Implant-based breast surgery and capsular formation: when, how and why?—a narrative review
Background
Implant-based breast surgery is common, including both reconstructive and cosmetic procedures, with over 300,000 breast implant surgeries performed last year in the United States alone; in 2020, approximately 200,000 procedures performed were cosmetic, and approximately 100,000 were reconstructive (1). Immediately following implantation, breast implants evoke a foreign body reaction (FBR) that ultimately results in a fibrous capsule surrounding the breast implant. Capsule formation is a benign physiologic process mediated by the immune response. Typically, capsule formation does not result in any cosmetic or clinical problems (2). However, excessive capsule fibrosis can occur, resulting in capsule contraction, excessive tightness, physical distortion and chronic pain (3,4). Capsular contracture is the most common complication related to implant-based breast surgery (5). However, the exact etiology and optimal management strategies remain heavily debated.
Although rare, other serious complications can arise from the implant capsule that can affect surgical outcomes, reduce patients’ quality of life and potentially lead to mortality. An increasing body of knowledge on breast implant associated anaplastic large cell lymphoma (BIA-ALCL), as well as squamous cell carcinoma (BIA-SCC), has alarmed plastic surgeons to please the potential of the pathologic sequelae that can result from breast implant-based surgery. These rare pathologies highlight the need for plastic surgeons to have a strong understanding of capsular physiology and factors that can lead to capsule-related pathology.
This manuscript will provide an overview of the literature on the physiology of capsule formation, the pathophysiology of capsular related disease, and an up-to-date review of strategies to prevent and manage capsular complications. We present this article in accordance with the Narrative Review reporting checklist (available at https://atm.amegroups.com/article/view/10.21037/atm-23-131/rc).
Methods
Medline, Embase and PubMed databases were searched from inception to September 2022 (inclusively) to identify relevant articles for inclusion in this review Figure 1. No restrictions were applied at the time of the search (e.g., language, year, status of publication). Keywords and index/subject terms were joined by Boolean operators “AND” or “OR”. Two authors searched the database independently, and a third author resolved any disagreements. The search strategy is summarized in Table 1.
Table 1
Items | Specification |
---|---|
Date of search | 01/09/2022 |
Databases | Medline, Embase and PubMed |
Search terms used | “Capsular Formation”, “Capsular Contracture”, “Implant based breast surgery” |
Time frame | From inception to September 2022 |
Records identified | 7,755 studies |
Records after removing duplicates | 5,819 studies |
Inclusion criteria | • Articles published in English |
• Narrative reviews/literature reviews/systematic reviews and meta-analyses discussing capsular contracture/capsule formation, prospective and retrospective primary studies (including case reports, case series, case-control studies, cohort studies and randomized controlled studies) | |
• Basic science studies (ex vivo and in vivo culture models and animal models) studying the formation of implant capsule/development of capsular contracture as well as any other topics relating to capsule/capsular contracture | |
• Studies focusing on risk factors for the development of capsular contracture, studies focusing on background/epidemiology for the development of capsular contracture and studies focusing on the treatment options available for capsular contracture | |
• Letters to the editor, commentaries and viewpoints were also included to provide new perspectives in the field | |
Exclusion criteria | • Language other than English |
• Abstracts (conference or other) or protocols that were not traced to full text | |
Studies included | 95 studies |
Selection process | Two authors searched the database independently. A third author resolved any disagreements between the other two reviewers |
Physiology of capsule formation
Foreign body reaction and capsule formation
The FBR is the benign physiological reaction of the immune system in response to implanted foreign material that proceeds through several distinct but overlapping phases that mirror wound healing. This process serves as a protective mechanism to ultimately isolate foreign material from the rest of the body with a protective layer of collagenous capsule tissue. The FBR proceeds through the following phases: injury, blood-material interaction, surface provisional matrix formation, acute and chronic inflammation, foreign body giant cell formation and fibrous capsule formation (6-8) (Figure 2).
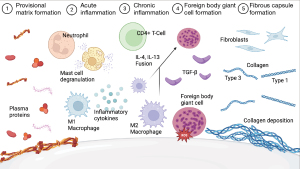
The first stages of the FBR are initiated immediately after implantation. Once inevitable tissue injury occurs, contents of the vascular and lymphatic systems are released and interact with the implant surface. A variety of proteins present in host plasma (e.g., albumin, fibrinogen, complement, fibronectin) spontaneously adsorb on the implant surface, forming a transient provisional matrix. Early protein matrix and implant surface interactions are guided by the Vroman effect, in which mobile low-affinity proteins initially adsorb to the surface but are gradually replaced with less mobile, higher-affinity proteins. The protein matrix becomes rich with chemo-attractants, cytokines and bioactive agents and serves as the physical connection between the implant surface and the immune response, directly guiding further immune cell activity (7).
The inflammatory phases follow (i.e., acute and chronic inflammation). The acute inflammatory phase lasts a few hours to days and is characterized by the infiltration of neutrophils and mast cells (8). Neutrophils serve as first responders to general tissue injury with the role of clearing debris and bacteria and stimulating inflammatory response through the release of cytokines (7). Mast cells degranulate, releasing histamine, serotonin, interleukin-4 (IL-4), IL-13, and IL-8, which cause vasodilation and recruitment of monocytes from the blood stream (8,9). Once within the tissue, monocytes differentiate into macrophages, one of the core cell types responsible for mediating the FBR and marking the transition to the beginning of the chronic inflammatory stages at 2 to 5 weeks post-implantation.
The chronic inflammatory stages are characterized by the presence of macrophages and lymphocytes (8). The main function of macrophages is to phagocytose foreign material, dead cells, and damaged tissues. Macrophages exist on a spectrum of phenotypes and release inflammatory or anti-inflammatory factors depending on their state of differentiation (10). Early in the FBR, pro-inflammatory M1 macrophages (CD68+NOS2+) dominate and boost inflammation by releasing inflammatory cytokines tumor necrosis factor-alpha (TNF-alpha), IL-1, IL-6, IL-8 and degrade biomaterial through phagocytosis and by the release of reactive oxygen species (ROS), degradative enzymes and lysosomes (7,8).
Failure of macrophage phagocytosis degradation of foreign material leads to the chronic inflammatory stage. A hallmark of the FBR to a sufficiently large implant is the fusion of macrophages to terminally differentiated foreign body giant cells (FBGCs). FBGCs are capable of phagocytosing larger particles (>10 µm) compared to macrophages (<5 µm) (7). FBGCs also release further degradative factors and create an acidic environment (11,12). Their presence is pathognomonic for the FBR, and they are associated with the biodegradation of implant material and device failure (13,14). Both IL-4 and IL-13 are associated with macrophage fusion and the transition of pro-inflammatory M1 macrophages to the wound healing M2 phenotype (CD68+CD206+). Wound healing M2 macrophages dampen the inflammatory response through secretion of the anti-inflammatory cytokine IL-10. M2 macrophages are also key in orchestrating tissue remodelling and fibrosis through the production of transforming growth factor-beta (TGF-β), a potent growth factor and master regulator of fibrosis (15). Both M2 macrophages and FBGCs stimulate CD4+ T-cell lymphocytes, key players in mediating chronic inflammation, which contributes to the overall production of a profibrotic milieu to promote fibrous implant encapsulation (16,17).
Following the acute and chronic inflammatory phases, which ideally resolve, fibroblasts become the main cell type responsible for the final stages of the FBR. Early capsule tissue is characteristically granulation tissue, which is composed of immature type 3 collagen, capillaries, macrophages and fibroblasts (8). As the granulation tissue matures, the tissue becomes less cellular and more collagenous and predominated by mature and strong collagen type 1. Ideally, the FBR resolves, and the capsule eventually reaches a steady state where immune activity and fibrosis cease.
The benign capsule is relatively thin, varying between 21 µm to 10 mm in thickness (18,19). Capsules can contain up to three distinct layers: (I) an inner cellular layer, synovial-like metaplasia, with fibroblasts and macrophages (variable presence); (II) a middle vascular layer with loose connective tissue; and (III) a dense outer collagenous layer supplied by an outer vascular supply (20,21). The capsule remains for the lifespan of the device and may alter its phenotype depending on the properties or integrity of the enclosed implant, host or characteristics of the capsule itself.
The development of capsular contracture
In the majority of cases, capsule formation remains benign and does not pose any risk to the patient. However, 10 years following the procedure, in 5–19% of breast augmentation cases and 19–25% of implant-based breast reconstruction cases, the capsule becomes excessively Scarred and fibrotic and manifests clinically as capsular contracture (22-26). Figure 3 demonstrates the thick fibrotic tissue that forms around the implant as a result of this process. Capsular contracture results in symptoms including excessive breast firmness, physical distortion, and pain and can ultimately lead to device failure. To date, two predominant hypotheses have been proposed to explain why capsular contracture occurs.
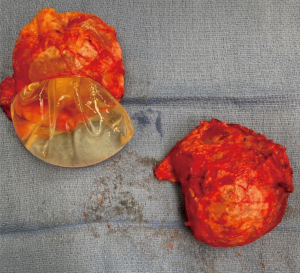
Chronic inflammation in capsular contracture
Here it is supposed that the inflammatory response, which is initiated during the FBR, is either accelerated or prolonged, which ultimately leads to unregulated and continued stimulation of fibrosis (6,27). The exact etiology underlying the prolonged inflammatory response is unknown, however, local events that promote capsular inflammation, such as the presence of hematoma, infection and silicone implant leaking (28-31), have been shown to increase the immune and inflammatory response and risk of capsular contracture (32-34). Histological studies of contracted capsule tissue have demonstrated significantly increased numbers of macrophages and CD4+ T cells (17), a key inflammatory cell implicated in the FBR. Figure 4 demonstrates histological differences between benign and contracted capsule specimens. Molecular analysis has also demonstrated increased expression of inflammatory cytokines IL-1 and IL-6 (17,20,35,36), compared to non-contracted capsule specimens. Other studies have identified significantly increased numbers of fibroblasts (37) and two subpopulations of fibroblasts, including myofibroblasts, which have strong contractile properties (37,38), and CD26+ fibroblasts which produce excess collagen and possess increased profibrotic genes which promote excessive collagen deposition which is another histological hallmark of contracted capsule (38). Ultimately, continued inflammation drives excess stimulation of collagen production, which destroys tissue structure and function and manifests as symptomatic capsular contracture.
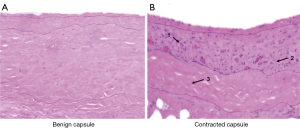
Chronic subclinical infection/biofilm formation in capsular contracture
Another theory relating to the development of capsular contracture involves biofilm formation at the host-implant interface (39,40). Biofilms begin with planktonic bacteria that adhere to a solid surface (i.e., foreign material) (41). Biofilm growth is characterized by bacterial multiplication and extracellular polymeric material synthesis (EPS) (41). In biofilms, the bacteria are sessile. As adhering bacteria divide and produce EPS, they form a microcolony that is highly organized and adherent to the surface and adjacent microcolonies (41). The biofilm results in increased antibiotic resistance by multiple mechanisms, including limiting diffusion of antibiotics through the biofilm matrix, enzyme-mediated resistance, increased metabolic activity, efflux pumps and outer membrane structure (42). Biofilms are often multi-microbial, and under some conditions, a few species may be overrepresented in the biofilm community. Staphylococcus epidermidis is a part of the skin’s microflora and breast’s endogenous flora and has been repeatedly identified in implants from capsule contracture patients (43-45). Propionibacterium acnes, a commensal of the skin and gastrointestinal tract, is a common microbe found on removed breast implants. During surgery, these bacteria may get access to the implants, particularly during peri-nipple-areola or trans-nipple-areola procedures. Streptococci, Bacillus species, Escherichia coli, Mycobacterium species, Corynebacterium, Lactobacilli and other Staphylococcus species (e.g., S. aureus) are also implicated in the production of biofilm on breast implants (43,44,46-49). The presence of biofilm has been suggested to extend the proinflammatory phase, which then results in excess capsular fibrosis (50).
Mitigating risk factors for capsular contracture
The formation of a contracted capsule is a multifactorial process that is incompletely understood. As such, when preparing for breast augmentation or alloplastic breast reconstruction, many factors must be considered to optimize results and reduce complications. Below is a detailed overview of the literature discussing factors that impact the rates of capsular contracture. Figure 5 provides an overview of risk factors for capsular contracture.
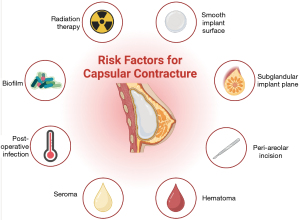
Smooth vs. textured implants
To date, several systematic reviews and meta-analyses have been performed investigating the role of surface texture in the development of capsular contracture. These studies demonstrated that using textured implants significantly reduces capsular contracture, suggesting that textured implants have a protective effect (51-53). Moreover, in 2022, Stevens et al. performed a 5-year prospective trial in patients undergoing breast augmentation to investigate risk factors that predispose patients to developing capsular contracture (54). They found that only 2.6% of patients developed capsular contracture when textured implants were used compared to 6.8% with smooth implants (P<0.0001) (54). A retrospective study of patients undergoing primary breast augmentation demonstrated that smooth implants exhibited a greater rate of capsular contracture at one year postoperatively than textured implants, albeit the difference was statistically insignificant (P=0.06) (55). The surface pores on textured implant shells are hypothesized to induce a multidirectional collagenous fibre orientation and, in so doing, limit the contractile capacity of the fibrous capsule, ultimately reducing the likelihood of developing capsular contracture (56-58). In a study examining capsule samples from 10 patients who had undergone breast reconstruction, there was a substantial increase in the amount of myofibroblasts in the capsule around the smooth implants compared to textured implants (59).
It should be noted that the implant texture can affect the growth of the bacterial biofilm around it. Due to their large and rough surface area, it is thought that macrotextured implants might be associated with an increased risk of bacterial colonization, potentially increasing the risk of subclinical infection. According to the subclinical infection theory, high biofilm load is associated with an increased risk of developing capsular contracture. Therefore, we should expect that macrotextured implants could be associated with a higher risk of capsular contracture. However, textured implants have been associated with lower capsular contracture compared to smooth implants. In light of this, it is important to note that the relationship between bacterial biofilm and capsular contracture is not yet fully understood, and further research is needed to confirm or refute the subclinical infection theory.
Silicone vs. saline implants
The role of silicone vs. saline implants as a risk factor for developing capsular contracture has been highly debated. The predominant underlying foundation of this controversy is that both saline and silicone implants have a silicone outer shell. Therefore, the implant content theoretically should not have an impact. Despite this fact, clinically, it has been suggested that saline implants have lower rates of capsular contracture than their silicone counterparts (60). Several studies have been conducted assessing the role of implant fill material on the development of capsular contracture; however, there is little reliable data available (61). Currently, the role of implant content as a risk factor for capsular contracture remains unclear. One proposed hypothesis as to why saline implants may have lower rates of capsular contracture is because they are inserted prior to filling, thereby reducing the surface area which is in contact with the skin and parenchyma.
Insertion plane
In breast augmentation, an implant can be inserted in a subglandular, submuscular (subpectoral) or subfascial plane. Submuscular placement of implants confers a lower risk of developing capsular contracture compared to subglandular implants. This is supported by meta-analysis data reported by Li et al. 2019 (62) which aggregated data from eleven trials that evaluated and compared capsular contracture rates between submuscular and subglandular groups and found higher rates of capsular contracture in the subglandular group (9.6% subglandular vs. 1.7% submuscular, P<0.00001). However, significant heterogeneity was present (I2=91%) (62). Although the constant rubbing of the pectoralis muscles over the implant is a potential inflammatory stimulus, submuscular placement is believed to cause fewer cases of capsular contracture, given that the pectoralis provides a vascularized tissue layer that can protect the implant from endogenous bacterial invasion found in the glandular tissues that may lead to a chronic inflammatory response that promotes capsular contracture (63,64).
Incision location
Incision location is another factor which has been demonstrated to impact capsular contracture rates in breast augmentation. The rates of capsular contracture associated with periareolar, inframammary and transaxillary incisions in breast augmentation patients were compared via meta-analysis (65). Statistically higher rates of capsular contracture were seen in periareolar incisions as compared to inframammary and transaxillary incisions combined (7.2% periareolar vs. 3.1% non-periareolar, P=0.03) as well as compared to inframammary incisions alone (P=0.03) (65,66). While the underlying mechanism of increased capsular contracture rates in peri-areolar incisions has not been proven, the theory underlying this clinical observation is linked to the endogenous bacteria that might be released from the breast glandular tissue as the breast implant pocket is developed (67). Of note, a study by Newman et al. compared the effect of the Keller funnel on the rate of capsular contracture in periareolar breast augmentation (68). The capsular contracture rate was 1.3% among cases where a Keller funnel was used vs. 10% when it was not (68); this has been suggested to be associated with the fact that given the no-touch technique, there is less bacterial contamination (68).
The no-touch technique was first described as a retraction technique to prevent the implant from touching the skin around the incision to limit bacterial contamination (69). Of the different techniques utilized, the most popular technique involves the use of the Keller funnel; a nylon sleeve made of transparent polymeric material made with a hydrophilic coating that allows implants to slide into a pocket (69). Inserting the funnel into the incision limits exposure to surrounding tissues and to the surgeon’s gloves (69). As mentioned above, reductions in capsular contraction rates have been reported when a funnel is used (70). Of note, a study by Besnick et al. in 2021 showed a higher prevalence of capsular contracture on the second-side funnels when used in women undergoing breast augmentation compared to the first side, further reinforcing the benefit of using a pristine funnel to insert breast implants (second side: 3.33% vs. first-side 1.17%, P=0.0179) (71).
Previous treatment with radiation therapy to the breasts
Post mastectomy radiation therapy is an important adjunct for the treatment of breast cancer (72). Unfortunately, post mastectomy radiation therapy also increases the risk of capsular contracture in implant-based breast reconstruction (IBBR). A meta-analysis reinforced the deleterious effects of post mastectomy radiation therapy on IBBR, showing capsular contracture rates of up to 50% in patients receiving post mastectomy radiation therapy (73). This is further cemented by the largest prospective study in the literature conducted by Cordeiro et al., which showed significantly higher capsular contracture rates in irradiated implants than in non-irradiated implants (6.9% vs. 0.5%, P<0.001) (74). The cause of this increased risk is thought to be due to the inflammation caused by radiation as evidenced by higher concentrations of fibroblasts and macrophages in irradiated capsules (75,76). Additionally, the process of collagen deposition is also disrupted, leading to an irregular pattern in irradiated capsules (77).
Undeniably, we can conclude that post mastectomy radiation therapy can impact IBBR negatively. However, it is essential to note how prepectoral implant insertion can help alleviate some of these adverse effects. In subpectoral implants, radiation can lead to muscle scarring and fibrosis, which leads to muscle shortening. As the muscle tightens, the implant will be elevated, causing the entire pocket to contract. On the other hand, in prepectoral reconstruction, the implant is unaffected by any muscle scarring or shortening (78). The impact of post mastectomy radiation therapy on prepectoral implants was reported in a recent study by Sigalove et al., where they observed a rate of 0% of clinically significant capsular contracture (78).
Perioperative factors
Complications occurring soon after breast implant surgery can lead to early (<6 months after surgery) capsular contracture. These complications include implant rupture, hematoma, bleeding in the pocket and surgical trauma (79). For example, in a prospective study conducted by Handel et al. in a cohort of 752 patients, capsular contracture occurred more commonly among those with a hematoma (29% capsular contracture in the hematoma group vs. 13% capsular contracture no hematoma group, P<0.01) (61). Similar results were seen by Codner et al. (80). Capsular contracture occurred at a rate of 25% among those with a hematoma vs. only in 8% that did not have a hematoma (80).
As discussed before, bacterial contamination of breast implants leads to the formation of a biofilm (i.e., late factors; >6 months after surgery) around the implant, which has been found to be a significant contributor to the development of capsular contracture. The growing body of evidence on the development of bacterial biofilm around breast implants and its association with capsular contracture and other complications has led to the creation of clinical recommendations to mitigate the risk of developing bacterial biofilm. The 14-point plan proposed by Deva et al. to help reduce the risk of bacterial contamination is summarized in Table 2 (81-83).
Table 2
No. | Recommendation |
---|---|
1 | The use of IV antibiotics at the time of induction especially antibiotics targeting S. epidermidis |
2 | Avoiding Periareolar incisions and using inframammary incisions when possible as discussed before |
3 | The use of nipple shield to prevent bacterial migration into the breast pocket (82) |
4 | Minimizing traumatic dissection and devascularized tissues |
5 | Careful hemostasis |
6 | Avoid dissection of breast parenchyma |
7 | The use of submuscular plane as mentioned before |
8 | Irrigating the entire breast pocket using triple antibiotic solution or povidone-iodine solution |
9 | Minimizing the skin to implant contact “no-touch” technique (83) |
10 | Minimizing the time between implant opening and insertion |
11 | Changing surgical gloves prior to handling the implant and clean all instruments with antibiotic solution |
12 | Avoid draining tube for breast augmentation, and use proper technique if drain is necessary |
13 | Layered closure |
14 | Antibiotic prophylaxis for IBBR patients undergoing breast procedure such as tattoos and piercings |
IBBR, implant-based breast reconstruction.
Management of capsular contracture
The decision to treat capsular contracture depends on various factors, but chief among them are the presenting symptoms and their effect on the patient’s quality of life. For instance, patients with severe capsular contracture could only present with no symptoms at all or mild discomfort that doesn’t bother the patient. The patient might not want to undergo surgery to treat capsular contracture in these situations. Classifications such as Baker and Baker-Spear exist to classify and grade the severity of capsular contracture, however, they have been criticized due to a lack of validation studies and their subjective nature. A study by de Bakker et al. (84) assessed interobserver reliability and observer agreement of the Baker classification in women undergoing breast augmentation. Specifically, the interobserver reliability of the Baker classification was poor [Kappa 0.55; 95% confidence interval (CI): 0.37 to 0.72], and the interobserver agreement was 48%, highlighting that the Baker Classification is an unreliable diagnostic tool. Therefore, when it comes to surgical planning, these classifications should be approached as adjuncts to clinical assessment, and surgeons should rely on their clinical experience and the patient’s preferences in each case.
The current approach in managing capsular contracture includes capsulectomy, implant exchange, and plane change. Although this approach is considered the current gold standard in managing capsular contracture, more evidence is needed to support one approach’s superiority over another (85).
Capsulectomy vs. capsulotomy
Capsulectomy usually involves more dissection and operating time compared to capsulotomies. It is associated with more complications such as bleeding and pneumothorax when compared to capsulotomy (86). Capsulotomy involves incision and release of a tethered capsule. Although it is associated with less morbidity, some have regarded it as inadequate and could lead to recurrent capsular contracture (86). However, this fact has been argued by several researchers who have been investigating capsulectomy vs. open capsulotomy.
Studies have reported variable recurrence rates ranging from 0–53% for capsulectomy and 0–54% for open capsulotomy. In a recent systematic review by Wan and Rohrich, they found inadequate evidence to support the effectivity of capsulectomy over open capsulotomy in treating capsular contracture (85). Therefore, the effectiveness of capsulotomy alone should not be overlooked in the management of capsular contracture. In the same review, they also reported weak evidence to support the superiority of total over partial capsulectomy. Therefore, they suggest that the choice of the extent of the capsulectomy should be tailored to the patient and the clinical judgement of the treating team.
Implant exchange
Implant exchange is an important aspect of the current management approach for capsular contracture. Wan and Rohrich report in their recent systematic review that implant exchange is associated with lower (0–26%) recurrence rates of capsular contracture compared with no implant exchange (0–54%) (85). There are several potential reasons why implant exchange is associated with lower recurrence rates. Firstly, the old implants might harbor subclinical amounts of bacteria that might induce local inflammation and subsequent fibrosis leading to capsular contracture. Another reason is that implant exchange allows the surgeon to replace old implants, which may have subclinical bacterial colonization, with a newer one that may be less prone to developing capsular contracture (85). Although implant exchange is crucial for managing capsular contracture, in some cases, implant exchange is not feasible due to patient preference or financial reasons. In these cases, replacing the old implants in a new pocket is advised rather than replacing them in the old pocket. This is supported by several studies reporting a high recurrence rate of capsular contracture when the implant was replaced in the same pocket vs. a new pocket (54% vs. 26%) (87-89).
Plane change
Changing the plane of implant insertion can help reduce the recurrence of capsular contracture (85). Although there is no evidence to support one technique over another (85), conversion from subglandular plane to submuscular or dual plane is the most favoured (4,90-92). As mentioned before, the submuscular plane provides a vascularized muscle layer that can protect the implant from endogenous bacteria from the breast tissue, explaining the reduced incidence of recurrence.
Acellular dermal matrix (ADM)
ADM is a tissue matrix that was originally developed to expand the soft tissue envelope after mastectomy (3), but emerging data suggest that placement of ADM can be a powerful adjunct in preventing recurrent capsular contracture. Placing ADM in the breast pocket following capsulotomy or capsulectomy is thought to provide additional support to the breast implant. The ADM may also integrate within the capsule, and the fibrous dermal matrix may interrupt the circumferential contractile activity of the capsule around the implant, thereby reducing symptomatic contracture. Moreover, it can act as an insulating layer around the implant that prevents the migration of inflammatory cells and endogenous bacteria from surrounding tissues, thus preventing host immune response, local inflammation and capsular contracture.
Notably, a recent study by Hidalgo et al. reports a 96.9% success rate while using ADM to treat capsular contracture compared to a 72.5% success rate when it was not used (93). Based on their findings, they proposed a new treatment algorithm for treating grade III/IV capsular contracture. This algorithm suggests that all bilateral capsular contracture cases should be treated with ADM. While unilateral cases can be treated using the previously mentioned conventional way. If the unilateral cases present with a second recurrence, ADM should be strongly considered.
Alternative treatments
Several medications and treatments aimed at reducing peri-prosthetic inflammation have been investigated to reduce the development of capsular contracture. Although some of these treatments have shown promising results, most of these medications have not yet been investigated extensively enough to provide definitive evidence.
Leukotriene antagonists: specifically, montelukast and zafirlukast have been utilized in the pharmacologic prevention and treatment of capsular contracture (94,95). This is explained by their effect on inhibiting inflammatory leukotrienes C4, D4, and E4 that could be linked to capsular contracture (94). Possible side effects of these medications include headache, flu-like symptoms, abdominal pain, and dyspepsia (94). Although liver failure and hepatitis are rare complications, it is advised to monitor transaminase levels (94,96). While currently available studies indicate that leukotriene antagonists are effective at reversing and preventing capsular contracture, further studies are necessary to assess clinical efficacy, duration, safety and their mechanism in periprosthetic capsular contracture (94).
Cromolyn sodium is known to stabilize mast cells and thus prevent the release of inflammatory mediators such as histamine and leukotrienes (97). Recently, it was tested as a means of prophylaxis for capsular contracture in Wistar albino rats (98). Compared to control and sham groups, the cromolyn sodium group had less acute inflammation, lower mean inflammatory severity scores and foreign body reaction occurrence, mast cell counts and capsular thickness (98). When compared to montelukast and zafirlukast, outcomes were better in the cromolyn sodium group (98). These results suggest a potential role for cromolyn sodium as a prophylactic agent to reduce capsular contracture (98).
Pirfenidone: a new antifibrotic drug approved in 2014 by the FDA for treating pulmonary fibrosis and subsequently for hepatic cirrhosis, peritoneal sclerosis and hypertrophic scars (99). Interestingly, this drug has shown promising results in preventing capsular contracture in rat models (100). This was supported by a recent clinical trial by the same authors that showed a reduction of capsular contracture in all the patients enrolled in their study (n=17) (101). Moreover, they report only minor side effects, such as nausea and vomiting.
Steroids: They are known for their anti-inflammatory effects, which could reduce the inflammatory stimulus for capsular contracture. Therefore, in 1994 Caffee et al. attempted using an intracapsular injection of triamcinolone for patients unable to undergo capsulectomy or patients who underwent multiple capsulotomies (102). They observed the resolution of capsular contracture in 70% of the cases. A similar study was conducted in 2011 by Schonfienza et al., where they injected triamcinolone under ultrasound guidance. In their study, they report a reduction in the capsule thickness and a reduction in pain. Although steroids have potentially promising results, no other researchers attempted to exclusively study their effect on the management of capsular contracture to our knowledge.
Breast implant capsule associated pathologies
Since the discovery of BIA-ALCL, there has been an increased interest in investigating breast implant associated pathologies. In the literature, there have been several reports on benign and malignant pathologies associated with the breast implant capsule. However, in this review, we discuss the most commonly reported pathologies to this date.
BIA-ALCL
BIA-ALCL is a T-cell lymphoma of the breast implant capsule affecting women 7–10 years post implant-based surgery (103,104). In all cases, patients who developed BIA-ALCL have had a history of a textured breast implant or tissue expander. BIA-ALCL is rare, with the highest reported incidences coming from two separate studies from Memorial Sloan Kettering Cancer Center reporting rates of 1:355–1:559 (105,106). Although the exact pathophysiology is not yet fully understood, the main hypothesis links the macrotextured implants with chronic inflammation, which could be induced by bacterial biofilm, silicone particulate wear or tribology, or genetic predisposition. This hypothesis can be explained by recent studies reporting the association of textured implants with higher biofilm load (107). The high bacterial load could result in chronic inflammation that leads to T-cell activation and possible malignancies. Moreover, the “bacteria theory” posits that gram negative bacteria colonizing the implant can release endotoxins that can precipitate to BIA-ALCL (107). Originally, they reported the association of BIA-ALCL with specific bacteria such as Ralstonia. However, other studies argued the causality of BIA-ALCL and these specific bacterial species (108). Another theory is the “tribology theory”, which postulates that textured implants exert stress of >100 pa on the surrounding capsule leading to cell apoptosis and chronic inflammation, which result in activation and proliferation of T-lymphocytes leading to monoclonal mutations (109,110).
Clinically, patients affected by BIA-ALCL usually present with sudden onset of unilateral swelling with a mean time to onset of 7–10 years post implant. Any suspected case should be investigated per the National Comprehensive Cancer Network (NCCN) guidelines. Firstly, the patient should undergo breast imaging using ultrasound. If any fluid collection or mass is detected, it should be aspirated or biopsied respectively. If the results are inconclusive, MRI is warranted to help in diagnosis. The excised mass or the aspirated fluid will be sent for cytology, immunohistochemistry for CD30 and ALK, along with flow cytometry for T-cell markers. Figure 6 shows the histology of the capsule from a patient with BIA-ALCL compared to the patients’ opposite benign breast capsule. Other additional differential markers include CD2, CD3, CD4, CD5, CD7, CD8, and CD45. If the results are equivocal or negative, the sample must be sent for another pathologic analysis at a tertiary cancer center. If negative, the fluid collection should be treated as a seroma. However, if the results came back positive, the patient should be referred to a multi-disciplinary oncologic team to undergo complete capsule, implant and lymphoma excision, and adjuvant therapy where indicated. In some cases, capsulectomy of the contralateral side is warranted. Moreover, the patient should be followed by hematology for possible adjuvant chemotherapy. Studies have found no clear role in radical mastectomy, sentinel lymph node biopsy or axillary lymph node dissection.
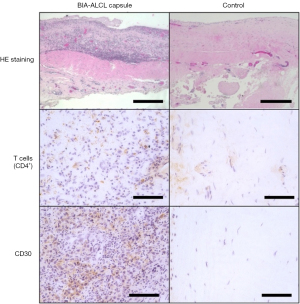
BIA-SCC
To date, 16 cases of BIA-SCC have been reported as per the latest ASPS statement on BIA-SCC (111-117). Although the exact etiology is unknown, the cause of BIA-SCC is thought to be the result of chronic inflammation or irritation of a capsule that has undergone epithelization or squamous metaplasia in a manner similar to a Marjolin’s ulcer. Even though the origin of the epithelial cells is not definitively determined, they may come from capsular cell transformation, metaplasia of the breast ductal cells, or seeded from surgical incisions.
Patients presenting with BIA-SCC have an average of 52 years old and an average implantation time of more than 15 years (113,114,116). Based on the current case reports in the literature, the majority of BIA-SCC patients have been associated with silicone implants except for two cases that had a saline and textured saline implant (115). The majority of these cases had no history of breast cancer. At the time of presentation, the patients presented with painful swelling/mass, pain, and erythema with possible axillary lymphadenopathy.
Although BIA-SCC is a rare malignancy, the overwhelming and devastating outcomes dictate the importance of early diagnosis and management. Following the NCCN BIA-ALCL guidelines (118), all patients presenting with unilateral painful breast swelling, capsular mass or delayed (7–10 years) periprosthetic fluid collection should be evaluated and worked up. Similar to BIA-ALCL, all patients should undergo breast ultrasound and MRI with and without contrast (119). Subsequently, the aspirated fluid or capsular mass should be sent for cytology, immunohistochemistry for CD5, CD6, and p63 and flow cytometry for squamous cells and keratin (119). When examined, the mass shows dysplastic keratinized epithelium of the capsule, and the fluid aspirates are usually rich in keratin (111,114). Once the disease is confirmed, all patients should undergo a PET scan prior to surgical management due to the high risk (80%) of regional metastasis.
Management of BIA-SCC patients should be discussed in a multidisciplinary tumor board to plan the best course of action for the patients. Per the latest ASPS statement, BIA-SCC patients should at least undergo explantation and complete capsulectomy. Based on existing data from case reports, incomplete resection of the cancer could result in aggressive recurrence. Subsequently, similar to patients who develop breast squamous cell carcinoma without implants, BIA-SCC patients should be referred to medical oncology and radiation oncology services for consideration of adjuvant chemotherapy and radiotherapy, respectively.
Breast implant illness (BII)
BII is defined as a variety of symptoms that patients report that they attribute to their implants. Over 100 symptoms (local and systemic) have been associated with BII. Yet, to date, no specific symptoms, diagnostic criteria, abnormal physical exam, or laboratory findings have been associated with BII. Two mechanisms by which BII has been postulated to occur are via the presence of subclinical infections/biofilms and the presence of heavy metals within the implants. In a prospective blinded study by McGuire et al. in 2022, they investigated microbes, histology, blood analysis, enterotoxin, and cytokines in patients complaining of BII relative to controls (120). There was no statistical difference in blood analysis (TSH, vitamin D levels, CBC) or next-generation microbiome sequencing from implant and capsule swabs. To assess the role of a Staphylococcal infection in causing a systemic inflammatory response, serum was assessed for IgE and IgG anti-SEA, anti-SEB and anti-TSST. Higher levels of IgG anti-SEA were present in patients with BII symptoms requesting removal of their implant. Otherwise, there was no significant differences (120). They observed no significant histologic differences except for a greater number of capsules with synovial metaplasia in the non-BII cohort (120). However, they identified significantly higher levels of IL-17A, IL-13 and IL-22 in patients with BII symptoms requesting implant removal compared to groups requesting removal/exchange with no symptoms and patients undergoing mastopexy who never had an implant (120). Given that the differences were of low clinical value and the many non-significant differences between groups, they concluded that their study further supported the existing literature that there are few biomedical factors which explain the BII symptoms (120).
With regards to heavy metals, Wixtrom et al. 2022 compared patients who had BII symptoms vs. those who had implants and did not (121). Twenty-two metals were tested relative to patients with implants and no BII symptoms; the cohort with BII symptoms had statistically higher levels of arsenic and zinc and lower levels of cobalt, manganese, silver, and tin (121). The higher levels of zinc and arsenic were attributed to smoking, gluten-free diets, dietary supplements, and the presence of tattoos. Of note, all measured levels of the metals were below the acceptable exposure level set by regulatory agencies (121). More interesting was that heavy metals were detected in the non-implant group breast tissue, with certain metals presenting higher levels than in the implant groups (121). Altogether, the data suggest that the indications for total capsulectomy should not include the risk of heavy metal toxicity (121).
Many patients believe that they require an en bloc capsulectomy for BII to resolve, however, this procedure does not come without risks. From the same cohort, Glicksman et al. 2022 demonstrated that patients who self-reported BII had a statistically significant improvement in their symptoms when they received an explantation, but the type of capsulectomy (intact total, total or partial) performed did not have an impact on BII symptoms (122). It follows from this study that while patients may experience some relief of their symptoms following surgery, it appears to have more to do with implant removal rather than the capsule. Another interesting finding from this study is that patients with self-reported BII had elevated levels of anxiety, which was decreased and maintained six months post-explantation, suggesting that anxiety can be a possible etiological factor for BII (122). These findings suggest that mental health may play an important role in this self-reported condition.
Conclusions
Capsular formation is a benign physiologic response to implant-based breast surgery that can evolve into capsular contracture or other serious complications that can affect the patient’s life. Understanding the process of capsular formation and the pathophysiology of its complications is crucial in improving surgical outcomes and providing possible ways, whether for prevention or treatment. This could also provide plastic surgeons with evidence-based data for surgical planning and patient education. Although researchers and plastic surgeons have been working hand in hand to provide cutting-edge research on this topic, various pieces are still missing to understand the scope of capsular formation and its complications fully. Therefore, extensive research is encouraged on this topic, focusing mainly on the pathophysiology of the disease and its management and how to prevent serious complications such as BIA-ALCL and SCC.
Acknowledgments
Funding: None.
Footnote
Provenance and Peer Review: This article was commissioned by the Editorial Office, Annals of Translational Medicine for the series “The Modern Plastic and Reconstructive Surgeon – Collaborator, Innovator, Leader”. The article has undergone external peer review.
Reporting Checklist: The authors have completed the Narrative Review Reporting checklist. Available at https://atm.amegroups.com/article/view/10.21037/atm-23-131/rc
Conflicts of Interest: All authors have completed the ICMJE uniform disclosure form (available at https://atm.amegroups.com/article/view/10.21037/atm-23-131/coif). The series “The Modern Plastic and Reconstructive Surgeon – Collaborator, Innovator, Leader” was commissioned by the editorial office without any funding or sponsorship. JIE served as the unpaid Guest Editor of the special series. The authors have no other conflicts of interest to declare.
Ethical Statement: The authors are accountable for all aspects of the work in ensuring that questions related to the accuracy or integrity of any part of the work are appropriately investigated and resolved.
Open Access Statement: This is an Open Access article distributed in accordance with the Creative Commons Attribution-NonCommercial-NoDerivs 4.0 International License (CC BY-NC-ND 4.0), which permits the non-commercial replication and distribution of the article with the strict proviso that no changes or edits are made and the original work is properly cited (including links to both the formal publication through the relevant DOI and the license). See: https://creativecommons.org/licenses/by-nc-nd/4.0/.
References
- ASPS 2020 Plastic Surgery Report. Available online: https://www.plasticsurgery.org/documents/News/Statistics/2020/plastic-surgery-statistics-full-report-2020.pdf. 2020.
- Bachour Y. Capsular Contracture in Breast Implant Surgery: Where Are We Now and Where Are We Going? Aesthetic Plast Surg 2021;45:1328-37. [Crossref] [PubMed]
- Safran T, Nepon H, Chu CK, et al. Current Concepts in Capsular Contracture: Pathophysiology, Prevention, and Management. Semin Plast Surg 2021;35:189-97. [Crossref] [PubMed]
- Adams WP Jr. Capsular contracture: what is it? What causes it? How can it be prevented and managed? Clin Plast Surg 2009;36:119-26. vii. [Crossref] [PubMed]
- Chopra K, Gowda A, Holton LH, et al. Complications After Primary Breast Augmentation: Capsular Contracture. Eplasty 2015;15:ic40.
- Bayston R. Capsule formation around breast implants. JPRAS Open 2022;31:123-8. [Crossref] [PubMed]
- Anderson JM, Rodriguez A, Chang DT. Foreign body reaction to biomaterials. Semin Immunol 2008;20:86-100. [Crossref] [PubMed]
- Klopfleisch R, Jung F. The pathology of the foreign body reaction against biomaterials. J Biomed Mater Res A 2017;105:927-40. [Crossref] [PubMed]
- Zdolsek J, Eaton JW, Tang L. Histamine release and fibrinogen adsorption mediate acute inflammatory responses to biomaterial implants in humans. J Transl Med 2007;5:31. [Crossref] [PubMed]
- Henson PM. The immunologic release of constituents from neutrophil leukocytes. I. The role of antibody and complement on nonphagocytosable surfaces or phagocytosable particles. J Immunol 1971;107:1535-46.
- Miron RJ, Bosshardt DD. Multinucleated Giant Cells: Good Guys or Bad Guys? Tissue Eng Part B Rev 2018;24:53-65. [Crossref] [PubMed]
- Milde R, Ritter J, Tennent GA, et al. Multinucleated Giant Cells Are Specialized for Complement-Mediated Phagocytosis and Large Target Destruction. Cell Rep 2015;13:1937-48. [Crossref] [PubMed]
- Anderson JM, McNally AK. Biocompatibility of implants: lymphocyte/macrophage interactions. Semin Immunopathol 2011;33:221-33. [Crossref] [PubMed]
- Sheng N, Fairbanks MB, Heinrikson RL, et al. Cleaved high molecular weight kininogen binds directly to the integrin CD11b/CD18 (Mac-1) and blocks adhesion to fibrinogen and ICAM-1. Blood 2000;95:3788-95.
- Kim HS, Kim S, Shin BH, et al. Silicone Implants Immobilized with Interleukin-4 Promote the M2 Polarization of Macrophages and Inhibit the Formation of Fibrous Capsules. Polymers (Basel) 2021;13:2630. [Crossref] [PubMed]
- Cappellano G, Ploner C, Lobenwein S, et al. Immunophenotypic characterization of human T cells after in vitro exposure to different silicone breast implant surfaces. PLoS One 2018;13:e0192108. [Crossref] [PubMed]
- Wolfram D, Rabensteiner E, Grundtman C, et al. T regulatory cells and TH17 cells in peri-silicone implant capsular fibrosis. Plast Reconstr Surg 2012;129:327e-37e. [Crossref] [PubMed]
- Bui JM, Perry T, Ren CD, et al. Histological characterization of human breast implant capsules. Aesthetic Plast Surg 2015;39:306-15. [Crossref] [PubMed]
- Prantl L, Schreml S, Fichtner-Feigl S, et al. Clinical and morphological conditions in capsular contracture formed around silicone breast implants. Plast Reconstr Surg 2007;120:275-84. [Crossref] [PubMed]
- Wolfram D, Rainer C, Niederegger H, et al. Cellular and molecular composition of fibrous capsules formed around silicone breast implants with special focus on local immune reactions. J Autoimmun 2004;23:81-91. [Crossref] [PubMed]
- de Bakker E, van den Broek LJ, Ritt MJPF, et al. The Histological Composition of Capsular Contracture Focussed on the Inner Layer of the Capsule: An Intra-Donor Baker-I Versus Baker-IV Comparison. Aesthetic Plast Surg 2018;42:1485-91. [Crossref] [PubMed]
- Bengtson BP, Van Natta BW, Murphy DK, et al. Style 410 highly cohesive silicone breast implant core study results at 3 years. Plast Reconstr Surg 2007;120:40S-8S. [Crossref] [PubMed]
- Spear SL, Murphy DK, Slicton A, et al. Inamed silicone breast implant core study results at 6 years. Plast Reconstr Surg 2007;120:8S-16S. [Crossref] [PubMed]
- Spear SL, Murphy DK, Allergan Silicone Breast Implant US.. Natrelle round silicone breast implants: Core Study results at 10 years. Plast Reconstr Surg 2014;133:1354-61. [Crossref] [PubMed]
- Hölmich LR, Breiting VB, Fryzek JP, et al. Long-term cosmetic outcome after breast implantation. Ann Plast Surg 2007;59:597-604. [Crossref] [PubMed]
- Marques M, Brown SA, Oliveira I, et al. Long-term follow-up of breast capsule contracture rates in cosmetic and reconstructive cases. Plast Reconstr Surg 2010;126:769-78. [Crossref] [PubMed]
- Lee SG, Lee SD, Kim MK, et al. Effect of Antiadhesion Barrier Solution and Fibrin on Capsular Formation After Silicone Implant Insertion in a White Rat Model. Aesthetic Plast Surg 2015;39:162-70. [Crossref] [PubMed]
- Shin BH, Kim BH, Kim S, et al. Silicone breast implant modification review: overcoming capsular contracture. Biomater Res 2018;22:37. [Crossref] [PubMed]
- Bachour Y, Poort L, Verweij SP, et al. PCR Characterization of Microbiota on Contracted and Non-Contracted Breast Capsules. Aesthetic Plast Surg 2019;43:918-26. [Crossref] [PubMed]
- Hallab NJ, Samelko L, Hammond D. The Inflammatory Effects of Breast Implant Particulate Shedding: Comparison With Orthopedic Implants. Aesthet Surg J 2019;39:S36-48. [Crossref] [PubMed]
- Walker JN, Pinkner CL, Pinkner JS, et al. The Detection of Bacteria and Matrix Proteins on Clinically Benign and Pathologic Implants. Plast Reconstr Surg Glob Open 2019;7:e2037. [Crossref] [PubMed]
- Moyer HR, Ghazi BH, Losken A. The effect of silicone gel bleed on capsular contracture: a generational study. Plast Reconstr Surg 2012;130:793-800. [Crossref] [PubMed]
- Kim L, Castel N, Parsa FD. Case of late hematoma after breast augmentation. Arch Plast Surg 2018;45:177-9. [Crossref] [PubMed]
- Gallo A, Solfrizzo M, Epifani F, et al. Effect of temperature and water activity on gene expression and aflatoxin biosynthesis in Aspergillus flavus on almond medium. Int J Food Microbiol 2016;217:162-9. [Crossref] [PubMed]
- Chung L, Maestas DR Jr, Lebid A, et al. Interleukin 17 and senescent cells regulate the foreign body response to synthetic material implants in mice and humans. Sci Transl Med 2020;12:eaax3799. [Crossref] [PubMed]
- Siggelkow W, Faridi A, Spiritus K, et al. Histological analysis of silicone breast implant capsules and correlation with capsular contracture. Biomaterials 2003;24:1101-9. [Crossref] [PubMed]
- Coleman DJ, Sharpe DT, Naylor IL, et al. The role of the contractile fibroblast in the capsules around tissue expanders and implants. Br J Plast Surg 1993;46:547-56. [Crossref] [PubMed]
- Schlesinger SL, Ellenbogen R, Desvigne MN, et al. Zafirlukast (Accolate): A new treatment for capsular contracture. Aesthet Surg J 2002;22:329-36. [Crossref] [PubMed]
- Donlan RM. Biofilms: microbial life on surfaces. Emerg Infect Dis 2002;8:881-90. [Crossref] [PubMed]
- Costerton JW, Stewart PS. Battling biofilms. Sci Am 2001;285:74-81. [Crossref] [PubMed]
- Srinivasan R, Santhakumari S, Poonguzhali P, et al. Bacterial Biofilm Inhibition: A Focused Review on Recent Therapeutic Strategies for Combating the Biofilm Mediated Infections. Front Microbiol 2021;12:676458. [Crossref] [PubMed]
- Singh S, Singh SK, Chowdhury I, et al. Understanding the Mechanism of Bacterial Biofilms Resistance to Antimicrobial Agents. Open Microbiol J 2017;11:53-62. [Crossref] [PubMed]
- Pajkos A, Deva AK, Vickery K, et al. Detection of subclinical infection in significant breast implant capsules. Plast Reconstr Surg 2003;111:1605-11. [Crossref] [PubMed]
- Schreml S, Heine N, Eisenmann-Klein M, et al. Bacterial colonization is of major relevance for high-grade capsular contracture after augmentation mammaplasty. Ann Plast Surg 2007;59:126-30. [Crossref] [PubMed]
- Ahn CY, Ko CY, Wagar EA, et al. Microbial evaluation: 139 implants removed from symptomatic patients. Plast Reconstr Surg 1996;98:1225-9. [Crossref] [PubMed]
- Washer LL, Gutowski K. Breast implant infections. Infect Dis Clin North Am 2012;26:111-25. [Crossref] [PubMed]
- Macadam SA, Mehling BM, Fanning A, et al. Nontuberculous mycobacterial breast implant infections. Plast Reconstr Surg 2007;119:337-44. [Crossref] [PubMed]
- Vinh DC, Rendina A, Turner R, et al. Breast implant infection with Mycobacterium fortuitum group: report of case and review. J Infect 2006;52:e63-7. [Crossref] [PubMed]
- Del Pozo JL, Tran NV, Petty PM, et al. Pilot study of association of bacteria on breast implants with capsular contracture. J Clin Microbiol 2009;47:1333-7. [Crossref] [PubMed]
- Tamboto H, Vickery K, Deva AK. Subclinical (biofilm) infection causes capsular contracture in a porcine model following augmentation mammaplasty. Plast Reconstr Surg 2010;126:835-42. [Crossref] [PubMed]
- Barnsley GP, Sigurdson LJ, Barnsley SE. Textured surface breast implants in the prevention of capsular contracture among breast augmentation patients: a meta-analysis of randomized controlled trials. Plast Reconstr Surg 2006;117:2182-90. [Crossref] [PubMed]
- Wong CH, Samuel M, Tan BK, et al. Capsular contracture in subglandular breast augmentation with textured versus smooth breast implants: a systematic review. Plast Reconstr Surg 2006;118:1224-36. [Crossref] [PubMed]
- Larsen A, Rasmussen LE, Rasmussen LF, et al. Histological Analyses of Capsular Contracture and Associated Risk Factors: A Systematic Review. Aesthetic Plast Surg 2021;45:2714-28. [Crossref] [PubMed]
- Stevens WG, Nahabedian MY, Calobrace MB, et al. Risk factor analysis for capsular contracture: a 5-year Sientra study analysis using round, smooth, and textured implants for breast augmentation. Plast Reconstr Surg 2013;132:1115-23. [Crossref] [PubMed]
- Filiciani S, Siemienczuk GF, Etcheverry MG. Smooth versus Textured Implants and Their Association with the Frequency of Capsular Contracture in Primary Breast Augmentation. Plast Reconstr Surg 2022;149:373-82. [Crossref] [PubMed]
- Rubino C, Mazzarello V, Farace F, et al. Ultrastructural anatomy of contracted capsules around textured implants in augmented breasts. Ann Plast Surg 2001;46:95-102. [Crossref] [PubMed]
- Abramo AC, De Oliveira VR, Ledo-Silva MC, et al. How texture-inducing contraction vectors affect the fibrous capsule shrinkage around breasts implants? Aesthetic Plast Surg 2010;34:555-60. [Crossref] [PubMed]
- Wickman M, Johansson O, Olenius M, et al. A comparison of the capsules around smooth and textured silicone prostheses used for breast reconstruction. A light and electron microscopic study. Scand J Plast Reconstr Surg Hand Surg 1993;27:15-22. [Crossref] [PubMed]
- Kuriyama E, Ochiai H, Inoue Y, et al. Characterization of the Capsule Surrounding Smooth and Textured Tissue Expanders and Correlation with Contracture. Plast Reconstr Surg Glob Open 2017;5:e1403. [Crossref] [PubMed]
- Headon H, Kasem A, Mokbel K. Capsular Contracture after Breast Augmentation: An Update for Clinical Practice. Arch Plast Surg 2015;42:532-43. [Crossref] [PubMed]
- Handel N, Jensen JA, Black Q, et al. The fate of breast implants: a critical analysis of complications and outcomes. Plast Reconstr Surg 1995;96:1521-33. [Crossref] [PubMed]
- Li S, Mu D, Liu C, et al. Complications Following Subpectoral Versus Prepectoral Breast Augmentation: A Meta-analysis. Aesthetic Plast Surg 2019;43:890-8. [Crossref] [PubMed]
- Handel N, Silverstein MJ, Jensen JA, et al. Comparative experience with smooth and polyurethane breast implants using the Kaplan-Meier method of survival analysis. Plast Reconstr Surg 1991;88:475-81. [Crossref] [PubMed]
- Schaub TA, Ahmad J, Rohrich RJ. Capsular contracture with breast implants in the cosmetic patient: saline versus silicone--a systematic review of the literature. Plast Reconstr Surg 2010;126:2140-9. [Crossref] [PubMed]
- Li S, Chen L, Liu W, et al. Capsular Contracture Rate After Breast Augmentation with Periareolar Versus Other Two (Inframammary and Transaxillary) Incisions: A Meta-Analysis. Aesthetic Plast Surg 2018;42:32-7. [Crossref] [PubMed]
- Namnoum JD, Largent J, Kaplan HM, et al. Primary breast augmentation clinical trial outcomes stratified by surgical incision, anatomical placement and implant device type. J Plast Reconstr Aesthet Surg 2013;66:1165-72. [Crossref] [PubMed]
- Wiener TC. Relationship of incision choice to capsular contracture. Aesthetic Plast Surg 2008;32:303-6. [Crossref] [PubMed]
- Newman AN, Davison SP. Effect of Keller Funnel on the Rate of Capsular Contracture in Periareolar Breast Augmentation. Plast Reconstr Surg Glob Open 2018;6:e1834. [Crossref] [PubMed]
- Morkuzu S, Ozdemir M, Leach GA, et al. Keller Funnel Efficacy in "No Touch" Breast Augmentation and Reconstruction: A Systematic Review. Plast Reconstr Surg Glob Open 2022;10:e4676. [Crossref] [PubMed]
- Flugstad NA, Pozner JN, Baxter RA, et al. Does Implant Insertion with a Funnel Decrease Capsular Contracture? A Preliminary Report. Aesthet Surg J 2016;36:550-6. [Crossref] [PubMed]
- Bresnick SD. Higher Prevalence of Capsular Contracture with Second-side Use of Breast Implant Insertion Funnels. Plast Reconstr Surg Glob Open 2021;9:e3906. [Crossref] [PubMed]
- Dragun AE, Huang B, Gupta S, et al. One decade later: trends and disparities in the application of post-mastectomy radiotherapy since the release of the American Society of Clinical Oncology clinical practice guidelines. Int J Radiat Oncol Biol Phys 2012;83:e591-6. [Crossref] [PubMed]
- Magill LJ, Robertson FP, Jell G, et al. Determining the outcomes of post-mastectomy radiation therapy delivered to the definitive implant in patients undergoing one- and two-stage implant-based breast reconstruction: A systematic review and meta-analysis. J Plast Reconstr Aesthet Surg 2017;70:1329-35. [Crossref] [PubMed]
- Cordeiro PG, Albornoz CR, McCormick B, et al. The impact of postmastectomy radiotherapy on two-stage implant breast reconstruction: an analysis of long-term surgical outcomes, aesthetic results, and satisfaction over 13 years. Plast Reconstr Surg 2014;134:588-95. [Crossref] [PubMed]
- Borrelli MR, Irizzary D, Patel RA, et al. Pro-Fibrotic CD26-Positive Fibroblasts Are Present in Greater Abundance in Breast Capsule Tissue of Irradiated Breasts. Aesthet Surg J 2020;40:369-79. [Crossref] [PubMed]
- Moyer HR, Pinell-White X, Losken A. The effect of radiation on acellular dermal matrix and capsule formation in breast reconstruction: clinical outcomes and histologic analysis. Plast Reconstr Surg 2014;133:214-21. [Crossref] [PubMed]
- Lipa JE, Qiu W, Huang N, et al. Pathogenesis of radiation-induced capsular contracture in tissue expander and implant breast reconstruction. Plast Reconstr Surg 2010;125:437-45. [Crossref] [PubMed]
- Sigalove S. Prepectoral breast reconstruction and radiotherapy-a closer look. Gland Surg 2019;8:67-74. [Crossref] [PubMed]
- Brown MH, Somogyi RB, Aggarwal S. Secondary Breast Augmentation. Plast Reconstr Surg 2016;138:119e-35e. [Crossref] [PubMed]
- Codner MA, Mejia JD, Locke MB, et al. A 15-year experience with primary breast augmentation. Plast Reconstr Surg 2011;127:1300-10. [Crossref] [PubMed]
- Deva AK, Adams WP Jr, Vickery K. The role of bacterial biofilms in device-associated infection. Plast Reconstr Surg 2013;132:1319-28. [Crossref] [PubMed]
- Collis N, Mirza S, Stanley PR, et al. Reduction of potential contamination of breast implants by the use of 'nipple shields'. Br J Plast Surg 1999;52:445-7. [Crossref] [PubMed]
- Mladick RA. "No-touch" submuscular saline breast augmentation technique. Aesthetic Plast Surg 1993;17:183-92. [Crossref] [PubMed]
- de Bakker E, Rots M, Buncamper ME, et al. The Baker Classification for Capsular Contracture in Breast Implant Surgery Is Unreliable as a Diagnostic Tool. Plast Reconstr Surg 2020;146:956-62. [Crossref] [PubMed]
- Wan D, Rohrich RJ. Revisiting the Management of Capsular Contracture in Breast Augmentation: A Systematic Review. Plast Reconstr Surg 2016;137:826-41. [Crossref] [PubMed]
- Swanson E. Open Capsulotomy: An Effective but Overlooked Treatment for Capsular Contracture after Breast Augmentation. Plast Reconstr Surg Glob Open 2016;4:e1096. [Crossref] [PubMed]
- Little G, Baker JL Jr. Results of closed compression capsulotomy for treatment of contracted breast implant capsules. Plast Reconstr Surg 1980;65:30-3. [Crossref] [PubMed]
- Hipps CJ, Raju R, Straith RE. Influence of some operative and postoperative factors on capsular contracture around breast prostheses. Plast Reconstr Surg 1978;61:384-9. [Crossref] [PubMed]
- Moufarrege R, Beauregard G, Bosse JP, et al. Outcome of mammary capsulotomies. Ann Plast Surg 1987;19:62-4. [Crossref] [PubMed]
- Rohrich RJ, Parker TH 3rd. Aesthetic management of the breast after explantation: evaluation and mastopexy options. Plast Reconstr Surg 2007;120:312-5. [Crossref] [PubMed]
- Spear SL, Carter ME, Ganz JC. The correction of capsular contracture by conversion to "dual-plane" positioning: technique and outcomes. Plast Reconstr Surg 2003;112:456-66. [Crossref] [PubMed]
- Hidalgo DA, Spector JA. Breast augmentation. Plast Reconstr Surg 2014;133:567e-83e. [Crossref] [PubMed]
- Hidalgo DA, Weinstein AL. Surgical Treatment for Capsular Contracture: A New Paradigm and Algorithm. Plast Reconstr Surg 2020;146:516-25. [Crossref] [PubMed]
- Wang Y, Tian J, Liu J. Suppressive Effect of Leukotriene Antagonists on Capsular Contracture in Patients Who Underwent Breast Surgery with Prosthesis: A Meta-Analysis. Plast Reconstr Surg 2020;145:901-11. [Crossref] [PubMed]
- Graf R, Ascenço ASK, Freitas RDS, et al. Prevention of Capsular Contracture Using Leukotriene Antagonists. Plast Reconstr Surg 2015;136:592e-6e. [Crossref] [PubMed]
- Scuderi N, Mazzocchi M, Fioramonti P, et al. The effects of zafirlukast on capsular contracture: preliminary report. Aesthetic Plast Surg 2006;30:513-20. [Crossref] [PubMed]
- Minutello K, Gupta V. Cromolyn Sodium. 2023.
- Menkü Özdemir FD, Üstün GG, Kősemehmetoğlu K, et al. Comparison of Cromolyn Sodium, Montelukast, and Zafirlukast Prophylaxis for Capsular Contracture. Plast Reconstr Surg 2022;150:1005e-14e. [Crossref] [PubMed]
- Macías-Barragán J, Sandoval-Rodríguez A, Navarro-Partida J, et al. The multifaceted role of pirfenidone and its novel targets. Fibrogenesis Tissue Repair 2010;3:16. [Crossref] [PubMed]
- Gancedo M, Ruiz-Corro L, Salazar-Montes A, et al. Pirfenidone prevents capsular contracture after mammary implantation. Aesthetic Plast Surg 2008;32:32-40. [Crossref] [PubMed]
- Veras-Castillo ER, Cardenas-Camarena L, Lyra-Gonzalez I, et al. Controlled clinical trial with pirfenidone in the treatment of breast capsular contracture: association of TGF-β polymorphisms. Ann Plast Surg 2013;70:16-22. [Crossref] [PubMed]
- Caffee HH. Intracapsular injection of triamcinolone for intractable capsule contracture. Plast Reconstr Surg 1994;94:824-8. [Crossref] [PubMed]
- Clemens MW, Nava MB, Rocco N, et al. Understanding rare adverse sequelae of breast implants: anaplastic large-cell lymphoma, late seromas, and double capsules. Gland Surg 2017;6:169-84. [Crossref] [PubMed]
- Clemens MW, DeCoster RC, Fairchild B, et al. Finding Consensus After Two Decades of Breast Implant-Associated Anaplastic Large Cell Lymphoma. Semin Plast Surg 2019;33:270-8. [Crossref] [PubMed]
- Cordeiro PG, Ghione P, Ni A, et al. Risk of breast implant associated anaplastic large cell lymphoma (BIA-ALCL) in a cohort of 3546 women prospectively followed long term after reconstruction with textured breast implants. J Plast Reconstr Aesthet Surg 2020;73:841-6. [Crossref] [PubMed]
- Nelson JA, Dabic S, Mehrara BJ, et al. Breast Implant-associated Anaplastic Large Cell Lymphoma Incidence: Determining an Accurate Risk. Ann Surg 2020;272:403-9. [Crossref] [PubMed]
- Hu H, Johani K, Almatroudi A, et al. Bacterial Biofilm Infection Detected in Breast Implant-Associated Anaplastic Large-Cell Lymphoma. Plast Reconstr Surg 2016;137:1659-69. [Crossref] [PubMed]
- Rondón-Lagos M, Rangel N, Camargo-Villalba G, et al. Biological and genetic landscape of breast implant-associated anaplastic large cell lymphoma (BIA-ALCL). Eur J Surg Oncol 2021;47:942-51. [Crossref] [PubMed]
- Hall-Findlay EJ. Breast implant complication review: double capsules and late seromas. Plast Reconstr Surg 2011;127:56-66. [Crossref] [PubMed]
- Giot JP, Paek LS, Nizard N, et al. The double capsules in macro-textured breast implants. Biomaterials 2015;67:65-72. [Crossref] [PubMed]
- Buchanan PJ, Chopra VK, Walker KL, et al. Primary Squamous Cell Carcinoma Arising From a Breast Implant Capsule: A Case Report and Review of the Literature. Aesthet Surg J 2018;
- Kitchen SB, Paletta CE, Shehadi SI, et al. Epithelialization of the lining of a breast implant capsule. Possible origins of squamous cell carcinoma associated with a breast implant capsule. Cancer 1994;73:1449-52. [Crossref] [PubMed]
- Olsen DL, Keeney GL, Chen B, et al. Breast implant capsule-associated squamous cell carcinoma: a report of 2 cases. Hum Pathol 2017;67:94-100. [Crossref] [PubMed]
- Paletta C, Paletta FX Jr, Paletta FX Sr. Squamous cell carcinoma following breast augmentation. Ann Plast Surg 1992;29:425-9; discussion 429-32. [Crossref] [PubMed]
- Whaley RD, Aldrees R, Dougherty RE, et al. Breast Implant Capsule-Associated Squamous Cell Carcinoma: Report of 2 Patients. Int J Surg Pathol 2022;30:900-7. [Crossref] [PubMed]
- Zhou YM, Chaudhry HE, Shah A, et al. Breast Squamous Cell Carcinoma Following Breast Augmentation. Cureus 2018;10:e3405. [Crossref] [PubMed]
- Zomerlei TA, Samarghandi A, Terando AM. Primary Squamous Cell Carcinoma Arising from a Breast Implant Capsule. Plast Reconstr Surg Glob Open 2015;3:e586. [Crossref] [PubMed]
- Clemens MW, Jacobsen ED, Horwitz SM. 2019 NCCN Consensus Guidelines on the Diagnosis and Treatment of Breast Implant-Associated Anaplastic Large Cell Lymphoma (BIA-ALCL). Aesthet Surg J 2019;39:S3-S13. [Crossref] [PubMed]
- ASPS statement on Breast Implant Associated-Squamous Cell Carcinoma (BIA-SCC). Available online: https://www.plasticsurgery.org/for-medical-professionals/publications/psn-extra/news/asps-statement-on-breast-implant-associated-squamous-cell-carcinoma. 2022.
- McGuire P, Glicksman C, Wixtrom R, et al. Microbes, Histology, Blood Analysis, Enterotoxins, and Cytokines: Findings From the ASERF Systemic Symptoms in Women-Biospecimen Analysis Study: Part 3. Aesthet Surg J 2023;43:230-44. [Crossref] [PubMed]
- Wixtrom R, Glicksman C, Kadin M, et al. Heavy Metals in Breast Implant Capsules and Breast Tissue: Findings from the Systemic Symptoms in Women-Biospecimen Analysis Study: Part 2. Aesthet Surg J 2022;42:1067-76. [Crossref] [PubMed]
- Glicksman C, McGuire P, Kadin M, et al. Impact of Capsulectomy Type on Post-Explantation Systemic Symptom Improvement: Findings From the ASERF Systemic Symptoms in Women-Biospecimen Analysis Study: Part 1. Aesthet Surg J 2022;42:809-19. [Crossref] [PubMed]