Vagal electrostimulation in postoperative thoracic surgery reduces the systemic inflammatory response and cardiopulmonary complications: an experimental study in pigs
Highlight box
Key findings
• Vagal nerve stimulation maintained a more stable heart rate during post-operative period, decreasing cardiac complications;
• VNS prevents IL-6 increased levels after lobectomy.
What is known and what is new?
• Even though, VNS has been discussed as an anti-inflammatory neuromodulation tool, its use during post-operative period in invasive surgical procedures has not yet been completely elucidated. Here, we show that intra-operatory VNS prevents the cytokine storm triggered by lobectomy while prevents cardiac complications.
What is the implication, and what should change now?
• Intra-operatory and post-operative VNS should be further investigated in clinical trials aiming to comprehend the hemodynamics protection and attenuation of inflammatory response after surgical interventions.
Introduction
Thoracotomy is widely used for many surgical procedures, including pulmonary lobectomy, which is considered the gold standard treatment for an early-stage non-small cell lung cancer (1). Post-thoracotomy systemic inflammatory response syndrome (SIRS) is associated with an increased risk of complications, such as septic shock, multiple organ dysfunction, and death (2). Interestingly, increased levels of inflammatory cytokines in the plasma are related to SIRS and its complications (3). Hence, minimally invasive surgical procedures have been used widely to decrease strategically postoperative complications (4,5). Robotic surgery (RS) has a shorter median length of hospital stay (6), however, this type of surgery costs are significantly higher than those of thoracoscopic lobectomy or conventional thoracotomy (CT) (7-9). As a result, CT remains the most commonly used approach with higher complication rates (7).
Here, we propose the use of vagus nerve stimulation (VNS). VNS can induce parasympathetic cardiac events, such as chronotropy, dromotropy, inotropy, and lusitropy (10). Beyond visceral modulation, the vagus nerve is also a major component of the neuroendocrine and immune systems (11). The vagus nerve recognizes pro-inflammatory cytokines, such as TNF-α, IL-1, and IL-6, released by immune cells (12,13) and indirectly activates the hypothalamus-pituitary-adrenal axis, releasing glucocorticoids that decrease peripheral inflammation (14). Additionally, the release of acetylcholine by VNS activates α-7-nicotinic ACh receptors (a7nAChR), which inhibits the classical pro-inflammatory activation of macrophages (15). Interestingly, vagotomy increases the inflammatory response in a colitis pre-clinical model (16), while a7nAChR agonists have been used as therapeutic treatments (17). In this sense, the inhibition of exacerbated immune responses post-surgery, which induces systemic pro-inflammatory or immunosuppressive alternations (18), is a crucial strategy to control the complication rates, suggesting the role of VNS in different types of surgery.
Considering the beneficial effects of VNS on the inflammatory response, in this study, we aimed to compare hemodynamic features and cytokine levels in the blood, lung, and bronchoalveolar lavage (BAL) fluids of animals subjected to CT or RS with or without VNS. We expected to demonstrate that VNS may be used as a strategic tool to decrease the negative effects of pulmonary lobectomy, improve the surgical procedure, and improve the quality of life of individuals undergoing this type of surgery. We present this article in accordance with the ARRIVE reporting checklist (available at https://atm.amegroups.com/article/view/10.21037/atm-22-2919/rc).
Methods
Ethical statement
The protocols used during the execution of this project were approved by the Ethics Committee on the Use of Animals at Hospital Sírio-Libanês (Sao Paulo, Brazil) under protocol number CEUA 2014-08 and the Ethics Committee of the University of Sao Paulo Medical School, Sao Paulo, Brazil, under protocol number 018/16. Experiments were performed in compliance with international guidelines for the care and use of animals.
Experimental animals
Twenty-four male BR-1 minipigs (45–55 kg) obtained from Minipig Research and Development (Campina do Monte Alegre, SP, Brazil) were used in the study with sequential thoracotomy and sequential RS with vagal stimulation randomization.
Housing and husbandry
The minipigs were housed in the animal facilities of the Hospital Sírio-Libanês. The animals were maintained in appropriate rooms with a controlled light/dark cycle (12/12 h) and temperature (22±2 ℃) with free access to water and chow pellets.
Allocating animals to experimental groups
The animals were allocated in the CT group and RS group with randomization of the VNS in each group.
Outcomes measures
The primary outcome measure was to evaluate the hemodynamic features. The secondary outcome measure was to evaluate the cytokine comparing animals subjected to CT or RS with or without VNS.
Experimental procedure
Animals were kept fasting for 12 h in preparation for the surgical procedure. Animals were anesthetized (0.5 mg/kg, midazolam, i.m; 5 mg/kg, ketamine, i.m.; 8 mg/kg, propofol, i.v.), intubated (n. 8, Smartcam Android 5 mm), and mechanically ventilated (EVITA XL, Drager, Lubeck, Germany). General anesthesia was maintained at 11 µg/kg/h, 3 mg/kg/h, and midazolam 2 mg/kg/h, i.v.). The avascular sheath was introduced through the pulmonary artery for continuous blood pressure measurements, venous oxygen saturation, and final diastolic volume. Additionally, an avascular sheath was introduced through the right femoral artery to monitor the invasive blood pressure and collect arterial blood samples. Through cystostomy, a catheter was inserted to monitor the urine output. The animals received a continuous infusion of ringer lactate (4 mL/kg/h). Afterwards, the animal was placed in the right lateral decubitus position, with the left hemithorax exposed.
CT for pulmonary lobectomy and placement of vagal electrodes
The animals were submitted to an incision from the corresponding intercostal space at the rib’s upper edge until the opening of the pleural plane by lateral thoracotomy (approximately 15 cm). Lesions and pleuropulmonary adhesions were carefully investigated. Next, the electrodes were placed on the vagus nerve, which was identified by pleural dissection immediately above the azygos vein (Figure 1A).
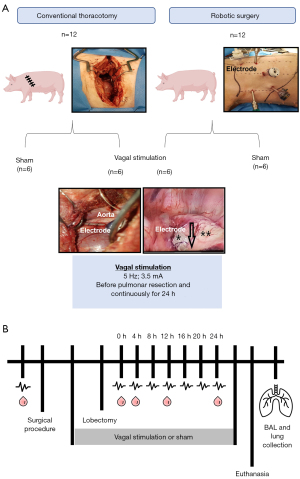
RS for pulmonary lobectomy and placement of vagal electrodes
RS was performed using the Da Vinci® Surgical System (Intuitive Surgical, CA, USA). All surgical and stimulation procedure were performed by the same surgeon. Five incisions were performed: (I) the camera incision was placed in the 8th intercostal space in the posterior axillary line at the level of the xiphoid appendage line; (II) scissors were incised in the 7th intercostal space; (III) incision for the dissection forceps was performed in the 6–7th intercostal space in the posterior vertebral line; (IV) an incision was made on the anterior axillary line in the 9th intercostal space for the placement of an endoscopic retractor; and finally; (V) a trocar was placed in the 5th intercostal space, at the paravertebral line as a cannula for the vagal electrode (Figure 1A). Animals were submitted to a CO2 lung insufflation of 6 mL with a pressure of 2 L/min.
Vagal stimulation
For both groups, CT and RS, the vagus nerve was dissected intraoperatively immediately before surgery in the mediastinal region above the azygos vein. After the electrode placement, VNS was performed using appropriated equipment (Resume® TL 3986ª Medtronic, 3.5 mA and 5 Hz) (19) before the pulmonary lobectomy model and for 24 h. Of note, the electrode implant did not induce any complications. Electrostimulation was confirmed by clinical observation from the surgeon when transitory bradycardia occurred with a decrease in the heart rate of approximately 20% of the original frequency and immediate recovery to both groups’ original parameters. The VNS was started intraoperatively immediately before the beginning of the dissection of the pulmonary hilum structures.
Additionally, a digital multi-meter (Multilaser®, China) was used in the CT group. For sham animals, the electrode was placed in the vagus nerve, but no stimulation was performed.
Pulmonary lobectomy
To make a pulmonary lobectomy, the left pulmonary hilum, pulmonary artery to the upper lobe, and left upper lobe bronchus were dissected (approximately 4 cm) in both groups. The surgical specimen was removed, and a rigorous review of the surgical site and animal hemostasis was performed, followed by thoracic drainage and skin sutures.
Measurements and sample collection
Data on the hemodynamic parameters, such as heart rate, blood pressure, central venous pressure, cardiac output, venous O2 saturation, body temperature (through the Swan Ganz catheter); urine output, and blood samples were collected before the surgical preparation (BM), immediately after the beginning of the CT or RS surgeries (0 h) and at 4, 8, 12, 16, 20, and 24 h after pulmonary lobectomy, with or without VNS. Hypercapnia was considered as CO2 expired fraction above 50, necessity of increase the respiratory rate above 20, and increase in the inhalation and exhalation ratio. Bradycardia was considered as heart rate below 40 after consistent application of atropine or presence of second-degree atrioventricular block. Blood samples were collected in EDTA-vacutainer tubes, and plasma was separated. Additionally, BAL from the left lung was collected at the end of each experiment (24 h after pulmonary lobectomy). Next, the lungs were inflated (with 25 cm3 air) and collected (Figure 1B). All collected samples were stored at −80 ℃ until measurement. After the end of the experiment animals were euthanized by anesthetic overdose and intravenous KCl.
Inflammatory evaluation
Lung tissues were gently homogenized at 4 ℃ in radioimmunoprecipitation assay (RIPA) buffer (50 mM Tris, 150 mM NaCl, 1 mM EDTA, 0.1% SDS, 0.5% deoxycholate, 1% NP-40) with fresh protease inhibitors. The Luminex assay was used to quantify the levels of IL-1α, IL-1β, IL-1ra, IL-2, IL-4, IL-6, IL-8, IL-10, IL-12, IL-18, TNF-α, IFN-γ, and GM-CSF in the serum, BALF, and lungs (PCYTMAG23PMX13BK, Merck-Millipore). The assay was performed following the manufacturer’s recommendations.
Statistical methods
The descriptive analyses for the quantitative data are presented as means ± standard deviation (SD). The normal distribution assumptions in each group and the homogeneity of the variances between the groups were evaluated using the Shapiro-Wilk and Levene tests. Categorical variables are expressed as frequencies and percentages. For the quantitative variables, where three factors were analyzed (surgery, stimulus, and time), the three-factor analysis of variance of repeated measures for a single factor (time) was used. For the quantitative variables, where two factors were analyzed (surgery and stimulus), the double factor analysis of variance was used. When it was necessary to perform multiple comparisons of means, the Bonferroni test was used. For comparison of proportions, the Chi-square or Fisher’s exact tests were used when necessary. The Kaplan-Meier curve was used to assess animal survival, and the log-rank test was used to compare the curves. Statistical analyses were performed using SPSSÒ Statistics software (version 21.0 for Windows) with a significance level of P<0.05.
Results
Clinical aspects of pulmonary lobectomy surgery and VNS
No significant difference was found in the time of surgery between sham and VNS animals on CT (P=0.19, Figure 2A) or RS (P=0.2, Figure 2B), even though there was a reduction of 10% and 14% of duration of the surgery, respectively, when using VNS, we think it was an isolated result and not influenced by VNS. Also, there were no surgical complications during the electrode implant. Interestingly, VNS maintained a more stable heart rate in the immediate postoperative recovery in the CT group (P=0.0019 regarding time parameter, Figure 2C). In the RS group, VNS stimulation slightly increased the heart rate at the last 12 h of the postoperative recovery (P<0.0001 compared to sham animals, Figure 2D). Considering that the CT increased the recovery complications, such as hypercapnia, bradycardia, and overall complications compared to the RS, we observed that VNS could significantly prevent desaturation, hypercapnia, bradycardia, atrial fibrillation and general cardiac complications (Table 1). As we simulated the immediate postoperative period (POP) of 24 h, we didn’t observe any infections and we did not have any deaths.
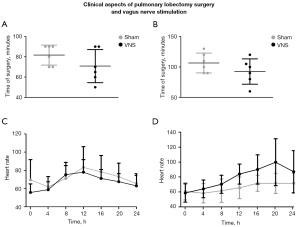
Table 1
Complications | Conventional thoracotomy | Robotic surgery | |||||
---|---|---|---|---|---|---|---|
Sham, n (%) | VNS, n (%) | P | Sham, n (%) | VNS, n (%) | P | ||
Desaturation | 5 (83.0) | 0 (0.0) | 0.015* | 1 (16.7) | 0 (0.0) | >0.999 | |
Hypercapnia | 5 (83.0) | 1 (17.0) | 0.08 | 3 (50.0) | 1 (16.7) | 0.55 | |
Bradycardia | 5 (83.0) | 0 (0.0) | 0.015* | 1 (16.7) | 0 (0.0) | >0.999 | |
Overall complications | 6 (100.0) | 2 (33.3) | 0.06 | 4 (66.7) | 1 (16.7) | 0.242 |
Hemodynamic parameters from the sham and vagus nerve stimulation groups submitted to conventional thoracotomy or robotic surgery. The frequency of events was evaluated by the Fisher’s exact test (n=6 per group). *, P<0.05 when compared to the sham animals. VNS, vagus nerve stimulation.
Inflammatory markers in pulmonary lobectomy surgery and VNS
We evaluated the levels of inflammatory cytokines in the serum of CT and RS animals with or without VNS at 0, 4, 12, and 24 h after the surgical procedure (Figure 3). We only found a relevant discrimination regarding CT submitted animals, that showed that VNS prevented a pro-inflammatory IL-6 increase in the serum 12 h after the surgical procedure (P<0.005, Figure 3A), but no significant difference was observed in the expression of IL-10 (P=0.87, Figure 3C) or IL-12 (P=0.36, Figure 3E). Similarly, we did not find any relevant difference after RS in the expression of IL-6 (P=0.87, Figure 3B) and IL-12 (P=0.69, Figure 3F). In the RS group with VNS, a 75% increase of the anti-inflammatory IL-10 in the serum was observed 24 h after the surgical procedure, although it was not statistically significant (P=0.07, Figure 3D). Curiously, we were unable to find any difference regarding the cytokine levels in the BAL fluid (Table 2) and lung tissue (Table 3), collected 24 h after the surgical procedure.
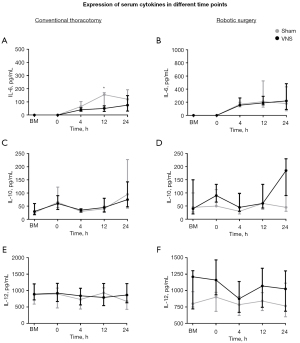
Table 2
Cytokine | Conventional thoracotomy | Robotic surgery | |||||
---|---|---|---|---|---|---|---|
Sham | Vagus nerve stimulation | P | Sham | Vagus nerve stimulation | P | ||
GM-CSF | 53.33±45.02 | 130.00±93.17 | 0.16 | 26.67±5.16 | 20.00±0.00 | 0.70 | |
IFN-γ | 596.67±429.82 | 1,666.67±1,146.64 | 0.18 | 1,435.00±551.35 | 948.33±237.10 | 0.06 | |
IL-1α | 251.67±249.91 | 408.33±630.16 | >0.99 | 1,261.67±1,807.08 | 280.00±223.25 | 0.24 | |
IL-1β | 12,015.00±12,326.18 | 12,566.67±24,558.53 | >0.99 | 9,425.00±10,350.29 | 2,495.00±1,599.91 | 0.13 | |
IL-1ra | 6,836.67±3,815.03 | 10,855.00±10,744.68 | 0.70 | 11,145.00±8,934.77 | 6,188.33±4,764.67 | 0.31 | |
IL-6 | 881.67±531.69 | 908.33±858.38 | 0.80 | 1,623.33±1,382.54 | 480.00±359.00 | 0.06 | |
IL-8 | 41,486.67±55,069.90 | 12,895.00±16,158.19 | 0.80 | 142,733.33±156,499.32 | 69,781.67±125,796.52 | 0.24 | |
IL-10 | 55.00±40.87 | 216.67±320.48 | 0.30 | 70.00±109.36 | 18.33±16.02 | 0.24 | |
IL-12 | 35.00±18.71 | 175.00±233.05 | 0.90 | 160.00±290.86 | 21.67±14.72 | 0.27 | |
IL-18 | 421.67±305.97 | 566.67±577.43 | 0.80 | 1,270.00±1,614.89 | 1,001.67±819.40 | >0.99 | |
TNF-α | 135.00±93.75 | 305.00±230.72 | 0.10 | 160.00±124.90 | 160.00±246.66 | 0.31 |
Cytokine expression (the unit for each cytokine is pg/mL) in the BAL fluid of animals submitted to conventional thoracotomy or robotic surgery and sham or vagus nerve stimulation 24 h after the surgical procedure. Data are presented as mean ± standard deviation evaluated by two-way analysis of variance followed by the Bonferroni post-hoc test (n=6 per group). BAL, bronchoalveolar lavage; GM-CSF, granulocyte-macrophage colony-stimulating factor; IFN, interferon; IL, interleukin; TNF, tumor necrosis factor.
Table 3
Cytokine | Conventional thoracotomy | Robotic surgery | |||||
---|---|---|---|---|---|---|---|
Sham | Vagus nerve stimulation | P | Sham | Vagus nerve stimulation | P | ||
GM-CSF | 23.36±12.30 | 18.05±13.20 | 0.30 | 34.20±50.52 | 11.97±8.61 | >0.99 | |
IFN-γ | 213.03±198.44 | 257.09±400.25 | 0.70 | 176.54±157.20 | 158.32±184.73 | >0.99 | |
IL-1α | 163.32±85.48 | 231.37±259.00 | 0.93 | 87.68±44.07 | 138.94±114.96 | 0.59 | |
IL-1β | 5,626.72±3,447.50 | 5,315.50±5,450.08 | 0.70 | 2160.85±565.35 | 2,422.61±1,783.69 | 0.93 | |
IL-1ra | 1,905.35±1,025.86 | 2,396.82±1,538.38 | 0.82 | 755.98±285.50 | 1,104.22±874.79 | 0.40 | |
IL-6 | 147.20±126.23 | 101.60±82.97 | 0.70 | 130.06±113.54 | 72.31±71.52 | 0.31 | |
IL-8 | 146.11±44.50 | 104.73±31.33 | 0.20 | 98.44±33.71 | 93.84±74.15 | 0.48 | |
IL-10 | 15.59±10.56 | 19.12±5.52 | 0.13 | 6.37±3.57 | 7.83±2.27 | 0.24 | |
IL-12 | 39.65±15.78 | 100.64±58.65 | 0.06 | 41.44±27.52 | 31.87±23.21 | 0.48 | |
IL-18 | 6,558.80±1,692.93 | 7,657.79±2,394.72 | >0.99 | 4,124.71±1,257.05 | 4,999.48±3,745.66 | >0.99 | |
TNF-α | 37.59±15.69 | 38.37±48.22 | 0.40 | 36.07±47.08 | 11.12±7.39 | 0.94 |
Cytokine expression (the unit for each cytokine is pg/mg of total protein) in the lung of animals submitted to conventional thoracotomy or robotic surgery and sham or vagus nerve stimulation 24 h after the surgical procedure. Data are presented as mean ± standard deviation evaluated by two-way analysis of variance followed by the Bonferroni post-hoc test (n=6 per group). GM-CSF, granulocyte-macrophage colony-stimulating factor; IFN, interferon; IL, interleukin; TNF, tumor necrosis factor.
Since IL-6 was differentially expressed in the CT group when comparing sham and VNS animals 12 h after the surgical procedure (P<0.05, Figure 4A), we sought to understand the consequences of IL-6 expression in another pro-inflammatory cytokine, IL-12. The increase of IL-6 at 12 h showed a moderate negative correlation with IL-12 levels in the serum (r=−0.6, P=0.02, Figure 4B) and in the lung tissue (r=−0.6, P=0.03, Figure 4C) at a later time point (24 h). When comparing the anti-inflammatory cytokine IL-10 to IL-6 or IL-12 in the serum, we observed no correlation; however, IL-10 levels in the serum at 24 h showed a strong negative correlation with IL-6 expression in the BAL fluid 24 h after the surgical procedure (r=−0.7, P=0.008, Figure 4D), indicating the relevance of IL-6 control in this model.
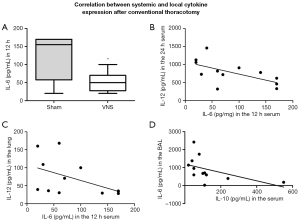
Discussion
Early preventive anti-inflammatory strategies are required to reduce the overall complications considering the importance of inflammation in major invasive surgery, as in pulmonary lobectomy. This study demonstrated the clinical aspects and local and systemic inflammatory profile of VNS impact in CT and RS in an animal pre-clinical model. In CT, where surgery is indisputably more invasive than RS, we found that VNS can stabilize heart rate and decrease clinical complications, such as desaturation, hypercapnia, and bradycardia in the immediate POP (which is considered 24 h after the surgical procedure). Interestingly, VNS prevented the increase of IL-6 systemic levels at 12 h in the CT model and augmented systemic IL-10 levels at 24 h after the surgical procedure in the RS group.
Thoracic surgery is a major risk factor for arrhythmia in 20% of cases, especially atrial fibrillation and supraventricular tachycardia (20). We showed that VNS might act as a cardioprotective strategy, considering that stimulated animals did not present any of the overall complications observed in the sham CT group. Our results corroborate previous findings suggesting that VNS protects against cardiac events by vasodilatory properties and activation of stellate stimulation, which induces a balance between parasympathetic and sympathetic pathways (21). As CO2 insufflation in the lungs is required for pulmonary lobectomy, it often leads to bradycardia and arterial hypotension (22). A trend to higher heart rates in VNS animals during robotic procedures, our hypothesis is even with a low pressure used (6 mmHg) we observed that the pressure caused a small amount of hypotension and a drop-in heart rate. But in the group VNS RS, here was a better cardio-hemodynamic adaptation in the POP, with the animals showing a higher heart rate during the 24 hours of experiment. Our hypothesis is that the vagal stimulus releases Vasoactive Intestinal Peptide (VIP) or a “VIP-like” substance that significantly increases contraction right ventricular and heart rate (23). CT has higher complication rates, and VNS could be a potential therapeutic tool in improving postoperative outcomes.
To further investigate the mechanisms of VNS in both CT and RS, we evaluated the expression of pro-and anti-inflammatory cytokines in the serum, BAL fluid, and lung tissue of all animals. We found that VNS could prevent IL-6 increase 12 h after the surgical procedure in the CT group, while IL-10 was found to be discretely increased 24 h after the surgical procedure in the RS group. IL-6 is a pleiotropic cytokine that induces pro-inflammatory responses. It also modulates the inflammatory cascade by negative feedback, initiating an anti-inflammatory and reparative cascade (24). In line with this finding, it has also been shown in humans that autonomic neuromodulation, including VNS can reduce IL-6 after cardiac surgery (25). IL-10 is a classic anti-inflammatory cytokine promoting tissue repair in a rodent model of pulmonary inflammation (26). Of note, even though we found significant differences in systemic inflammatory markers during the immediate POP, we did not find any differences in the BAL fluid and lung tissue. This is probably due to a methodological limitation, where blood can be collected throughout the 24 h, and BAL fluid and lung can only be collected at a one-time point. Interestingly, we found an inverse correlation between systemic IL-6 levels at 12 h and systemic IL-12 levels 24 h after the surgical procedure in the sham CT group. IL-12 is a pro-inflammatory cytokine required for IFN-γ activity in lymphocytes (27). Of note, IL-12 is also pivotal for IL-10 production and release in an attempt to balance the inflammatory response (28).
Here, we showed that VNS prevented systemic IL-6 increase 12 h after lobectomy. It is essential to highlight that systemic inflammation is a response to local inflammation (18). Hence, our data suggest that CT increases IL-6 levels in the serum, probably due to a local inflammatory response. This IL-6 increase observed 12 h after the surgical procedure leads to negative physiological feedback of local inflammation, decreasing IL-12 in the lung tissue and the serum 24 h after the surgical procedure. However, this can lead to undesirable complications. The role of IL-6 in inflammatory models has been extensively described (29,30) and the blockade of IL-6 (tocilizumab) has been applied for inflammatory diseases (31). Since VNS can restrain IL-6 increase after 12 h of the surgical procedure, it could improve surgical recovery or could even be used as a possible therapeutic alternative for inflammatory diseases. Similarly, IL-6 expression in the BAL fluid was negatively correlated with IL-10 expression in the serum 24 h after the surgical procedure. Therefore, the slight increase of IL-10 in the serum observed in VNS animals may be due to a reparative role where the stimulation responds in a specific time window to prevent exacerbated inflammation. In this sense, our work proposes that the inflammatory signature of VNS stimulation includes systemic IL-6 and IL-10 levels.
Considering the stimulation parameters, a previous work (32) applied high-frequency electric stimulation (20 Hz, 0.1 ms duration, square waves) in comparison with our work that used the lowest voltage of high-frequency that induced atrial fibrillation. The main reason for our choice was the individualized treatment parameters based on each animal’s response.
Moreover, we showed that VNS could be an optimal strategy to prevent CT’s overall complications by balancing the hemodynamics and inflammatory aspects. However, in RS, a less invasive surgery, VNS plays a role, especially in improving heart rate. Although less invasive surgical procedures may be the future, to this date, due to financial resources, CT is still widely used. Of note, video-assisted thoracic surgery may also be used as a valued minimal invasive and less expansive tool that was not evaluated in the present work. Moreover, in our work the VNS was invasive due to limitations in preclinical settings, however, in patients the VNS would be done transcutaneous to prevent another invasive treatment. Hence, we suggest VNS as an advanced methodology aiming to prevent SIRS and cardiac events, which may improve the quality of life of individuals with elective pulmonary lobectomy. However, further studies should be conducted to understand the mechanisms of VNS and the optimal stimulation protocol for inducing balance between the parasympathetic and sympathetic systems. Currently auricular vagus nerve stimulation (aVNS) delivered percutaneously or transcutaneously offers a method to modulate neural activity on the vagus nerve with the potential for a more favorable safety profile. The auricular branch of the vagus nerve runs superficially, which makes it a favorable target for non-invasive stimulation techniques to modulate vagal activity (33) and may facilitate the clinical use of VNS in thoracic surgery.
Limitations
Our study’s limitations included the inability to evaluate the length of hospital stay after surgery and the evaluation of further complications during the follow-up period. The study also contains a technical limitation concerning the Luminex assay. Protein quantitation might present variation of absolute concentration as described previously (34,35), but not in relative concentration. Since we had a considerable large number of samples, we performed the analysis in different plates, which might affect the comparison of cytokines between CT versus RS group. We emphasize that this limitation does not impact on the results and conclusions presented here, once we were cautions to make the comparisons restricted to each surgical procedure.
Conclusions
Our results suggest the potential benefits of VNS during CT procedures regarding cardioprotective and anti-inflammatory effects and highlight the importance of updating surgical protocols to prevent possible complications.
Acknowledgments
Funding: This research was supported by
Footnote
Reporting Checklist: The authors have completed the ARRIVE reporting checklist. Available at https://atm.amegroups.com/article/view/10.21037/atm-22-2919/rc
Data Sharing Statement: Available at https://atm.amegroups.com/article/view/10.21037/atm-22-2919/dss
Peer Review File: Available at https://atm.amegroups.com/article/view/10.21037/atm-22-2919/prf
Conflicts of Interest: All authors have completed the ICMJE uniform disclosure form (available at https://atm.amegroups.com/article/view/10.21037/atm-22-2919/coif). RMT serves as a speaker/proctor for Medtronic, Strattner/Intuitive, AstraZeneca, MSD, BMS. The other authors have no conflicts of interest to declare.
Ethical Statement: The authors are accountable for all aspects of the work in ensuring that questions related to the accuracy or integrity of any part of the work are appropriately investigated and resolved. The protocols used during the execution of this project were approved by the Ethics Committee on the Use of Animals at Hospital Sírio-Libanês (Sao Paulo, Brazil) under protocol number CEUA 2014-08 and the Ethics Committee of the University of Sao Paulo Medical School, Sao Paulo, Brazil, under protocol number 018/16. Experiments were performed in compliance with international guidelines for the care and use of animals.
Open Access Statement: This is an Open Access article distributed in accordance with the Creative Commons Attribution-NonCommercial-NoDerivs 4.0 International License (CC BY-NC-ND 4.0), which permits the non-commercial replication and distribution of the article with the strict proviso that no changes or edits are made and the original work is properly cited (including links to both the formal publication through the relevant DOI and the license). See: https://creativecommons.org/licenses/by-nc-nd/4.0/.
References
- Ettinger DS, Wood DE, Aisner DL, et al. Non-Small Cell Lung Cancer, Version 5.2017, NCCN Clinical Practice Guidelines in Oncology. J Natl Compr Canc Netw 2017;15:504-35. [Crossref] [PubMed]
- Sengupta S. Post-operative pulmonary complications after thoracotomy. Indian J Anaesth 2015;59:618-26. [Crossref] [PubMed]
- Takenaka K, Ogawa E, Wada H, et al. Systemic inflammatory response syndrome and surgical stress in thoracic surgery. J Crit Care 2006;21:48-53; discussion 53-5. [Crossref] [PubMed]
- Villamizar NR, Darrabie MD, Burfeind WR, et al. Thoracoscopic lobectomy is associated with lower morbidity compared with thoracotomy. J Thorac Cardiovasc Surg 2009;138:419-25. [Crossref] [PubMed]
- Ceppa DP, Kosinski AS, Berry MF, et al. Thoracoscopic lobectomy has increasing benefit in patients with poor pulmonary function: a Society of Thoracic Surgeons Database analysis. Ann Surg 2012;256:487-93. [Crossref] [PubMed]
- Yang HX, Woo KM, Sima CS, et al. Long-term Survival Based on the Surgical Approach to Lobectomy For Clinical Stage I Nonsmall Cell Lung Cancer: Comparison of Robotic, Video-assisted Thoracic Surgery, and Thoracotomy Lobectomy. Ann Surg 2017;265:431-7. [Crossref] [PubMed]
- Oh DS, Reddy RM, Gorrepati ML, et al. Robotic-Assisted, Video-Assisted Thoracoscopic and Open Lobectomy: Propensity-Matched Analysis of Recent Premier Data. Ann Thorac Surg 2017;104:1733-40. [Crossref] [PubMed]
- Paul S, Jalbert J, Isaacs AJ, et al. Comparative effectiveness of robotic-assisted vs thoracoscopic lobectomy. Chest 2014;146:1505-12. [Crossref] [PubMed]
- Park BJ, Flores RM. Cost comparison of robotic, video-assisted thoracic surgery and thoracotomy approaches to pulmonary lobectomy. Thorac Surg Clin 2008;18:297-300. vii. [Crossref] [PubMed]
- Randall WC, Ardell JL, Becker DM. Differential responses accompanying sequential stimulation and ablation of vagal branches to dog heart. Am J Physiol 1985;249:H133-40. [PubMed]
- Johnston GR, Webster NR. Cytokines and the immunomodulatory function of the vagus nerve. Br J Anaesth 2009;102:453-62. [Crossref] [PubMed]
- Tracey KJ. The inflammatory reflex. Nature 2002;420:853-9. [Crossref] [PubMed]
- Goehler LE, Gaykema RP, Hansen MK, et al. Vagal immune-to-brain communication: a visceral chemosensory pathway. Auton Neurosci 2000;85:49-59. [Crossref] [PubMed]
- Bonaz B, Sinniger V, Pellissier S. Anti-inflammatory properties of the vagus nerve: potential therapeutic implications of vagus nerve stimulation. J Physiol 2016;594:5781-90. [Crossref] [PubMed]
- Pavlov VA, Wang H, Czura CJ, et al. The cholinergic anti-inflammatory pathway: a missing link in neuroimmunomodulation. Mol Med 2003;9:125-34. [Crossref] [PubMed]
- Ghia JE, Blennerhassett P, Kumar-Ondiveeran H, et al. The vagus nerve: a tonic inhibitory influence associated with inflammatory bowel disease in a murine model. Gastroenterology 2006;131:1122-30. [Crossref] [PubMed]
- van Westerloo DJ, Giebelen IA, Florquin S, et al. The vagus nerve and nicotinic receptors modulate experimental pancreatitis severity in mice. Gastroenterology 2006;130:1822-30. [Crossref] [PubMed]
- Alazawi W, Pirmadjid N, Lahiri R, et al. Inflammatory and Immune Responses to Surgery and Their Clinical Impact. Ann Surg 2016;264:73-80. [Crossref] [PubMed]
- Czura CJ, Schultz A, Kaipel M, et al. Vagus nerve stimulation regulates hemostasis in swine. Shock 2010;33:608-13. [Crossref] [PubMed]
- Imperatori A, Mariscalco G, Riganti G, et al. Atrial fibrillation after pulmonary lobectomy for lung cancer affects long-term survival in a prospective single-center study. J Cardiothorac Surg 2012;7:4. [Crossref] [PubMed]
- Huang WA, Shivkumar K, Vaseghi M. Device-based autonomic modulation in arrhythmia patients: the role of vagal nerve stimulation. Curr Treat Options Cardiovasc Med 2015;17:379. [Crossref] [PubMed]
- Ohtsuka T, Imanaka K, Endoh M, et al. Hemodynamic effects of carbon dioxide insufflation under single-lung ventilation during thoracoscopy. Ann Thorac Surg 1999;68:29-32; discussion 32-3. [Crossref] [PubMed]
- Henning RJ, Feliciano L, Coers CM. Vagal nerve stimulation increases right ventricular contraction and relaxation and heart rate. Cardiovasc Res 1996;32:846-53. [Crossref] [PubMed]
- Scheller J, Chalaris A, Schmidt-Arras D, et al. The pro- and anti-inflammatory properties of the cytokine interleukin-6. Biochim Biophys Acta 2011;1813:878-88. [Crossref] [PubMed]
- Zafeiropoulos S, Doundoulakis I, Farmakis IT, et al. Autonomic Neuromodulation for Atrial Fibrillation Following Cardiac Surgery: JACC Review Topic of the Week. J Am Coll Cardiol 2022;79:682-94. Erratum in: J Am Coll Cardiol 2022;79:1966. [Crossref] [PubMed]
- Zhao Y, Xiong Z, Lechner EJ, et al. Thrombospondin-1 triggers macrophage IL-10 production and promotes resolution of experimental lung injury. Mucosal Immunol 2014;7:440-8. [Crossref] [PubMed]
- Trinchieri G. Interleukin-12: a cytokine produced by antigen-presenting cells with immunoregulatory functions in the generation of T-helper cells type 1 and cytotoxic lymphocytes. Blood 1994;84:4008-27. [Crossref] [PubMed]
- Ma X, Yan W, Zheng H, et al. Regulation of IL-10 and IL-12 production and function in macrophages and dendritic cells. F1000Res 2015;4:F1000 Faculty Rev-1465.
- Nowell MA, Williams AS, Carty SA, et al. Therapeutic targeting of IL-6 trans signaling counteracts STAT3 control of experimental inflammatory arthritis. J Immunol 2009;182:613-22. [Crossref] [PubMed]
- Greenhill CJ, Rose-John S, Lissilaa R, et al. IL-6 trans-signaling modulates TLR4-dependent inflammatory responses via STAT3. J Immunol 2011;186:1199-208. [Crossref] [PubMed]
- Scott LJ. Tocilizumab: A Review in Rheumatoid Arthritis. Drugs 2017;77:1865-79. [Crossref] [PubMed]
- Li S, Scherlag BJ, Yu L, et al. Low-level vagosympathetic stimulation: a paradox and potential new modality for the treatment of focal atrial fibrillation. Circ Arrhythm Electrophysiol 2009;2:645-51. [Crossref] [PubMed]
- Verma N, Mudge JD, Kasole M, et al. Auricular Vagus Neuromodulation-A Systematic Review on Quality of Evidence and Clinical Effects. Front Neurosci 2021;15:664740. [Crossref] [PubMed]
- Verma N, Mudge JD, Kasole M, et al. Auricular Vagus Neuromodulation-A Systematic Review on Quality of Evidence and Clinical Effects. Front Neurosci 2021;15:664740. [Crossref] [PubMed]
- Fang R, Wey A, Bobbili NK, et al. An analytical approach to reduce between-plate variation in multiplex assays that measure antibodies to Plasmodium falciparum antigens. Malar J 2017;16:287. [Crossref] [PubMed]