and patient transportation (66-69). LUS may be a safe and feasible tool for lung recruitment assessment in real-time at the bedside and could be considered as an alternative to CT scan. It can identify re-aeration as a sequential change in the pattern from consolidation to B-lines and ultimately A-lines. However, consolidation may transform directly into a normal pattern (20). Similarly, LUS can detect lung collapse with high specificity and sensitivity (18).
Ultrasound assessment of aeration changes in ARDS patients was first reported using TEE and LUS (70-72). Although these studies were limited to non-aerated dependent regions, they demonstrated significant correlations between the reduction of the consolidated areas and the improvement of oxygenation. Bouhemad et al. reported a significant correlation between PEEP-induced lung recruitment based on ultrasound reaeration score and pressure-volume (PV) curve (20). The LUS score is based on the examination of all intercostal spaces of 12 regions of interest: upper and lower parts of anterior, lateral, and posterior regions of both the left and right chest. The worst ultrasound abnormality detected in each region characterizes the region examined. Four ultrasound aeration patterns were defined: (I) normal aeration (N), presence of lung sliding with A lines or fewer than two isolated B lines; (II) moderate loss of lung aeration, multiple well-defined B lines (B1 lines); (III) severe loss of lung aeration, multiple coalescent B lines (B2 lines); and lung consolidation (C), the presence of a tissue pattern characterized by dynamic air bronchograms. The LUS score ranges from 0 to 36 and is calculated as the sum of points. An ultrasound reaeration score can also be calculated from changes in the ultrasound pattern in each region, allowing regional analysis. Recruitment mainly results from the reaeration of poorly aerated lung regions (coalescent and separated B-lines) rather than from consolidations.
As has already been proposed (73), a practical approach based on LUS could be used to personalized recruitment at the bedside. First, LUS can be used to assess the degree and distribution of loss of aeration, to consider the potential for recruitment. Second, it may help to guide and individualize PEEP settings based on lung morphology. LUS may identify closing pressure during a step-wise decremental PEEP trial.
Although LUS cannot identify lung overinflation, severely decreased or abolished lung sliding may be associated with the impaired regional ventilation associated with high PEEP settings (74), suggesting PEEP-induced hyperinflation in non-dependent lung regions, especially if lung sliding reappears when PEEP is decreased (42).
Similarly, ultrasonography may be used to follow-up ventilator-associated pneumonia (VAP). Changes in aeration pattern (disappearance of B-lines and subpleural consolidation) have been correlated with CT scan improvements and may be associated with antibiotic efficacy (16).
Diaphragm-protective ventilation
Diaphragm atrophy and injury caused by mechanical ventilation have been associated with poor clinical outcomes (75). The concept of diaphragm-protective ventilation has recently emerged and encourages physicians to monitor and optimize respiratory effort and synchrony with the aim of preventing diaphragm atrophy and muscle injury while maintaining lung protection (76). Diaphragm ultrasound may be used to assess respiratory effort. It has been suggested that a diaphragm thickening fraction in the range of 15–30% may be associated with the shortest duration of mechanical ventilation compared to lower or higher thickening fraction values (77). However, controversy remains regarding cut-off values for assessing respiratory effort and regarding the effect that positive pressure ventilation may have on patient effort (26).
Right ventricle assessment
ARDS is one of the most common causes of acute right ventricular failure (RVF) in the critical care setting (78). Acute cor pulmonale (ACP) is the most severe presentation of RVF due to an acute increase in RV afterload. It occurs in 25% of ARDS patients under protective mechanical ventilation (79,80) and is associated with poor outcomes (78,81). Underlying mechanisms include lung inflammation, pulmonary artery injury and the effects of positive pressure ventilation (82). Pneumonia (as the cause of ARDS), driving pressure ≤18 cmH2O, arterial oxygen partial pressure to fractional inspired oxygen ratio (PaO2/FiO2) ≤150 mmHg, and arterial carbon dioxide partial pressure (PaCO2) ≥48 mmHg have been reported to be associated with ACP (81). Acute right ventricular (RV) after loading may lead to patent foramen oval (PFO). The prevalence of PFO in ARDS ranges between 16% and 19% (83,84). Although PFO may reduce the harmful effects of elevated pulmonary vascular resistance on RV systolic function, it may worsen hypoxemia and limit the beneficial effects of recruitment maneuvers and PEEP (83,85).
It has been proposed that early assessment of RV function would be important to best tailor clinical management and prevent further injury to the RV (81). The diagnosis of ACP and PFO relies on echocardiography. It facilitates non-invasive assessment of RV preload, contractility, and afterload (86), as well as monitoring of the response to different strategies at the bedside. A RV protective approach, based on echocardiographic monitoring, has been suggested by Vieillard-Baron et al. (87), involving adapting the therapeutic lung protective ventilation strategy according to the function of the right ventricle. However, it remains unclear whether this “RV protective approach” would be associated with better outcomes compared to a more conventional approach (80).
How may LUS be helpful during the weaning from mechanical ventilation?
Weaning from mechanical ventilation can be considered as an exercise test and induces significant changes in lung aeration and cardiac function (88). The cause of weaning failure is often multifactorial. Spontaneous breathing may lead to an increase in aeration of posterior and dependent lung regions due to the active contraction of the diaphragm, whereas nondependent lung regions may decrease their aeration (89). Lung aeration score changes during spontaneous breathing trial (SBT) may predict extubation success or failure. A LUS score >17 at the end of the SBT has been reported to predict post-extubation distress (89). LUS can also be used to assess pleural effusion, which may also play a role in weaning failure. Ferré et al. also reported that an increase in the number of B-lines allows the diagnosis of weaning-induced pulmonary edema (90).
CCE may be helpful for evaluating left ventricle systolic and diastolic function, which are associated with weaning failure (91). It may accurately identify patients at high risk of weaning failure, but the ability to predict failure remains uncertain.
Diaphragmatic dysfunction is common in critically ill patients and is associated with prolonged mechanical ventilation and length of stay. Ultrasound measurement of excursion and thickening fraction seems to strongly correlate with diaphragm strength (27). However, conflicting results have been reported regarding its ability to predict successful weaning (92-95). A significant proportion of patients can be successfully extubated despite diaphragm dysfunction (94,96). Recently, the predictive value of diaphragm ultrasound was also tested in mechanically ventilated COVID-19 patients, but TFdi was not predictive of weaning failure (97). Lung aeration loss appears more frequently in patients with diaphragm dysfunction; thus, even when diaphragm dysfunction is detected, physicians should be encouraged to investigate other causes of failure (98). Nevertheless, it remains uncertain whether diaphragm assessment adds to clinical decision-making.
A multimodal approach, integrating LUS, diaphragm ultrasound, and echocardiography findings, may help identify patients at risk and predict SBT failure (88,99). Although a holistic ultrasound approach has previously been determined to be a weak predictor for extubation failure (100), understanding the pathophysiology of weaning failure can help physicians to optimize the clinical status and physiological function before proceeding to mechanical ventilation discontinuation. A weaning trial can be considered as a cardio-pulmonary stress test. Therefore, failure may be the result of a combination of cardiac dysfunction, impaired gas exchange, and/or diaphragmatic dysfunction. Tuinman et al. proposed a standardized sonographic approach to weaning, including LUS aeration score, pleural effusion and ascites assessment, CCE, and evaluation of diaphragm and extra-diaphragmatic respiratory muscles (23). The ultrasound examination may be performed before the SBT for the identification of patients at high risk of weaning failure and during the SBT for prediction of weaning outcome or to diagnose the cause of failure.
Limitations
Ultrasound examination has several limitations. It is an operator-dependent technique that requires training for image acquisition and interpretation, as well as appropriate integration with the clinical scenario. Simple LUS examination can be easily acquired with short training and is useful for detecting some abnormalities, such as pleural effusion and ruling out pneumothorax (101,102). Moreover, ultrasonographic aeration assessment has shown a strong interobserver agreement (53,55). For more advanced skills, such as lung ultrasound score computation and diaphragm function assessment, longer training may be required (103). CCE ranges from basic practice, which is used to rapidly characterize causes of shock and respiratory failure, to advanced CCE, which entails the performance of comprehensive diagnostic studies similar to the ones expected of a cardiologist, often with additional advanced hemodynamic assessment. Nevertheless, the development of training methods to assess competence is imperative for the safe and effective use of such systems (104).
Ultrasound examination can be difficult due to anatomical characteristics or artificial devices, such as obesity, subcutaneous emphysema, and large thoracic dressings. If the lung is aerated, the examination only allows analysis of the lung surface. Thus, results of LUS examination in diseases that may have no or minimal extension to peripheral fields should be interpreted with caution.
Finally, it should be also noted that LUS cannot detect lung overinflation resulting from an increase in intrathoracic pressures (42). This is a major limitation when assessing lung recruitment in ARDS patients.
Future perspectives
Critical care ultrasound has greatly developed in the last few years and has become essential to the modern practice of critical care medicine. Assessing competency is, therefore, a key point in the training process. Despite the increased use of LUS, training methods to acquire appropriate skills vary among centers and are not standardized (103). Specific and standardized learning programs and examinations should be part of the basic knowledge of all intensivists.
LUS allows a repeatable semiquantitative assessment of lung aeration at the bedside. As stated before, the current scoring system shows some limitations and leaves room for improvement (53,55,105). Automation of lung aeration based on computer-assisted gray-scale analysis has also been described (106), with the advantage that it would be operator-independent. Further studies are needed to assess the clinical utility of this automatic assessment of lung aeration.
Progress has been made in ultrasound technology, such as three-dimensional ultrasound and miniaturization. Pocket-sized ultrasound devices have been recently developed and have a relatively low cost, but image quality is lower than that of using traditional devices. Nevertheless, they are suited for bedside use in emergency settings.
The diagnostic accuracy of ultrasonography has been extensively reported. However, the influence on clinical decision-making and outcomes remains uncertain and needs further investigation.
Finally, whether personalizing mechanical ventilation—according to the lung morphology assessment performed during LUS—improves clinical outcomes in ARDS patients is currently under evaluation (NCT05492344).
Conclusions
Ultrasound is a non-invasive bedside radiation-free technique that has become widely available and highly feasible, and it can be easily applied in the critical care setting. A combination of LUS, echocardiography, and diaphragm ultrasound provides valuable physiological information in ARDS patients. This information could be used to personalize ventilator settings at the bedside. Moreover, ultrasound-based hemodynamic variables could improve fluid resuscitation. Finally, ultrasound techniques could inform about the possible causes of weaning failure. However, despite being physiologically attractive, whether clinical decisions based on ultrasound assessment may improve outcomes in ARDS patients remains uncertain and needs further investigation.
Acknowledgments
Funding: None.
Footnote
Provenance and Peer Review: This article was commissioned by the Guest Editors (Tobias Eckle and Dr. Benjamin Scott) for the series “Highlights in Anesthesia and Critical Care Medicine” published in Annals of Translational Medicine. The article has undergone external peer review.
Peer Review File: Available at https://atm.amegroups.com/article/view/10.21037/atm-22-4576/prf
Conflicts of Interest: All authors have completed the ICMJE uniform disclosure form (available at https://atm.amegroups.com/article/view/10.21037/atm-22-4576/coif). The series “Highlights in Anesthesia and Critical Care Medicine” was commissioned by the editorial office without any funding or sponsorship. The authors have no other conflicts of interest to declare.
Ethical Statement: The authors are accountable for all aspects of the work in ensuring that questions related to the accuracy or integrity of any part of the work are appropriately investigated and resolved.
Open Access Statement: This is an Open Access article distributed in accordance with the Creative Commons Attribution-NonCommercial-NoDerivs 4.0 International License (CC BY-NC-ND 4.0), which permits the non-commercial replication and distribution of the article with the strict proviso that no changes or edits are made and the original work is properly cited (including links to both the formal publication through the relevant DOI and the license). See: https://creativecommons.org/licenses/by-nc-nd/4.0/.
References
- Acute respiratory distress syndrome: the Berlin Definition, et al. Acute respiratory distress syndrome: the Berlin Definition. JAMA 2012;307:2526-33.
- Bellani G, Laffey JG, Pham T, et al. Epidemiology, Patterns of Care, and Mortality for Patients With Acute Respiratory Distress Syndrome in Intensive Care Units in 50 Countries. JAMA 2016;315:788-800. [Crossref] [PubMed]
- Brower RG, Matthay MA, Morris A, et al. Ventilation with lower tidal volumes as compared with traditional tidal volumes for acute lung injury and the acute respiratory distress syndrome. N Engl J Med 2000;342:1301-8. [Crossref] [PubMed]
- Briel M, Meade M, Mercat A, et al. Higher vs lower positive end-expiratory pressure in patients with acute lung injury and acute respiratory distress syndrome: systematic review and meta-analysis. JAMA 2010;303:865-73. [Crossref] [PubMed]
- Guérin C, Reignier J, Richard JC, et al. Prone positioning in severe acute respiratory distress syndrome. N Engl J Med 2013;368:2159-68. [Crossref] [PubMed]
- Wiedemann HP, Wheeler AP, Bernard GR, et al. Comparison of two fluid-management strategies in acute lung injury. N Engl J Med 2006;354:2564-75. [Crossref] [PubMed]
- Meade MO, Cook RJ, Guyatt GH, et al. Interobserver variation in interpreting chest radiographs for the diagnosis of acute respiratory distress syndrome. Am J Respir Crit Care Med 2000;161:85-90. [Crossref] [PubMed]
- Riviello ED, Kiviri W, Twagirumugabe T, et al. Hospital Incidence and Outcomes of the Acute Respiratory Distress Syndrome Using the Kigali Modification of the Berlin Definition. Am J Respir Crit Care Med 2016;193:52-9. [Crossref] [PubMed]
- Vieillard-Baron A, Millington SJ, Sanfilippo F, et al. A decade of progress in critical care echocardiography: a narrative review. Intensive Care Med 2019;45:770-88. [Crossref] [PubMed]
- Lichtenstein D, Goldstein I, Mourgeon E, et al. Comparative diagnostic performances of auscultation, chest radiography, and lung ultrasonography in acute respiratory distress syndrome. Anesthesiology 2004;100:9-15. [Crossref] [PubMed]
- Ross AM, Genton E, Holmes JH. Ultrasonic examination of the lung. J Lab Clin Med 1968;72:556-64. [PubMed]
- Mayo PH, Beaulieu Y, Doelken P, et al. American College of Chest Physicians/La Société de Réanimation de Langue Française statement on competence in critical care ultrasonography. Chest 2009;135:1050-60. [Crossref] [PubMed]
- Robba C, Wong A, Poole D, et al. Basic ultrasound head-to-toe skills for intensivists in the general and neuro intensive care unit population: consensus and expert recommendations of the European Society of Intensive Care Medicine. Intensive Care Med 2021;47:1347-67. [Crossref] [PubMed]
- Bouhemad B, Mongodi S, Via G, et al. Ultrasound for "lung monitoring" of ventilated patients. Anesthesiology 2015;122:437-47. [Crossref] [PubMed]
- Lichtenstein DA, Mezière GA. Relevance of lung ultrasound in the diagnosis of acute respiratory failure: the BLUE protocol. Chest 2008;134:117-25. [Crossref] [PubMed]
- Bouhemad B, Liu ZH, Arbelot C, et al. Ultrasound assessment of antibiotic-induced pulmonary reaeration in ventilator-associated pneumonia. Crit Care Med 2010;38:84-92. [Crossref] [PubMed]
- Mojoli F, Bouhemad B, Mongodi S, et al. Lung Ultrasound for Critically Ill Patients. Am J Respir Crit Care Med 2019;199:701-14. [Crossref] [PubMed]
- Bouhemad B, Brisson H, Le-Guen M, et al. Bedside ultrasound assessment of positive end-expiratory pressure-induced lung recruitment. Am J Respir Crit Care Med 2011;183:341-7. [Crossref] [PubMed]
- Bouhemad B, Zhang M, Lu Q, et al. Clinical review: Bedside lung ultrasound in critical care practice. Crit Care 2007;11:205. [Crossref] [PubMed]
- Lichtenstein D, Mézière G, Biderman P, et al. The comet-tail artifact. An ultrasound sign of alveolar-interstitial syndrome. Am J Respir Crit Care Med 1997;156:1640-6. [Crossref] [PubMed]
- Volpicelli G, Elbarbary M, Blaivas M, et al. International evidence-based recommendations for point-of-care lung ultrasound. Intensive Care Med 2012;38:577-91. [Crossref] [PubMed]
- Lichtenstein DA, Lascols N, Mezière G, et al. Ultrasound diagnosis of alveolar consolidation in the critically ill. Intensive Care Med 2004;30:276-81. [Crossref] [PubMed]
- Tuinman PR, Jonkman AH, Dres M, et al. Respiratory muscle ultrasonography: methodology, basic and advanced principles and clinical applications in ICU and ED patients-a narrative review. Intensive Care Med 2020;46:594-605. [Crossref] [PubMed]
- Matamis D, Soilemezi E, Tsagourias M, et al. Sonographic evaluation of the diaphragm in critically ill patients. Technique and clinical applications. Intensive Care Med 2013;39:801-10. [Crossref] [PubMed]
- Goligher EC, Laghi F, Detsky ME, et al. Measuring diaphragm thickness with ultrasound in mechanically ventilated patients: feasibility, reproducibility and validity. Intensive Care Med 2015;41:642-9. [Crossref] [PubMed]
- Haaksma ME, Smit JM, Boussuges A, et al. EXpert consensus On Diaphragm UltraSonography in the critically ill (EXODUS): a Delphi consensus statement on the measurement of diaphragm ultrasound-derived parameters in a critical care setting. Crit Care 2022;26:99. [Crossref] [PubMed]
- Dubé BP, Dres M, Mayaux J, et al. Ultrasound evaluation of diaphragm function in mechanically ventilated patients: comparison to phrenic stimulation and prognostic implications. Thorax 2017;72:811-8. [Crossref] [PubMed]
- Dres M, Similowski T, Goligher EC, et al. Dyspnoea and respiratory muscle ultrasound to predict extubation failure. Eur Respir J 2021;58:2100002. [Crossref] [PubMed]
- Vignon P, Merz TM, Vieillard-Baron A. Ten reasons for performing hemodynamic monitoring using transesophageal echocardiography. Intensive Care Med 2017;43:1048-51. [Crossref] [PubMed]
- Vignon P, Repessé X, Vieillard-Baron A, et al. Critical care ultrasonography in acute respiratory failure. Crit Care 2016;20:228. [Crossref] [PubMed]
- Gattinoni L, Caironi P, Pelosi P, et al. What has computed tomography taught us about the acute respiratory distress syndrome? Am J Respir Crit Care Med 2001;164:1701-11. [Crossref] [PubMed]
- Putman RK, Hunninghake GM, Dieffenbach PB, et al. Interstitial Lung Abnormalities Are Associated with Acute Respiratory Distress Syndrome. Am J Respir Crit Care Med 2017;195:138-41. [Crossref] [PubMed]
- Pesenti A, Tagliabue P, Patroniti N, et al. Computerised tomography scan imaging in acute respiratory distress syndrome. Intensive Care Med 2001;27:631-9. [Crossref] [PubMed]
- Lichtenstein D, Mezière G, Biderman P, et al. The "lung point": an ultrasound sign specific to pneumothorax. Intensive Care Med 2000;26:1434-40. [Crossref] [PubMed]
- Lichtenstein DA, Lascols N, Prin S, et al. The "lung pulse": an early ultrasound sign of complete atelectasis. Intensive Care Med 2003;29:2187-92. [Crossref] [PubMed]
- Lichtenstein DA, Menu Y. A bedside ultrasound sign ruling out pneumothorax in the critically ill. Lung sliding. Chest 1995;108:1345-8. [Crossref] [PubMed]
- Nazerian P, Vanni S, Volpicelli G, et al. Accuracy of point-of-care multiorgan ultrasonography for the diagnosis of pulmonary embolism. Chest 2014;145:950-7. [Crossref] [PubMed]
- Volpicelli G, Boero E, Sverzellati N, et al. Semi-quantification of pneumothorax volume by lung ultrasound. Intensive Care Med 2014;40:1460-7. [Crossref] [PubMed]
- Volpicelli G, Mussa A, Garofalo G, et al. Bedside lung ultrasound in the assessment of alveolar-interstitial syndrome. Am J Emerg Med 2006;24:689-96. [Crossref] [PubMed]
- Chan KK, Joo DA, McRae AD, et al. Chest ultrasonography versus supine chest radiography for diagnosis of pneumothorax in trauma patients in the emergency department. Cochrane Database Syst Rev 2020;7:CD013031. [PubMed]
- Copetti R, Soldati G, Copetti P. Chest sonography: a useful tool to differentiate acute cardiogenic pulmonary edema from acute respiratory distress syndrome. Cardiovasc Ultrasound 2008;6:16. [Crossref] [PubMed]
- Pesenti A, Musch G, Lichtenstein D, et al. Imaging in acute respiratory distress syndrome. Intensive Care Med 2016;42:686-98. [Crossref] [PubMed]
- Reissig A, Kroegel C. Transthoracic sonography of diffuse parenchymal lung disease: the role of comet tail artifacts. J Ultrasound Med 2003;22:173-80. [Crossref] [PubMed]
- Zanobetti M, Scorpiniti M, Gigli C, et al. Point-of-Care Ultrasonography for Evaluation of Acute Dyspnea in the ED. Chest 2017;151:1295-301. [Crossref] [PubMed]
- Mongodi S, Via G, Girard M, et al. Lung Ultrasound for Early Diagnosis of Ventilator-Associated Pneumonia. Chest 2016;149:969-80. [Crossref] [PubMed]
- Lichtenstein D, Mezière G, Seitz J. The dynamic air bronchogram. A lung ultrasound sign of alveolar consolidation ruling out atelectasis. Chest 2009;135:1421-5. [Crossref] [PubMed]
- Allinovi M, Parise A, Giacalone M, et al. Lung Ultrasound May Support Diagnosis and Monitoring of COVID-19 Pneumonia. Ultrasound Med Biol 2020;46:2908-17. [Crossref] [PubMed]
- Volpicelli G, Lamorte A, Villén T. What's new in lung ultrasound during the COVID-19 pandemic. Intensive Care Med 2020;46:1445-8. [Crossref] [PubMed]
- Haaksma ME, Heldeweg MLA, Lopez Matta JE, et al. Lung ultrasound findings in patients with novel SARS-CoV-2. ERJ Open Res 2020;6:00238-2020. [Crossref] [PubMed]
- Lieveld AWE, Kok B, Azijli K, et al. Assessing COVID-19 pneumonia-Clinical extension and risk with point-of-care ultrasound: A multicenter, prospective, observational study. J Am Coll Emerg Physicians Open 2021;2:e12429. [Crossref] [PubMed]
- Via G, Lichtenstein D, Mojoli F, et al. Whole lung lavage: a unique model for ultrasound assessment of lung aeration changes. Intensive Care Med 2010;36:999-1007. [Crossref] [PubMed]
- Zhao Z, Jiang L, Xi X, et al. Prognostic value of extravascular lung water assessed with lung ultrasound score by chest sonography in patients with acute respiratory distress syndrome. BMC Pulm Med 2015;15:98. [Crossref] [PubMed]
- Chiumello D, Mongodi S, Algieri I, et al. Assessment of Lung Aeration and Recruitment by CT Scan and Ultrasound in Acute Respiratory Distress Syndrome Patients. Crit Care Med 2018;46:1761-8. [Crossref] [PubMed]
- Seibel A, Zechner PM, Berghold A, et al. B-Lines for the assessment of extravascular lung water: Just focused or semi-quantitative? Acta Anaesthesiol Scand 2020;64:953-60. [Crossref] [PubMed]
- Mongodi S, Bouhemad B, Orlando A, et al. Modified Lung Ultrasound Score for Assessing and Monitoring Pulmonary Aeration. Ultraschall Med 2017;38:530-7. [Crossref] [PubMed]
- Mongodi S, Pozzi M, Orlando A, et al. Lung ultrasound for daily monitoring of ARDS patients on extracorporeal membrane oxygenation: preliminary experience. Intensive Care Med 2018;44:123-4. [Crossref] [PubMed]
- Constantin JM, Grasso S, Chanques G, et al. Lung morphology predicts response to recruitment maneuver in patients with acute respiratory distress syndrome. Crit Care Med 2010;38:1108-17. [Crossref] [PubMed]
- Prat G, Guinard S, Bizien N, et al. Can lung ultrasonography predict prone positioning response in acute respiratory distress syndrome patients? J Crit Care 2016;32:36-41. [Crossref] [PubMed]
- Casey JD, Semler MW, Rice TW. Fluid Management in Acute Respiratory Distress Syndrome. Semin Respir Crit Care Med 2019;40:57-65. [Crossref] [PubMed]
- Evans L, Rhodes A, Alhazzani W, et al. Surviving Sepsis Campaign: International Guidelines for Management of Sepsis and Septic Shock 2021. Crit Care Med 2021;49:e1063-143. [Crossref] [PubMed]
- Vincent JL, Rhodes A, Perel A, et al. Clinical review: Update on hemodynamic monitoring--a consensus of 16. Crit Care 2011;15:229. [Crossref] [PubMed]
- Wetterslev M, Møller-Sørensen H, Johansen RR, et al. Systematic review of cardiac output measurements by echocardiography vs. thermodilution: the techniques are not interchangeable. Intensive Care Med 2016;42:1223-33. [Crossref] [PubMed]
- Vignon P, Repessé X, Bégot E, et al. Comparison of Echocardiographic Indices Used to Predict Fluid Responsiveness in Ventilated Patients. Am J Respir Crit Care Med 2017;195:1022-32. [Crossref] [PubMed]
- Feissel M, Michard F, Mangin I, et al. Respiratory changes in aortic blood velocity as an indicator of fluid responsiveness in ventilated patients with septic shock. Chest 2001;119:867-73. [Crossref] [PubMed]
- Miller A, Mandeville J. Predicting and measuring fluid responsiveness with echocardiography. Echo Res Pract 2016;3:G1-G12. [Crossref] [PubMed]
- Gattinoni L, D'Andrea L, Pelosi P, et al. Regional effects and mechanism of positive end-expiratory pressure in early adult respiratory distress syndrome. JAMA 1993;269:2122-7. [Crossref] [PubMed]
- Gattinoni L, Pelosi P, Crotti S, et al. Effects of positive end-expiratory pressure on regional distribution of tidal volume and recruitment in adult respiratory distress syndrome. Am J Respir Crit Care Med 1995;151:1807-14. [Crossref] [PubMed]
- Gattinoni L, Caironi P, Valenza F, et al. The role of CT-scan studies for the diagnosis and therapy of acute respiratory distress syndrome. Clin Chest Med 2006;27:559-70; abstract vii. [Crossref] [PubMed]
- Malbouisson LM, Muller JC, Constantin JM, et al. Computed tomography assessment of positive end-expiratory pressure-induced alveolar recruitment in patients with acute respiratory distress syndrome. Am J Respir Crit Care Med 2001;163:1444-50. [Crossref] [PubMed]
- Tsubo T, Yatsu Y, Suzuki A, et al. Daily changes of the area of density in the dependent lung region - evaluation using transesophageal echocardiography. Intensive Care Med 2001;27:1881-6. [Crossref] [PubMed]
- Tsubo T, Yatsu Y, Tanabe T, et al. Evaluation of density area in dorsal lung region during prone position using transesophageal echocardiography. Crit Care Med 2004;32:83-7. [Crossref] [PubMed]
- Stefanidis K, Dimopoulos S, Nanas S. Basic principles and current applications of lung ultrasonography in the intensive care unit. Respirology 2011;16:249-56. [Crossref] [PubMed]
- Tusman G, Acosta CM, Costantini M. Ultrasonography for the assessment of lung recruitment maneuvers. Crit Ultrasound J 2016;8:8. [Crossref] [PubMed]
- Markota A, Golub J, Stožer A, et al. Absence of lung sliding is not a reliable sign of pneumothorax in patients with high positive end-expiratory pressure. Am J Emerg Med 2016;34:2034-6. [Crossref] [PubMed]
- Goligher EC, Brochard LJ, Reid WD, et al. Diaphragmatic myotrauma: a mediator of prolonged ventilation and poor patient outcomes in acute respiratory failure. Lancet Respir Med 2019;7:90-8. [Crossref] [PubMed]
- Goligher EC, Dres M, Patel BK, et al. Lung- and Diaphragm-Protective Ventilation. Am J Respir Crit Care Med 2020;202:950-61. [Crossref] [PubMed]
- Goligher EC, Dres M, Fan E, et al. Mechanical Ventilation-induced Diaphragm Atrophy Strongly Impacts Clinical Outcomes. Am J Respir Crit Care Med 2018;197:204-13. [Crossref] [PubMed]
- Bull TM, Clark B, McFann K, et al. Pulmonary vascular dysfunction is associated with poor outcomes in patients with acute lung injury. Am J Respir Crit Care Med 2010;182:1123-8. [Crossref] [PubMed]
- Boissier F, Katsahian S, Razazi K, et al. Prevalence and prognosis of cor pulmonale during protective ventilation for acute respiratory distress syndrome. Intensive Care Med 2013;39:1725-33. [Crossref] [PubMed]
- Repessé X, Charron C, Vieillard-Baron A. Acute cor pulmonale in ARDS: rationale for protecting the right ventricle. Chest 2015;147:259-65. [Crossref] [PubMed]
- Mekontso Dessap A, Boissier F, Charron C, et al. Acute cor pulmonale during protective ventilation for acute respiratory distress syndrome: prevalence, predictors, and clinical impact. Intensive Care Med 2016;42:862-70. [Crossref] [PubMed]
- Price LC, McAuley DF, Marino PS, et al. Pathophysiology of pulmonary hypertension in acute lung injury. Am J Physiol Lung Cell Mol Physiol 2012;302:L803-15. [Crossref] [PubMed]
- Mekontso Dessap A, Boissier F, Leon R, et al. Prevalence and prognosis of shunting across patent foramen ovale during acute respiratory distress syndrome. Crit Care Med 2010;38:1786-92. [Crossref] [PubMed]
- Lhéritier G, Legras A, Caille A, et al. Prevalence and prognostic value of acute cor pulmonale and patent foramen ovale in ventilated patients with early acute respiratory distress syndrome: a multicenter study. Intensive Care Med 2013;39:1734-42. [Crossref] [PubMed]
- Legras A, Caille A, Begot E, et al. Acute respiratory distress syndrome (ARDS)-associated acute cor pulmonale and patent foramen ovale: a multicenter noninvasive hemodynamic study. Crit Care 2015;19:174. [Crossref] [PubMed]
- Rudski LG, Lai WW, Afilalo J, et al. Guidelines for the echocardiographic assessment of the right heart in adults: a report from the American Society of Echocardiography endorsed by the European Association of Echocardiography, a registered branch of the European Society of Cardiology, and the Canadian Society of Echocardiography. J Am Soc Echocardiogr 2010;23:685-713; quiz 786-8. [Crossref] [PubMed]
- Vieillard-Baron A, Price LC, Matthay MA. Acute cor pulmonale in ARDS. Intensive Care Med 2013;39:1836-8. [Crossref] [PubMed]
- Mayo P, Volpicelli G, Lerolle N, et al. Ultrasonography evaluation during the weaning process: the heart, the diaphragm, the pleura and the lung. Intensive Care Med 2016;42:1107-17. [Crossref] [PubMed]
- Soummer A, Perbet S, Brisson H, et al. Ultrasound assessment of lung aeration loss during a successful weaning trial predicts postextubation distress*. Crit Care Med 2012;40:2064-72. [Crossref] [PubMed]
- Ferré A, Guillot M, Lichtenstein D, et al. Lung ultrasound allows the diagnosis of weaning-induced pulmonary oedema. Intensive Care Med 2019;45:601-8. [Crossref] [PubMed]
- Saleh M, Vieillard-Baron A. On the role of left ventricular diastolic function in the critically ill patient. Intensive Care Med 2012;38:189-91. [Crossref] [PubMed]
- Llamas-Álvarez AM, Tenza-Lozano EM, Latour-Pérez J. Diaphragm and Lung Ultrasound to Predict Weaning Outcome: Systematic Review and Meta-Analysis. Chest 2017;152:1140-50. [Crossref] [PubMed]
- Qian Z, Yang M, Li L, et al. Ultrasound assessment of diaphragmatic dysfunction as a predictor of weaning outcome from mechanical ventilation: a systematic review and meta-analysis. BMJ Open 2018;8:e021189. [Crossref] [PubMed]
- Vivier E, Muller M, Putegnat JB, et al. Inability of Diaphragm Ultrasound to Predict Extubation Failure: A Multicenter Study. Chest 2019;155:1131-9. [Crossref] [PubMed]
- Demoule A, Molinari N, Jung B, et al. Patterns of diaphragm function in critically ill patients receiving prolonged mechanical ventilation: a prospective longitudinal study. Ann Intensive Care 2016;6:75. [Crossref] [PubMed]
- Jung B, Moury PH, Mahul M, et al. Diaphragmatic dysfunction in patients with ICU-acquired weakness and its impact on extubation failure. Intensive Care Med 2016;42:853-61. [Crossref] [PubMed]
- Vetrugno L, Orso D, Corradi F, et al. Diaphragm ultrasound evaluation during weaning from mechanical ventilation in COVID-19 patients: a pragmatic, cross-section, multicenter study. Respir Res 2022;23:210. [Crossref] [PubMed]
- Dres M, Rozenberg E, Morawiec E, et al. Diaphragm dysfunction, lung aeration loss and weaning-induced pulmonary oedema in difficult-to-wean patients. Ann Intensive Care 2021;11:99. [Crossref] [PubMed]
- Mongodi S, Via G, Bouhemad B, et al. Usefulness of combined bedside lung ultrasound and echocardiography to assess weaning failure from mechanical ventilation: a suggestive case*. Crit Care Med 2013;41:e182-5. [Crossref] [PubMed]
- Haaksma ME, Tuinman PR, Heunks L. Weaning the patient: between protocols and physiology. Curr Opin Crit Care 2021;27:29-36. [Crossref] [PubMed]
- Vignon P, Mücke F, Bellec F, et al. Basic critical care echocardiography: validation of a curriculum dedicated to noncardiologist residents. Crit Care Med 2011;39:636-42. [Crossref] [PubMed]
- Krishnan S, Kuhl T, Ahmed W, et al. Efficacy of an online education program for ultrasound diagnosis of pneumothorax. Anesthesiology 2013;118:715-21. [Crossref] [PubMed]
- Rouby JJ, Arbelot C, Gao Y, et al. Training for Lung Ultrasound Score Measurement in Critically Ill Patients. Am J Respir Crit Care Med 2018;198:398-401. [Crossref] [PubMed]
- Díaz-Gómez JL, Frankel HL, Hernandez A. National Certification in Critical Care Echocardiography: Its Time Has Come. Crit Care Med 2017;45:1801-4. [Crossref] [PubMed]
- Gardelli G, Feletti F, Gamberini E, et al. Using sonography to assess lung recruitment in patients with acute respiratory distress syndrome. Emerg Radiol 2009;16:219-21. [Crossref] [PubMed]
- Corradi F, Brusasco C, Vezzani A, et al. Computer-Aided Quantitative Ultrasonography for Detection of Pulmonary Edema in Mechanically Ventilated Cardiac Surgery Patients. Chest 2016;150:640-51. [Crossref] [PubMed]