An evaluation of the total ankle replacement in the modern era: a narrative review
Introduction
Total ankle replacement (TAR) has undergone tremendous developments since its inception 50 years ago. In the modern era, there has been immense growth in annual volumes, increasing by 564% between 2005 to 2017 (1), as indications for surgery expand, surgical techniques refine, and outcomes improve. Concurrently, the literature surrounding ankle replacement continues to evolve as new insights and discoveries are published. At this time, the field of TAR has introduced its fourth generation of implants, increased its surgical indications, improved its implant survivorship, minimized its complications, and developed new technology aimed to optimize patient outcomes.
This review aims to summarize the current knowledge of TAR as described through its evolution, improvements, and future directions. We present this article in accordance with the Narrative Review reporting checklist (available at https://atm.amegroups.com/article/view/10.21037/atm-23-1569/rc).
Methods
We reviewed the results of clinical studies and meta-analyses of TARs presented on PubMed. These articles included topics about the history, indications, surgical technique, complications, outcomes, and future of TARs, as well as comparative studies between TARs and ankle arthrodesis. These articles were identified using the following keywords: “total ankle replacement”, “total ankle arthroplasty”, and “total ankle” (Table 1).
Table 1
Items | Specification |
---|---|
Date of search | March 1, 2023 |
Databases and other sources searched | PubMed |
Search terms used | Total ankle replacement, total ankle arthroplasty, and total ankle |
Timeframe | English abstracts and articles before March 2023 |
Inclusion criteria | English abstracts and articles |
Selection process | Literature search was conducted by I.S. Final approval of literature search was conducted by all authors |
Discussion
History
Prior to the introduction of ankle replacements, the only operative treatment option for end-stage ankle arthritis was ankle arthrodesis, considered the ‘gold-standard’ treatment. Though the results of ankle arthrodesis were shown to achieve good clinical outcomes and high satisfaction scores, concerns about complications and functional outcomes still existed (2,3). Namely, ankle arthrodesis had complications with nonunion, malunion, and infection following surgery, with limited salvage options (4). Furthermore, patients with ankle arthrodesis experienced decreased sagittal plane motion in the hindfoot, slower, asymmetrical gait, and ultimately, degenerative arthritis changes in adjacent joints (5-7). These shortcomings in ankle arthrodesis prompted exploration in alternative treatment options, including joint replacement.
First generation
The first generation of TAR implants, developed in the 1970s, featured a variety of designs that attempted to mimic the successful features of hip and knee arthroplasty. Though there was wide variability in prosthesis designs, the basic model of this generation featured a two-part system with a polyethylene concave articular component and a convex cobalt chrome metal component. This generation had both constrained and unconstrained systems, each with their own ramifications contributing to high failure rates and unsatisfactory outcomes. Constrained systems limited the dissipation of stresses between the contact surfaces, contributing to high rates of loosening; unconstrained systems placed increased stresses on the surrounding ligaments of the ankle, leading to problems of malalignment (8,9). Moreover, this early generation of implants used cemented fixation and required extensive bone resection to properly position the component. This first generation of implants encountered several issues: high rates of loosening (between 29–90% at 10 years), low satisfaction scores, and poor survivorship (8-11). The poor results associated with the implants of this generation led to the complete abandonment of these designs. Nonetheless, this generation offered immense insight for improvements in the future generations of implants, including considerations about minimizing bony resection, balancing soft tissue, and decreasing shear stress forces (10,12).
Second generation
The second generation of TAR was introduced to the market in the mid-1980s, incorporating novel implant features that attempted to address the shortcomings of the previous generation. Poor outcomes attributed to cemented fixation and large bone resection in the first generation led to a transition towards cementless implants in the second generation. In addition, these designs featured porous-coated metallic tibial implants intended to stimulate osseous integration and decrease high rates of loosening.
In the previous generation, both highly constrained and highly unconstrained designs were associated with a litany of complications. Learning from this insight, the second generation of TAR developed two categories of implants, fixed-bearing and mobile-bearing, that hoped to mitigate previous shortcomings. Fixed-bearing implants consist of a tibial, talar, and fixed polyethylene component, that function as a two-component implant. In contrast, mobile-bearing implants feature the same three components (tibia, talus, and polyethylene), but implement an unconstrained polyethylene insert that can articulate between the tibial and talar components (11,13,14). Fixed-bearing implants have higher constraint than mobile-bearing, which allows for greater stability, but also increases the risk of implant loosening. Though mobile-bearing implants have minimal constraint across the polyethylene insert to decrease load stress, there are still concerns about polyethylene wear, instability, and translation. Outcomes were still largely variable in the second-generation designs. In one meta-analysis of 1,105 second generation TARs, the average survivorship across 7 implants was 90% at 5 years, though survivorship ranged between 68% to 100% across different studies (13). Further, this generation cited residual issues with implant subsidence, residual pain, and limited range of motion (13).
Modern generations (third and fourth)
After the first two generations of TAR, modern implants were refined to minimize bony resection and respect local anatomy. Moreover, surgeons had greater appreciation for mechanical alignment and balancing the ankle with additional bony and soft tissue procedures to ensure a stable ankle and foot around the replacement. Modern implants include both fixed-bearing, two-component designs and mobile-bearing, three-component designs; although the former is more common in the United States, the latter in Europe. Acknowledging the pattern of failure associated with cemented implantation, third-generation implants featured cementless designs, utilizing titanium plasma-spray coatings for bone ingrowth (15). Popular third-generation implants include the INBONE (Wright Medical, Memphis, TN, USA), Salto Talaris (Integra Lifesciences, Princeton, NJ, USA), STAR (Stryker, Kalamazoo, MI, USA), and HINTEGRA (Integra Life Sciences, Newdeal, Lyon, France) and have been associated with good survivorship and high satisfaction scores.
The INBONE total ankle implant, originally created in 2005, features a unique modular stem tibial design and intermedullary stem alignment guide with the intention to maximize bony fixation (16). The original INBONE prosthesis (INBONE I) employed a flat-cut, saddle talar component that similarly featured a robust talar stem; however, after reports of talar-sided failures, the talar component was revised in its second iteration (INBONE II) (16). Using an external jig to secure the leg and fluoroscopy to achieve proper alignment, the tibial component is implanted via intramedullary reaming through the calcaneus and talus. The INBONE TAR can be used in both primary and revision settings, performing as a viable alternative in cases of failed TAR with loosening and bone loss. Moreover, added stability from the robust tibial stem allows the INBONE implant to be a reasonable option for patients with severe deformity or instability (15).
Surgical outcomes of the INBONE I TAR report survivorship of 89% at only 3.7 years of follow-up, with high incidence of talar subsidence (17). Further studies have identified the INBONE I as an independent risk factor for failure, again citing talar subsidence as the primary reason for revision (18). There has been some evidence to suggest the high incidence of talar subsidence is a result of talar osteonecrosis instigated by the intraoperative intermedullary reaming, though no definitive cause has been elucidated (19). In 2010, a revised version of the implant (INBONE II) was introduced, which implemented a sulcus-shaped profile and two anterior pegs to the talar component. Midterm outcomes of the INBONE II report survivorship of 98% and decreased incidence of talar subsidence (20).
The Salto Talaris fixed-bearing TAR was first introduced to the United States market in 2006. However, this was an adaptation to its mobile-bearing predecessor, the Salto Total Ankle, that had been used in Europe since 1997. The implant features a central keel in tibia and conical-shaped facet in the talar component, designed to optimize natural alignment of the patient’s rotational axis (15). Clinical outcomes of the Salto Talaris at midterm follow-up cite excellent survivorship and improvements in pain, though durability of the implant at the 10-year milestone remains undetermined (21-23).
The first iteration of the STAR implant was initially introduced in 1978, and five different versions of the STAR have been used for implantation since 1981. The most recent iteration is the 4th-generation STAR, which was approved in the United States in 2009 (15). The implant features a three-component, mobile-bearing design, with a tibial component with two cylindrical bars for fixation and a symmetrical, cylindrical talar component (15). The distal side of the tibial component has a smooth, flat surface that enables unconstrained motion for the polyethylene insert. The version of the STAR used in the United States possesses a titanium plasma spray to stimulate bone ongrowth, distinct from its European counterparts (15). Long-term outcomes of the STAR in the United States have reported survivorship rates between 90% to 95% at 10 years, which decreased to 73% at 15 years (24-26).
The HINTEGRA Total Ankle similarly was a three-component mobile-bearing implant prominently used in Europe, Canada, and Brazil following its approval in the early 2000s. The tibial component features a flat surface with an anterior shield that has two holes for screw fixation in the tibia. The talar component has a conical profile and employs an anterior shield with holes for screw fixation as well. Several long-term large cohort studies assessing survivorship of the HINTEGRA prosthesis have been published reporting varying survivorship ranging between 68% to 84% at 10 years postoperatively (27,28).
The Zimmer Trabecular Metal Total Ankle (Warsaw, IN, USA) was a notable introduction to the third generation of TAR implants. Contrary to other implants, which use an anterior surgical approach to the ankle joint, the Trabecular Metal TAR employs a lateral transfibular approach, which requires fibular osteotomy and anterior talofibular ligament resection to access the joint. The rationale behind this technique was to allow better replication of the natural curvature of the tibia and talus and minimize bone resection. Additionally, it was theorized that this approach would decrease incidence of wound healing complications (15). Midterm outcomes of the Trabecular Metal TAR have reported good implant survivorship and improved functional scores at 5 years (29,30). However, in one small case series of 16 lateral approach TAR patients, there was a 25% incidence of complications associated with the fibular osteotomy (31). Though this implant has demonstrated good survivorship and patient-reported outcomes, the increased risk of fibular nonunion, as well as the challenges for revision of the implant, remain a concern (30).
The fourth generation of TAR implants continues to improve upon the strengths of the third generation to optimize bone integration, mechanical alignment, and surgical technique. In the United States, modern fourth-generation implants include INFINITY (Stryker), Cadence (Integra LifeSciences, Princeton, NJ, USA), Vantage (Exactech, Gainesville, FL, USA), Axiom (Kinos, Wayne, PA, USA), Apex (Paragon 28, Englewood, CO, USA), Quantum (In2Bones, Memphis, TN, USA). These designs feature low-profile tibial and talar components which minimize bone resection while still maintaining robust surface contact (15). Given their relative novelty of these implants, the long-term outcomes are uncertain; however, early reports demonstrate good survivorship ranging between 92% to 98%, and significant improvements in functional and pain scores postoperatively in the first two years (32-34). Long-term follow-up and studies will be critical in the evaluation of implant survivorship after the early and mid-term periods.
Additional innovation in the field of TAR has led to the development of revision ankle implants. In the past, treatment options for TAR implant failure were limited to arthrodesis or below-knee amputation (9,11,35). In the modern era, the INBONE implant has commonly been used in the revision TAR setting (36), but there is significant room for improvement in the treatment of failed TAR. Currently, the only available revision systems on the market are the INVISION (Stryker) and Salto Talaris XT (Integra LifeSciences), which are designed for settings of large bone resection and augmented instability. Reports on revision TAR system outcomes are largely limited and require further investigation. It is expected that novel revision systems will continue to enter the market as more companies invest in this future direction of TAR.
Indications
The primary indication for TAR is end-stage ankle arthritis, which is identified through clinical and radiographic assessment. As the frequency of TARs performed each year increase, understanding of etiology of arthritis and associated outcomes remains a pertinent area of research. Post-traumatic arthritis is the most common etiology of ankle arthritis, accounting for between 70–90% of all incidences of end-stage ankle osteoarthritis (20,23,34,37); however, trauma may range from intra-articular ankle or talus fracture to extra-articular fracture, chondral injury, or chronic ligamentous insufficiency and instability. Other etiologies of ankle arthritis include primary osteoarthritis, inflammatory arthritis, and arthritis secondary to clubfoot deformity, avascular necrosis (AVN), or hemochromatosis.
Historically, the ideal TAR candidate was an older patient with low functional demands, minimal deformity at the ankle or foot, and minimal adjacent joint arthrosis. These characteristics have been associated with greater pain resolution, diminished complication risks, and lower risks of failure. However, improvements in surgeon experience, technique, and implant designs have contributed excellent outcomes in patient demographics beyond “ideal” criteria.
Age and physical demand are considered to have significant influence upon TAR outcomes. In particular, younger and more physically active patients have been thought to have an increased risk of failure in TAR, as a result of the increased implant lifespan and activity demand. However, some reports explicitly investigating outcomes of TAR by age groups have found no significant differences in risk (38,39), while others cite age as an independent predictor of failure (35,40). Despite conflicting evidence, younger patients still report excellent functional and clinical outcomes that warrants eligibility for TAR (39,41,42). In particular, the preservation of motion from TAR is especially beneficial for younger patients, as it can help to diminish future onset and severity of adjacent joint arthritis in the midfoot and hindfoot. In general, patient age and activity level should be considered in pre-surgical consultation, and surgeons should take these factors into account to guide decision-making and to manage patients’ expectations of outcome.
Preoperative coronal plane deformity has been cited as a relative contraindication for TAR historically. However, more recent studies demonstrate that severe preoperative deformity does not result in increased failure, as long as the deformity is able to be corrected intraoperatively (43). Current analysis of TAR outcomes in the setting of varus, valgus, and neutral preoperative alignment has reported similar pain and functional scores and similar rates of complications, reoperation, and survivorship across the three groups (44). Though preoperative coronal deformity exceeding 20° once was considered an absolute contraindication for TAR, advancements in surgical technique and implant design have helped achieve satisfactory outcomes for cases of severe coronal plane deformity (20° to 35° of varus or valgus) (45). Importantly, ensuring good outcomes in cases of foot and ankle deformity is dependent upon the use of concomitant procedures to balance the ankle.
Obesity has also been cited as a relative contraindication for TAR in the past, but these patients similarly have achieved significant improvements in outcomes in more recent literature (46,47). In current literature, the evidence assessing risk of complications and failures in obese patients is conflicting. While one report cited an increased failure risk in obese patients (48), other studies have found minimal differences in incidences of complications, infection, or failure (46,47,49). In spite of conflicting evidence, there is a consensus that obese patients can achieve significant improvements in pain and functional outcomes following TAR, though they may have lower functional scores compared to their non-obese counterparts.
Diabetes persists as a relative contraindication to TAR, especially in the setting of uncontrolled diabetes (A1C >7.0%) (50). Though diabetic patients can still achieve improvements in pain and functional outcomes, there is significant evidence demonstrating an increased risk of complications and delayed wound healing for diabetic TAR patients (50-52).
Absolute contraindications for TAR include active infection, excessive loss of bone stock, neuropathic or Charcot arthropathy, inadequate soft tissue envelope around the ankle, confirmed metal allergy, and vascular deficiency of the limb. In addition, surgeons should use discretion in patient selection for TAR beyond these absolute characteristics and develop their operative plan based on their patient’s characteristics, relative risks, and functional demands. Surgeons may use magnetic resonance imaging (MRI), computed tomography (CT), or weightbearing CT (WBCT) to better characterize bone quality, deformity, presence of periarticular cysts, and associated soft tissue pathology to finalize their surgical plan (53).
Techniques
An anterior approach is the most used approach for majority of TAR implants; there is one implant that employs a lateral approach for its design, and a posterior approach for TAR has been described in literature (54). A midline incision centered over the ankle joint and the interval between the tibialis anterior and extensor hallucis longus is utilized. The superficial peroneal nerve is identified and retracted throughout the case. The extensor retinaculum is incised with care for repair at the end of the case. The anterior tibial neurovascular bundle is encountered and retracted laterally. The capsule is then incised and elevated off the joint. Adequate exposure of the ankle joint should allow for complete visualization of the medial and lateral gutters of the ankle.
The operative sequences are specific to each implant, but generally include the following steps: (I) placement of an extramedullary alignment guide to facilitate cuts; (II) provisional pinning of a cutting block to the ankle; (III) bony cuts of the tibial and talus; (IV) trial component placement; and (V) placement of final components (Figure 1). Intraoperative fluoroscopy is critical through the process. In addition to placement of components, the other driving operative goal of TAR is to appropriately align the ankle joint and the foot underneath the ankle. Adequate alignment is achieved through a combination of intraarticular deformity correction and external procedures, which all assist in balancing of the ankle and foot (Table 2).
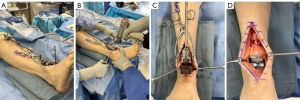
Table 2
Procedures for varus deformity | Procedures for valgus deformity |
---|---|
Deltoid ligament release | Deltoid/spring ligament reconstruction |
Lateral ligament repair | Lateral ligament repair |
Achilles lengthening | Achilles lengthening |
Gastrocnemius recession | Gastrocnemius recession |
Lateralizing calcaneal osteotomy | Medializing calcaneal osteotomy |
1st metatarsal dorsiflexion osteotomy | Fibular lengthening osteotomy |
Medial release | Medial column stabilization |
• Talonavicular joint capsule release | • Cotton osteotomy |
• Posterior tibial tendon release | • 1st tarsometatarsal fusion |
• Naviculocuneiform fusion | |
Peroneus longus to brevis transfer | Peroneus longus to brevis transfer |
Posterior tibial tendon to peroneus brevis | Hindfoot fusion for rigid deformity |
Hindfoot fusion for rigid deformity | |
Talonavicular fusion | |
Naviculocuneiform fusion |
TAA, total ankle arthroplasty.
Final radiographs are taken to ensure adequate implant contact to bone and mechanical alignment. The wound is closed in layers, with meticulous attention to extensor retinacular repair to reduce the risk of bowstringing from the tibialis anterior tendon, which can threaten the anterior skin.
Though the anterior approach to the ankle is most relevant for many implants in TAR literature, there is one implant (Trabecular Metal Total Ankle System) that employs a transfibular approach. In this case, an incision is made overlying the lateral malleolus, and the anterior talofibular ligament is identified and sectioned. After the fibula and anterior tibia are exposed, an oblique fibular osteotomy is performed approximately 1 cm proximal to the tibiotalar joint line. Following the fibular osteotomy, the ankle is placed into an external frame and cutting guides are placed. After TAR implantation, the fibula is anatomically reduced and fixed using a screw or plate, and the anterior talofibular ligament is repaired.
Postoperative recovery protocol for patients following TAR can vary by institution, especially in regard to the patient’s weightbearing timeline. Generally following TAR, the patient is immobilized in a short-leg plaster splint and is non-weightbearing for the first four to six weeks. Following discharge, patients are put on a course of pain medication consisting of acetaminophen, non-steroidal anti-inflammatory drugs (NSAIDs), and a limited dose of oral opioids; medication to prevent venous thromboembolism may be administered per the hospitalists or medical doctors’ discretion. At the two-week postoperative visit, the splint and sutures are removed, and the patient is transitioned to a controlled ankle motion (CAM) boot. At the four- to six-week postoperative visit, postoperative radiographs are obtained, and the patient begins following a progressive weightbearing protocol. At the 8- to 10-week postoperative visit, two-month radiographs are obtained, and if the patient is fully-weightbearing, they can now switch out of the CAM boot to a supportive sneaker. Follow-up visits and radiographs will continue at four months, seven months, and one year postoperatively, then are performed annually during subsequent follow-up visits.
Outcomes
In the modern era, TAR is associated with excellent outcomes in terms of pain relief and function. The past two decades of TAR research has demonstrated significant improvements in clinical and functional outcomes, such as increased implant survivorship, decreased complication and reoperation rates, and improvements in functional scores and perceived pain relief. As a result of these considerable advancements, TAR has become increasingly popular as a treatment option for end-stage arthritis and has raised debate about its merits over the current gold-standard treatment option, ankle arthrodesis.
Outcomes versus arthrodesis
Compared to arthrodesis, TAR has been demonstrated to have similar survivorship, better pain reduction, and decreased reoperation rates (55). Several studies have been published comparing outcomes of individual TAR implants (HINTEGRA, Salto Talaris, STAR, and INBONE) to ankle arthrodesis, which corroborate findings of survivorship and clinical improvements (55-58). Evolution of TAR in the past decade has further demonstrated its advantages over arthrodesis; third-generation TAR implants have significantly lower rates of aseptic loosening compared to rates of nonunion in arthrodesis (59). Furthermore, TAR patients report greater improvements in satisfaction scores and better fulfillment of preoperative expectations versus arthrodesis (60). Functional outcomes following TAR demonstrate superior results to arthrodesis in regards to gait, range of motion, and functional ability. Multiple studies assessing comparative gait analysis between TAR and arthrodesis have demonstrated more symmetrical gait timing, recovered bilateral gait, and restored ground reaction force transmission in TAR that better replicated that of a healthy control (61-63). Further, TAR patients have greater total arc of movement compared to arthrodesis, and subsequently less compensatory movement in adjacent joints, allowing for greater preservation of adjacent joints from degenerative changes (64). Improved performance ascending and descending stairs, and better negotiation of uneven surfaces have additionally been correlated with TAR (65,66). As modern, fourth-generation TAR implant reach 5-year and 10-year milestones, further studies are necessary to report upon outcomes and to compare with ankle arthrodesis. Though TAR outcomes are promising, there are inherent trade-offs between the two procedures, and patient selection remains an important consideration prior to surgical intervention.
Clinical and functional outcomes
Patients who undergo TAR experience significant improvements in pain and physical function. Assessments of patient-reported outcome have consistently confirmed significant improvements in pain reduction and quality of life following TAR (43,67,68). In addition, patient satisfaction following TAR is high, with rates ranging between 80–97%, but typically exceeding 90% (69). Functional outcomes for TAR patients have been assessed through various metrics, including patient-reported outcome scores, clinical assessment of range of motion, gait analysis, and participation in sports prior and following TAR. Postoperatively, range of motion increases on average by 5–10°, for a total arc of motion ranging between 34–40° (64,70). Patient-reported outcomes of functional abilities consistently demonstrate significant improvements postoperatively (69); further, patients with worse preoperative function have shown greater improvements in outcome scores compared to those with higher preoperative function scores (71). Moreover, patients have demonstrated a 20% increased participation in sports activities following TAR, though usually these activities were “low-impact”, such as swimming, golf, and cycling (72,73). Though high-impact sports are not advised to preserve the longevity of TAR implants, patients can still expect to achieve marked improvement in their daily function and abilities in low-impact sports.
Implant survivorship
Current survivorship for TAR implants ranges from 70% to 98% at 3–6 years and 80% to 95% at 8–12 years postoperatively (Table 3) (69). Comprehensive meta-analyses reporting upon outcomes for TAR are sparse; the most recent of which calculated an adjusted survivorship of 90% at 5-year across 1,105 TARs (13). These analyses, however, are limited to second and third generation implants, and do not report the outcomes of many modern implants currently used by surgeons. Fourth-generation implants such as the Vantage, INFINITY, and Cadence are widely used by current TAR surgeons, but their mid- to long-term reports on outcomes are limited by their relative novelty. Early reports on outcomes for these novel implants are promising, with survivorship ranging between 93.7% to 100% at 2 years (20,32,33,84). However, it is important to note that most TAR procedures are carried out in high-volume hospitals in metropolitan areas in the United States, and tend to be performed by surgeons with high volumes of TAR expertise (85,86). In spite of this, low volume hospitals for TAR have also been shown to achieve improved outcomes and good survivorship (87).
Table 3
Implant | Study | No. of implants | Follow-up (years), median (IQR) | Survivorship | Reoperation rate |
---|---|---|---|---|---|
Agility | Knecht, 2004 (74) | 132 | 9.0 (7.0 to 16) | 89.00% | Not recorded |
Raikin, 2017 (22) | 115 | 9.1 (4.0 to 14) | 78.20% | Not recorded | |
Cadence | Fram, 2022 (75) | 58 | Minimum 2 | 94.80% | 20.70% |
Kim, 2023 (30) | 48 | 2.8 (2.0 to 4.2) | 93.80% | 6.30% | |
HINTEGRA | Yang, 2019 (76) | 210 | 6.4 (2.0 to 13.4) | 91.70% | 9.00% |
Yoon, 2022 (77) | 151 | 11.3 (10 to 17) | 93.50% | 22.50% | |
INBONE I | Adams, 2014 (17) | 194 | 3.7 (2.2 to 5.5) | 89.00% | 25% |
Harston, 2017 (78) | 149 | 5.9 (4.0 to 9.4) | 90.60% | 13.40% | |
INBONE II | Lewis, 2015 (79) | 56 | 2.1 (1.3 to 2.9) | 97.40% | 15.90% |
Gagne, 2022 (20) | 51 | 6.4 (5.0 to 9.0) | 98.00% | 7.80% | |
INFINITY | Saito, 2018 (33) | 64 | 2.0 (1.5 to 3.3) | 95.30% | 17.10% |
Cody, 2019 (80) | 159 | 1.6 (1.0 to 3.1) | 90.00% | Not recorded | |
Salto Talaris | Stewart, 2017 (23) | 106 | 6.8 (5.0 to 9.6) | 95.80% | 19.00% |
Day, 2020 (22) | 85 | 7.1 (5.0 to 12) | 97.60% | 21.20% | |
STAR | Wood, 2003 (81) | 143 | 7.3 (5.0 to 13.0) | 80.30% | Not recorded |
Clough, 2019 (82) | 87 | 15.8 (11.1 to 24.5) | 76.16% | Not recorded | |
Zimmer | Barg, 2018 (83) | 55 | 2.2 (1.9 to 2.6) | 93.00% | 18.20% |
Maccario, 2022 (29) | 86 | 5.4 (5.0 to 7.5) | 97.70% | Not recorded |
IQR, interquartile range.
Revisions and risk factors
In the case of TAR failure, revision options include revision TAR, tibiotalar arthrodesis, and in more severe cases, tibiotalocalcaneal (TTC) fusion or below knee amputation (BKA). The most common indications for revision in TAR are due to infection, aseptic loosening, and subsidence. Revision is classically defined as implant failures necessitating a return to the operating room for exchange or removal of the tibial and/or talar implant (17,88), whereas reoperations are characterized as all other returns to the OR that preserve the metallic components. Outcomes for revision TARs demonstrate relatively good survivorship ranging between 80% to 97%, with improving survival rates in recent years following the introduction of robust, stemmed implants that account for loss of bone stock (36,89,90). Revision TAR has been found to preserve ankle range of motion and protect the adjacent joints from compensatory load, offering greater function compared to revision to ankle arthrodesis (91,92). Moreover, patient-reported outcome scores following revision TAR showed greater improvements compared to ankle arthrodesis, yet failed to reach the threshold of improvement observed with primary TAR (89,93). Tibiotalar arthrodesis following failed primary TAR has also had satisfactory outcomes and survivorship. In one meta-analysis of 193 patients with failed TARs converted to ankle arthrodesis, 84% had successful fusion; though these rates ranged from 50% to 100% when subcategorized by mode of fusion (94-96). Both revision TAR and ankle arthrodesis are viable treatment options following failed TAR, though differences in function, pain, and survivorship do exist between the two procedures.
Determining the patient factors that may contribute to implant failure is an important area of research in TAR. Recent assessments of patient demographics and TAR outcomes have identified prior ankle fusion and ipsilateral hindfoot fusion as risk factors for failure, likely due to the increased stresses placed on the foot and implant (18,97,98). Other theorized risk factors contributing to failure include activity level, body mass index (BMI), preoperative diagnosis of inflammatory arthritis, and severe ankle deformity, but reports on their associations are varied (18). Younger, more active patients have been thought to be at greater risk for failure due to greater estimated stress and longer implant lifetime, but large cohort analysis of TAR outcomes in younger patients did not identify any increased risk (18,38). Similarly, high BMI has also been identified as a potential risk factor for failure, but this association was not identified in recent outcome assessments (18,47). Preoperative diagnosis of inflammatory arthritis has had concerns for impact on TAR survivorship due to its correlation with poor bone stock, increased inflammatory response, and confounding influence of immunomodulatory medication (99,100). However, current analyses have reported similar outcomes in terms of survivorship, complications, and reoperations between patients with and without inflammatory arthritis (18,100). Finally, patients with severe varus or valgus deformity have demonstrated comparable results in recent studies, so long as the deformity is corrected intraoperatively (18,44,45). Further studies with longer follow-up are necessary to corroborate with the current literature about risk factors in TAR.
Survivorship, pain scores, and clinical outcomes have continued to improve in newer generations of implants, while complications and reoperation rates have decreased. However, despite the trends in improvements for TAR, outcomes studies for modern implants are inherently limited by the low-quality of evidence and insufficiency of long-term studies.
Complications
Complications associated with TAR include delayed wound healing, infection, periprosthetic fracture, impingement, and periprosthetic lucency and cysts. Treatment of these complications can involve nonoperative intervention, reoperation, revision, or conversion to ankle fusion/amputation based on case severity. Categorization of TAR complications based on their associated clinical outcomes was first proposed by Glazebrook et al., and established three categories: high-grade, medium-grade, and low-grade (101). This categorization can help guide surgeon decision making and intervention plans at the onset of complications.
Wound-healing complications are a prominent concern in the early postoperative period and can jeopardize the integrity of the implant. Wound-healing complications may be minor and have complete resolution of symptoms following treatment with local wound care or oral antibiotics. More severe wound issues may require a return to the operating room for more aggressive intervention, such as irrigation and debridement, vacuum-assisted closure, or flap coverage. Longer operative time and longer tourniquet time have been associated with higher rates of wound complications, as well as patients with a diagnosis of primary osteoarthritis, history of diabetes, and history of smoking (102-104).
Periprosthetic joint infection (PJI) following TAR has a reported incidence of 0% to 6.7% in current literature (13,105,106). PJI can be divided into two categories: acute PJI and chronic PJI. Acute PJI is characterized as infections either occurring in the early postoperative period or occurring with sudden onset in a patient previously doing well, with symptom duration below 4 weeks (106,107). Acute infections are typically treated with debridement, antibiotics, and implant retention (DAIR) with polyethylene exchange. The long-term outcomes following DAIR have been suboptimal, with recent reports citing a failure rate of 54% and high rates of reinfection (107). However, it has been determined that earlier surgical intervention following the onset of symptoms is directly correlated success rate of treatment with DAIR (107).
Chronic infections require a two-stage revision, consisting first of complete removal of all implants and insertion of an antibiotic cement spacer, with a course of intravenous antibiotics for at least six weeks. Depending on the patient’s condition, status of infection, and available bone stock, the second stage of the revision may involve reimplantation of a revision TAR implant, conversion to arthrodesis, permanent retention of the cement spacer, or below-knee amputation. Currently, reports detailing outcomes following 2-stage revision for chronic PJI are limited. In one single-center series of ankle PJI in 34 patients, the 10 patients treated with 2-stage revision had a reinfection rate of 0% (105). Similarly, a meta-analysis of 105 cases of ankle PJI across 6 studies reported a 0% reinfection rate in the 22 patients treated with 2-stage revision (108). Larger cohort studies are necessary to draw definitive conclusions on outcomes following ankle PJI, but current literature indicates 2-stage revision as an effective intervention for eradicating infection following TAR PJI.
Intraoperatively, the most common complication during TAR is peri-prosthetic fracture, typically medial or lateral malleolar fracture (109). Medial malleolar fractures are most frequent, with an incidence rate of 6%, while lateral malleolar fractures have a rate of 1% (109); however, the occurrence of intraoperative fractures has been shown to decrease with increased surgeon experience (110,111). Intraoperative fractures should be treated with open reduction internal fixation, though patients can achieve optimal outcomes without fixation if fracture is nondisplaced (112). In cases of medial malleolar thinning during bony resection at the time of index TAR, prophylactic fixation is recommended. Postoperatively, the incidence of fractures is between 2% to 4%, primarily around the medial malleolus, followed by the tibial diaphysis, talus, and fibula (113,114). Operative management is recommended for all instances of postoperative periprosthetic fracture, as nonoperative treatment has been demonstrated as a predictor of treatment failure in TAR (114). Periprosthetic fractures with implant stability can be successfully treated with open reduction and internal fixation; fracture with an unstable implant should be indicated for revision TAR or conversion to arthrodesis (113,114).
Symptomatic bony impingement is the most common indication for reoperation following TAR, and onset of impingement is largely correlated to inadequate gutter debridement at the time of the index procedure (22,115). The rate of reoperation for symptomatic impingement currently cited in literature ranges from 7% to 18% (18,115,116). In a single-center study for incidence of symptomatic impingement in 489 TARs, it was determined that incidence dropped from 18% to 2% if the patients underwent gutter debridement at the time of the index TAR (116). Other factors associated with impingement include implant malposition or subsidence, persistent malalignment, overstuffing of the ankle joint, heterotopic ossification, and shifting of the polyethylene insert (116). Gutter impingement is typically treated with open or arthroscopic gutter debridement, however, symptomatic impingement due to implant malposition, subsidence, or persistent malalignment may require further surgical intervention, including polyethylene exchange, revision of the metallic components, or deformity correction.
Aseptic loosening and subsidence continue to be the most common causes of implant failure in TAR (93,101,117), though the incidence of loosening and/or subsidence varies in the literature. Implant loosening and subsidence can be attributed to several factors: progressive osteolysis, poor bone quality, poor initial fixation, implant malposition, and increased contact pressure (118-120). Additionally, biomechanical models of implant fixation demonstrated that implant design may affect implant-bone micromotion and subsequent osseous integration (121), though further studies are warranted to investigate this association across implant types. Symptomatic aseptic loosening and/or subsidence typically is treated with revision of the tibial and/or talar component if sufficient bone stock is available. Otherwise, if revision is not feasible—due to insufficient bone stock, severe component subsidence, or insufficient soft tissue envelope—arthrodesis is a viable alternative.
Radiographic abnormalities, such as lucencies and peri-prosthetic cysts, are common findings in postoperative radiographs. The development of radiographic lucencies and osteolytic cysts has been associated with several potential factors, including implant micromotion, implant positioning, synovial fluid pressure, and immunologic response instigated by polyethylene insert wear or by bony necrosis (122-125). Though the association of many of these factors with osteolysis has been well described in hip and knee literature (123,125,126). further clinical studies are necessary to link to TARs. Although peri-implant lucency can be observed in around 30% of ankles following TAR, lucency does not always require surgical intervention (127,128). Radiolucencies in postoperative radiographs should be monitored for progression and correlated to clinical assessment to determine if surgical intervention is warranted (124,125). Peri-prosthetic cysts are less common than radiolucencies, but prevalent nonetheless. Peri-prosthetic cysts are typically evaluated with thorough clinical examination and radiographic imaging to assess symptoms, cyst size and location, progression, and imminent threat to implant integrity; patients with associated pain should also be worked up for infection (129,130). Incidences of cysts with significant progression or symptoms of pain can be treated with curettage and bone grafting, or with revision TAR or arthrodesis in cases of severe bone loss or implant subsidence (129). The intervention of symptomatic peri-prosthetic cysts with curettage and grafting has demonstrated a success rate of 90% (131). The optimal treatment for peri-prosthetic cysts and radiolucencies, however, has yet to be determined; intervention options are strongly dependent upon patient symptoms, cyst or radiolucency size and location, and integrity of implant and surrounding bone stock.
Future
With the mounting popularity of TAR over the past decade, there is considerable interest to continue to innovate, refine, and improve. The evolution of TAR over its 50 years of existence has provided tremendous insight for implant design, surgical technique and planning, and overall improvements of outcomes. Though the most recent fourth generation of TAR implants have succeeded in optimizing clinical, radiographic, and functional outcomes, there still exists areas of further development in TAR.
While demand for TAR has increased across the United States, the number of surgeons who regularly perform TAR procedures is fairly limited (85,86). TAR is associated with a steep learning curve that influences outcomes, as well as surgical time and risk of intraoperative fracture (132). This barrier has led to the development of patient-specific instrumentation (PSI) to assist in minimizing the learning curve and improving outcomes for naïve TAR surgeons. Results of PSI usage in TAR have demonstrated reduced operative time, accurate presurgical plans, and accurate joint alignment (133-135). Recently, two additional implants have introduced their own PSI systems, suggesting that PSI may become an established tool for ankle replacement surgeons. However, there are still some limitations that prevail in the current PSI technology, including inaccuracies in tibial sizing and limitations in presurgical planning for cases with severe deformity. Although the paucity of current literature assessing PSI in TAR makes it difficult to draw finite conclusions, initial evidence demonstrates promising results for PSI as a reliable and accurate tool for TAR.
Successful outcomes achieved in TAR has prompted interest in expanded anatomy-replicating implants, such as with the total talus replacement (TTR). TTR was designed as an alternative treatment option for patients with severe talar AVN, talar dome collapse, or significant loss of talar bone stock, when used in adjunct with total ankle arthroplasty (TAA) (136). Although the first report of a synthetic talar prosthesis was performed in 1997, for a series of 16 patients with AVN, significant attention towards TTR only recently developed in the past ten years in parallel to the growing prevalence of three-dimensional (3D) printing (137). Current literature reporting TTR outcomes is scarce, typically limited to case reports and anecdotal findings, which makes it difficult to determine the feasibility or relative success of the procedure. In a meta-analysis of outcomes in 196 TTR ankles, results reported a relatively low incidence of revisions (10 ankles), improvement in dorsiflexion, and improved patient-reported outcomes at four-year follow-up (138). However, there are several challenges that impact the feasibility of TTR, including the development of adjacent joint arthritis, prosthesis instability, and PJI (136,139). Moreover, following TTR failure, salvage options are limited and technically demanding. Due to the short-term follow-up and small sample sizes featured in TTR literature, definitive conclusions on survivorship, outcomes, and complications in the long term are impossible. Currently, TTR shows promise as a treatment option for patients with severe talar pathology, but further studies with adequate follow-up are necessary to validate current findings.
Conclusions
TAR has undergone marked innovation in the past 50 years, and has continued to grow in popularity in the past decade. The third and fourth generations of TAR implants currently circulating the market have implemented improvements in bone fixation, mechanical alignment, and soft tissue balance that have contributed to increases in survivorship, functional outcomes, and pain resolution. The continual refinement of prosthesis design and surgical technique have allowed indications for TAR to expand, and complications associated with the procedure to decrease. Current outcomes for TAR demonstrate its merit as a viable alternative treatment option to ankle arthrodesis in the setting of end-stage ankle osteoarthritis. Future innovation in the field of TAR looks to expand upon the implementation of PSI and revision TAR systems to further improve outcomes and guide surgical approach.
Acknowledgments
Funding: None.
Footnote
Provenance and Peer Review: This article was commissioned by the Guest Editor (Albert T. Anastasio) for the series “Foot and Ankle Surgery” published in Annals of Translational Medicine. The article has undergone external peer review.
Reporting Checklist: The authors have completed the Narrative Review reporting checklist. Available at https://atm.amegroups.com/article/view/10.21037/atm-23-1569/rc
Peer Review File: Available at https://atm.amegroups.com/article/view/10.21037/atm-23-1569/prf
Conflicts of Interest: All authors have completed the ICMJE uniform disclosure form (available at https://atm.amegroups.com/article/view/10.21037/atm-23-1569/coif). The series “Foot and Ankle Surgery” was commissioned by the editorial office without any funding or sponsorship. C.D. reports royalties received from Wolters Kluwer Heakth – Lippincott Williams & Wilkins, consulting fees from Exactech, Inc, In2Bones, Medshape, RTI Surgical, payments (for presentation) from Exactech, Inc, and serves as a board member for American Orthopaedic Foot and Ankle Society. The authors have no other conflicts of interest to declare.
Ethical Statement: The authors are accountable for all aspects of the work in ensuring that questions related to the accuracy or integrity of any part of the work are appropriately investigated and resolved.
Open Access Statement: This is an Open Access article distributed in accordance with the Creative Commons Attribution-NonCommercial-NoDerivs 4.0 International License (CC BY-NC-ND 4.0), which permits the non-commercial replication and distribution of the article with the strict proviso that no changes or edits are made and the original work is properly cited (including links to both the formal publication through the relevant DOI and the license). See: https://creativecommons.org/licenses/by-nc-nd/4.0/.
References
- Shah JA, Schwartz AM, Farley KX, Mahmoud K, Attia AK, Labib S, Kadakia RJ. Projections and Epidemiology of Total Ankle and Revision Total Ankle Arthroplasty in the United States to 2030. Foot Ankle Spec 2022; Epub ahead of print. [Crossref] [PubMed]
- Morgan CD, Henke JA, Bailey RW, et al. Long-term results of tibiotalar arthrodesis. J Bone Joint Surg Am 1985;67:546-50. [Crossref] [PubMed]
- Mann RA, Rongstad KM. Arthrodesis of the ankle: a critical analysis. Foot Ankle Int 1998;19:3-9. [Crossref] [PubMed]
- Johnson EW Jr, Boseker EH. Arthrodesis of the ankle. Arch Surg 1968;97:766-73. [Crossref] [PubMed]
- Mazur JM, Schwartz E, Simon SR. Ankle arthrodesis. Long-term follow-up with gait analysis. J Bone Joint Surg Am 1979;61:964-75. [Crossref] [PubMed]
- Wu WL, Su FC, Cheng YM, et al. Gait analysis after ankle arthrodesis. Gait Posture 2000;11:54-61. [Crossref] [PubMed]
- Coester LM, Saltzman CL, Leupold J, et al. Long-term results following ankle arthrodesis for post-traumatic arthritis. J Bone Joint Surg Am 2001;83:219-28. [Crossref] [PubMed]
- Bolton-Maggs BG, Sudlow RA, Freeman MA. Total ankle arthroplasty. A long-term review of the London Hospital experience. J Bone Joint Surg Br 1985;67:785-90. [Crossref] [PubMed]
- Pyevich MT, Saltzman CL, Callaghan JJ, et al. Total ankle arthroplasty: a unique design. Two to twelve-year follow-up. J Bone Joint Surg Am 1998;80:1410-20. [Crossref] [PubMed]
- Gill LH. Challenges in total ankle arthroplasty. Foot Ankle Int 2004;25:195-207. [Crossref] [PubMed]
- Easley ME, Vertullo CJ, Urban WC, et al. Total ankle arthroplasty. J Am Acad Orthop Surg 2002;10:157-67. [Crossref] [PubMed]
- Lewis G. Biomechanics of and research challenges in uncemented total ankle replacement. Clin Orthop Relat Res 2004;89-97. [Crossref] [PubMed]
- Gougoulias N, Khanna A, Maffulli N. How successful are current ankle replacements?: a systematic review of the literature. Clin Orthop Relat Res 2010;468:199-208. [Crossref] [PubMed]
- Cracchiolo A 3rd, Deorio JK. Design features of current total ankle replacements: implants and instrumentation. J Am Acad Orthop Surg 2008;16:530-40. [Crossref] [PubMed]
- Gross CE, Palanca AA, DeOrio JK. Design Rationale for Total Ankle Arthroplasty Systems: An Update. J Am Acad Orthop Surg 2018;26:353-9. [Crossref] [PubMed]
- DeOrio JK. INBONE Total Ankle Arthroplasty. Semin Arthroplasty 2010;21:288-94. [Crossref]
- Adams SB Jr, Demetracopoulos CA, Queen RM, et al. Early to mid-term results of fixed-bearing total ankle arthroplasty with a modular intramedullary tibial component. J Bone Joint Surg Am 2014;96:1983-9. [Crossref] [PubMed]
- Cody EA, Bejarano-Pineda L, Lachman JR, et al. Risk Factors for Failure of Total Ankle Arthroplasty With a Minimum Five Years of Follow-up. Foot Ankle Int 2019;40:249-58. [Crossref] [PubMed]
- Tennant JN, Rungprai C, Pizzimenti MA, et al. Risks to the blood supply of the talus with four methods of total ankle arthroplasty: a cadaveric injection study. J Bone Joint Surg Am 2014;96:395-402. [Crossref] [PubMed]
- Gagne OJ, Day J, Kim J, et al. Midterm Survivorship of the INBONE II Total Ankle Arthroplasty. Foot Ankle Int 2022;43:628-36. [Crossref] [PubMed]
- Hofmann KJ, Shabin ZM, Ferkel E, et al. Salto Talaris Total Ankle Arthroplasty: Clinical Results at a Mean of 5.2 Years in 78 Patients Treated by a Single Surgeon. J Bone Joint Surg Am 2016;98:2036-46. [Crossref] [PubMed]
- Day J, Kim J, O'Malley MJ, et al. Radiographic and Clinical Outcomes of the Salto Talaris Total Ankle Arthroplasty. Foot Ankle Int 2020;41:1519-28. [Crossref] [PubMed]
- Stewart MG, Green CL, Adams SB Jr, et al. Midterm Results of the Salto Talaris Total Ankle Arthroplasty. Foot Ankle Int 2017;38:1215-21. [Crossref] [PubMed]
- Palanca A, Mann RA, Mann JA, et al. Scandinavian Total Ankle Replacement: 15-Year Follow-up. Foot Ankle Int 2018;39:135-42. [Crossref] [PubMed]
- Jastifer JR, Coughlin MJ. Long-term follow-up of mobile bearing total ankle arthroplasty in the United States. Foot Ankle Int 2015;36:143-50. [Crossref] [PubMed]
- Mann JA, Mann RA, Horton E. STAR™ ankle: long-term results. Foot Ankle Int 2011;32:S473-84. [Crossref] [PubMed]
- Kvarda P, Peterhans US, Susdorf R, et al. Long-Term Survival of HINTEGRA Total Ankle Replacement in 683 Patients: A Concise 20-Year Follow-up of a Previous Report. J Bone Joint Surg Am 2022;104:881-8. [Crossref] [PubMed]
- Zafar MJ, Kallemose T, Benyahia M, et al. 12-year survival analysis of 322 Hintegra total ankle arthroplasties from an independent center. Acta Orthop 2020;91:444-9. [Crossref] [PubMed]
- Maccario C, Paoli T, Romano F, et al. Transfibular total ankle arthroplasty: a new reliable procedure at five-year follow-up. Bone Joint J 2022;104-B:472-8. [Crossref] [PubMed]
- Kim J, Gagne OJ, Rajan L, et al. Clinical Outcomes of the Lateral Trabecular Metal Total Ankle Replacement at a 5-Year Minimum Follow-up. Foot Ankle Spec 2023;16:288-99. [Crossref] [PubMed]
- DeVries JG, Derksen TA, Scharer BM, et al. Perioperative Complications and Initial Alignment of Lateral Approach Total Ankle Arthroplasty. J Foot Ankle Surg 2017;56:996-1000. [Crossref] [PubMed]
- Henry JK, Rajan L, Fuller R, et al. Early Revisions, Reoperation, and Survivorship of the Exactech Vantage Fixed-Bearing Total Ankle Arthroplasty Implant. Foot Ankle Orthop 2022;7:2473011421S00686.
- Saito GH, Sanders AE, de Cesar Netto C, et al. Short-Term Complications, Reoperations, and Radiographic Outcomes of a New Fixed-Bearing Total Ankle Arthroplasty. Foot Ankle Int 2018;39:787-94. [Crossref] [PubMed]
- Kim J, Rajan L, Bitar R, et al. Early Radiographic and Clinical Outcomes of a Novel, Fixed-Bearing Fourth-Generation Total Ankle Replacement System. Foot Ankle Int 2022;43:1424-33. [Crossref] [PubMed]
- Spirt AA, Assal M, Hansen ST Jr. Complications and failure after total ankle arthroplasty. J Bone Joint Surg Am 2004;86:1172-8. [Crossref] [PubMed]
- Jamjoom BA, Siddiqui BM, Salem H, et al. Clinical and Radiographic Outcomes of Revision Total Ankle Arthroplasty Using the INBONE II Prosthesis. J Bone Joint Surg Am 2022;104:1554-62. [Crossref] [PubMed]
- Valderrabano V, Horisberger M, Russell I, et al. Etiology of ankle osteoarthritis. Clin Orthop Relat Res 2009;467:1800-6. [Crossref] [PubMed]
- Kofoed H, Lundberg-Jensen A. Ankle arthroplasty in patients younger and older than 50 years: a prospective series with long-term follow-up. Foot Ankle Int 1999;20:501-6. [Crossref] [PubMed]
- Demetracopoulos CA, Adams SB Jr, Queen RM, et al. Effect of Age on Outcomes in Total Ankle Arthroplasty. Foot Ankle Int 2015;36:871-80. [Crossref] [PubMed]
- Henricson A, Nilsson JÅ, Carlsson A. 10-year survival of total ankle arthroplasties: a report on 780 cases from the Swedish Ankle Register. Acta Orthop 2011;82:655-9. [Crossref] [PubMed]
- Usuelli FG, Maccario C, D'Ambrosi R, et al. Age-Related Outcome of Mobile-Bearing Total Ankle Replacement. Orthopedics 2017;40:e567-73. [Crossref] [PubMed]
- Tenenbaum S, Bariteau J, Coleman S, et al. Functional and clinical outcomes of total ankle arthroplasty in elderly compared to younger patients. Foot Ankle Surg 2017;23:102-7. [Crossref] [PubMed]
- Trajkovski T, Pinsker E, Cadden A, et al. Outcomes of ankle arthroplasty with preoperative coronal-plane varus deformity of 10° or greater. J Bone Joint Surg Am 2013;95:1382-8. [Crossref] [PubMed]
- Lee GW, Wang SH, Lee KB. Comparison of Intermediate to Long-Term Outcomes of Total Ankle Arthroplasty in Ankles with Preoperative Varus, Valgus, and Neutral Alignment. J Bone Joint Surg Am 2018;100:835-42. [Crossref] [PubMed]
- Lee GW, Lee KB. Outcomes of Total Ankle Arthroplasty in Ankles with >20° of Coronal Plane Deformity. J Bone Joint Surg Am 2019;101:2203-11. [Crossref] [PubMed]
- Bouchard M, Amin A, Pinsker E, et al. The impact of obesity on the outcome of total ankle replacement. J Bone Joint Surg Am 2015;97:904-10. [Crossref] [PubMed]
- Gross CE, Lampley A, Green CL, et al. The Effect of Obesity on Functional Outcomes and Complications in Total Ankle Arthroplasty. Foot Ankle Int 2016;37:137-41. [Crossref] [PubMed]
- Schipper ON, Denduluri SK, Zhou Y, et al. Effect of Obesity on Total Ankle Arthroplasty Outcomes. Foot Ankle Int 2016;37:1-7. [Crossref] [PubMed]
- Sansosti LE, Van JC, Meyr AJ. Effect of Obesity on Total Ankle Arthroplasty: A Systematic Review of Postoperative Complications Requiring Surgical Revision. J Foot Ankle Surg 2018;57:353-6. [Crossref] [PubMed]
- Choi WJ, Lee JS, Lee M, et al. The impact of diabetes on the short- to mid-term outcome of total ankle replacement. Bone Joint J 2014;96-B:1674-80. [Crossref] [PubMed]
- Schipper ON, Jiang JJ, Chen L, et al. Effect of diabetes mellitus on perioperative complications and hospital outcomes after ankle arthrodesis and total ankle arthroplasty. Foot Ankle Int 2015;36:258-67. [Crossref] [PubMed]
- Patton D, Kiewiet N, Brage M. Infected total ankle arthroplasty: risk factors and treatment options. Foot Ankle Int 2015;36:626-34. [Crossref] [PubMed]
- de Cesar Netto C, Day J, Godoy-Santos AL, et al. The use of three-dimensional biometric Foot and Ankle Offset to predict additional realignment procedures in total ankle replacement. Foot Ankle Surg 2022;28:1029-34. [Crossref] [PubMed]
- Bibbo C. Posterior approach for total ankle arthroplasty. J Foot Ankle Surg 2013;52:132-5. [Crossref] [PubMed]
- Lawton CD, Butler BA, Dekker RG 2nd, et al. Total ankle arthroplasty versus ankle arthrodesis-a comparison of outcomes over the last decade. J Orthop Surg Res 2017;12:76. [Crossref] [PubMed]
- Shih CL, Chen SJ, Huang PJ. Clinical Outcomes of Total Ankle Arthroplasty Versus Ankle Arthrodesis for the Treatment of End-Stage Ankle Arthritis in the Last Decade: a Systematic Review and Meta-analysis. J Foot Ankle Surg 2020;59:1032-9. [Crossref] [PubMed]
- Fanelli D, Mercurio M, Castioni D, et al. End-stage ankle osteoarthritis: arthroplasty offers better quality of life than arthrodesis with similar complication and re-operation rates-an updated meta-analysis of comparative studies. Int Orthop 2021;45:2177-91. [Crossref] [PubMed]
- Saltzman CL, Kadoko RG, Suh JS. Treatment of isolated ankle osteoarthritis with arthrodesis or the total ankle replacement: a comparison of early outcomes. Clin Orthop Surg 2010;2:1-7. [Crossref] [PubMed]
- Lawton CD, Prescott A, Butler BA, et al. Modern total ankle arthroplasty versus ankle arthrodesis: A systematic review and meta-analysis. Orthop Rev (Pavia) 2020;12:8279. [Crossref] [PubMed]
- Younger AS, Wing KJ, Glazebrook M, et al. Patient expectation and satisfaction as measures of operative outcome in end-stage ankle arthritis: a prospective cohort study of total ankle replacement versus ankle fusion. Foot Ankle Int 2015;36:123-34. [Crossref] [PubMed]
- Chopra S, Rouhani H, Assal M, et al. Outcome of unilateral ankle arthrodesis and total ankle replacement in terms of bilateral gait mechanics. J Orthop Res 2014;32:377-84. [Crossref] [PubMed]
- Piriou P, Culpan P, Mullins M, et al. Ankle replacement versus arthrodesis: a comparative gait analysis study. Foot Ankle Int 2008;29:3-9. [Crossref] [PubMed]
- Singer S, Klejman S, Pinsker E, et al. Ankle arthroplasty and ankle arthrodesis: gait analysis compared with normal controls. J Bone Joint Surg Am 2013;95:e191(1-10).
- Pedowitz DI, Kane JM, Smith GM, et al. Total ankle arthroplasty versus ankle arthrodesis: a comparative analysis of arc of movement and functional outcomes. Bone Joint J 2016;98-B:634-40. [Crossref] [PubMed]
- Sanders AE, Kraszewski AP, Ellis SJ, et al. Differences in Gait and Stair Ascent After Total Ankle Arthroplasty and Ankle Arthrodesis. Foot Ankle Int 2021;42:347-55. [Crossref] [PubMed]
- Jastifer J, Coughlin MJ, Hirose C. Performance of total ankle arthroplasty and ankle arthrodesis on uneven surfaces, stairs, and inclines: a prospective study. Foot Ankle Int 2015;36:11-7. [Crossref] [PubMed]
- Nunley JA, Caputo AM, Easley ME, et al. Intermediate to long-term outcomes of the STAR Total Ankle Replacement: the patient perspective. J Bone Joint Surg Am 2012;94:43-8. [Crossref] [PubMed]
- Nodzo SR, Miladore MP, Kaplan NB, et al. Short to midterm clinical and radiographic outcomes of the Salto total ankle prosthesis. Foot Ankle Int 2014;35:22-9. [Crossref] [PubMed]
- Easley ME, Adams SB Jr, Hembree WC, et al. Results of total ankle arthroplasty. J Bone Joint Surg Am 2011;93:1455-68. [Crossref] [PubMed]
- Koivu H, Kohonen I, Mattila K, et al. Long-term Results of Scandinavian Total Ankle Replacement. Foot Ankle Int 2017;38:723-31. [Crossref] [PubMed]
- Frigg A, Germann U, Huber M, et al. Survival of the Scandinavian total ankle replacement (STAR): results of ten to nineteen years follow-up. Int Orthop 2017;41:2075-82. [Crossref] [PubMed]
- Johns WL, Sowers CB, Walley KC, et al. Return to Sports and Activity After Total Ankle Arthroplasty and Arthrodesis: A Systematic Review. Foot Ankle Int 2020;41:916-29. [Crossref] [PubMed]
- Bonnin MP, Laurent JR, Casillas M. Ankle function and sports activity after total ankle arthroplasty. Foot Ankle Int 2009;30:933-44. [Crossref] [PubMed]
- Knecht SI, Estin M, Callaghan JJ, et al. The Agility total ankle arthroplasty. Seven to sixteen-year follow-up. J Bone Joint Surg Am 2004;86:1161-71. [Crossref] [PubMed]
- Fram B, Corr DO, Rogero RG, et al. Short-Term Complications and Outcomes of the Cadence Total Ankle Arthroplasty. Foot Ankle Int 2022;43:371-7. [Crossref] [PubMed]
- Yang HY, Wang SH, Lee KB. The HINTEGRA total ankle arthroplasty: functional outcomes and implant survivorship in 210 osteoarthritic ankles at a mean of 6.4 years. Bone Joint J 2019;101-B:695-701. [Crossref] [PubMed]
- Yoon YK, Park KH, Park JH, et al. Long-Term Clinical Outcomes and Implant Survivorship of 151 Total Ankle Arthroplasties Using the HINTEGRA Prosthesis: A Minimum 10-Year Follow-up. J Bone Joint Surg Am 2022;104:1483-91. [Crossref] [PubMed]
- Harston A, Lazarides AL, Adams SB Jr, et al. Midterm Outcomes of a Fixed-Bearing Total Ankle Arthroplasty With Deformity Analysis. Foot Ankle Int 2017;38:1295-300. [Crossref] [PubMed]
- Lewis JS Jr, Green CL, Adams SB Jr, et al. Comparison of First- and Second-Generation Fixed-Bearing Total Ankle Arthroplasty Using a Modular Intramedullary Tibial Component. Foot Ankle Int 2015;36:881-90. [Crossref] [PubMed]
- Cody EA, Taylor MA, Nunley JA 2nd, et al. Increased Early Revision Rate With the INFINITY Total Ankle Prosthesis. Foot Ankle Int 2019;40:9-17. [Crossref] [PubMed]
- Wood PL, Deakin S. Total ankle replacement. The results in 200 ankles. J Bone Joint Surg Br 2003;85:334-41. [Crossref] [PubMed]
- Clough T, Bodo K, Majeed H, et al. Survivorship and long-term outcome of a consecutive series of 200 Scandinavian Total Ankle Replacement (STAR) implants. Bone Joint J 2019;101-B:47-54. [Crossref] [PubMed]
- Barg A, Bettin CC, Burstein AH, et al. Early Clinical and Radiographic Outcomes of Trabecular Metal Total Ankle Replacement Using a Transfibular Approach. J Bone Joint Surg Am 2018;100:505-15. [Crossref] [PubMed]
- Kim J, Rajan L, Fuller R, et al. Mid-term functional outcomes following reoperation after total ankle arthroplasty: A retrospective cohort study. Foot Ankle Surg 2022;28:1463-7. [Crossref] [PubMed]
- Stein B, Somerson J, Janney C, et al. Distribution of High-Volume Ankle Replacement Surgeons in United States Metropolitan Areas. Foot Ankle Spec 2022;15:127-35. [Crossref] [PubMed]
- Karzon AL, Kadakia RJ, Coleman MM, et al. The Rise of Total Ankle Arthroplasty Use: A Database Analysis Describing Case Volumes and Incidence Trends in the United States Between 2009 and 2019. Foot Ankle Int 2022;43:1501-10. [Crossref] [PubMed]
- Reuver JM, Dayerizadeh N, Burger B, et al. Total ankle replacement outcome in low volume centers: short-term followup. Foot Ankle Int 2010;31:1064-8. [Crossref] [PubMed]
- Schweitzer KM, Adams SB, Viens NA, et al. Early prospective clinical results of a modern fixed-bearing total ankle arthroplasty. J Bone Joint Surg Am 2013;95:1002-11. [Crossref] [PubMed]
- Egglestone A, Kakwani R, Aradhyula M, et al. Outcomes of revision surgery for failed total ankle replacement: revision arthroplasty versus arthrodesis. Int Orthop 2020;44:2727-34. [Crossref] [PubMed]
- Behrens SB, Irwin TA, Bemenderfer TB, et al. Clinical and Radiographic Outcomes of Revision Total Ankle Arthroplasty Using an Intramedullary-Referencing Implant. Foot Ankle Int 2020;41:1510-8. [Crossref] [PubMed]
- Hordyk PJ, Fuerbringer BA, Roukis TS. Sagittal Ankle and Midfoot Range of Motion Before and After Revision Total Ankle Replacement: A Retrospective Comparative Analysis. J Foot Ankle Surg 2018;57:521-6. [Crossref] [PubMed]
- Pfahl K, Röser A, Eder J, et al. Outcomes of Salvage Procedures for Failed Total Ankle Arthroplasty. Foot Ankle Int 2023;44:262-9. [Crossref] [PubMed]
- Lachman JR, Ramos JA, Adams SB, et al. Patient-Reported Outcomes Before and After Primary and Revision Total Ankle Arthroplasty. Foot Ankle Int 2019;40:34-41. [Crossref] [PubMed]
- Gross C, Erickson BJ, Adams SB, et al. Ankle arthrodesis after failed total ankle replacement: a systematic review of the literature. Foot Ankle Spec 2015;8:143-51. [Crossref] [PubMed]
- Doets HC, Zürcher AW. Salvage arthrodesis for failed total ankle arthroplasty. Acta Orthop 2010;81:142-7. [Crossref] [PubMed]
- Bullens P, de Waal Malefijt M, Louwerens JW. Conversion of failed ankle arthroplasty to an arthrodesis. Technique using an arthrodesis nail and a cage filled with morsellized bone graft. Foot Ankle Surg 2010;16:101-4. [Crossref] [PubMed]
- Pellegrini MJ, Schiff AP, Adams SB Jr, et al. Conversion of Tibiotalar Arthrodesis to Total Ankle Arthroplasty. J Bone Joint Surg Am 2015;97:2004-13. [Crossref] [PubMed]
- Hintermann B, Barg A, Knupp M, et al. Conversion of painful ankle arthrodesis to total ankle arthroplasty. J Bone Joint Surg Am 2009;91:850-8. [Crossref] [PubMed]
- Newton ST. An Artificial Ankle Joint. Clin Orthop Relat Res 1979;141-5. [PubMed]
- Mousavian A, Baradaran A, Schon LC, et al. Total Ankle Replacement Outcome in Patients With Inflammatory Versus Noninflammatory Arthritis: A Systematic Review and Meta-analysis. Foot Ankle Spec 2023;16:314-24. [Crossref] [PubMed]
- Glazebrook MA, Arsenault K, Dunbar M. Evidence-based classification of complications in total ankle arthroplasty. Foot Ankle Int 2009;30:945-9. [Crossref] [PubMed]
- Lampley A, Gross CE, Green CL, et al. Association of Cigarette Use and Complication Rates and Outcomes Following Total Ankle Arthroplasty. Foot Ankle Int 2016;37:1052-9. [Crossref] [PubMed]
- Raikin SM, Kane J, Ciminiello ME. Risk factors for incision-healing complications following total ankle arthroplasty. J Bone Joint Surg Am 2010;92:2150-5. [Crossref] [PubMed]
- Gross CE, Hamid KS, Green C, et al. Operative Wound Complications Following Total Ankle Arthroplasty. Foot Ankle Int 2017;38:360-6. [Crossref] [PubMed]
- Kessler B, Knupp M, Graber P, et al. The treatment and outcome of peri-prosthetic infection of the ankle: a single cohort-centre experience of 34 cases. Bone Joint J 2014;96-B:772-7. [Crossref] [PubMed]
- Myerson MS, Shariff R, Zonno AJ. The management of infection following total ankle replacement: demographics and treatment. Foot Ankle Int 2014;35:855-62. [Crossref] [PubMed]
- Lachman JR, Ramos JA, DeOrio JK, et al. Outcomes of Acute Hematogenous Periprosthetic Joint Infection in Total Ankle Arthroplasty Treated With Irrigation, Debridement, and Polyethylene Exchange. Foot Ankle Int 2018;39:1266-71. [Crossref] [PubMed]
- Kunutsor SK, Barrett MC, Whitehouse MR, et al. Clinical Effectiveness of Treatment Strategies for Prosthetic Joint Infection Following Total Ankle Replacement: A Systematic Review and Meta-analysis. J Foot Ankle Surg 2020;59:367-72. [Crossref] [PubMed]
- Zaidi R, Cro S, Gurusamy K, et al. The outcome of total ankle replacement: a systematic review and meta-analysis. Bone Joint J 2013;95-B:1500-7. [Crossref] [PubMed]
- Schimmel JJ, Walschot LH, Louwerens JW. Comparison of the short-term results of the first and last 50 Scandinavian total ankle replacements: assessment of the learning curve in a consecutive series. Foot Ankle Int 2014;35:326-33. [Crossref] [PubMed]
- Basques BA, Bitterman A, Campbell KJ, et al. Influence of Surgeon Volume on Inpatient Complications, Cost, and Length of Stay Following Total Ankle Arthroplasty. Foot Ankle Int 2016;37:1046-51. [Crossref] [PubMed]
- Tsitsilonis S, Schaser KD, Wichlas F, et al. Functional and radiological outcome of periprosthetic fractures of the ankle. Bone Joint J 2015;97-B:950-6. [Crossref] [PubMed]
- Manegold S, Haas NP, Tsitsilonis S, et al. Periprosthetic fractures in total ankle replacement: classification system and treatment algorithm. J Bone Joint Surg Am 2013;95:815-20, S1-3.
- Lazarides AL, Vovos TJ, Reddy GB, et al. Algorithm for Management of Periprosthetic Ankle Fractures. Foot Ankle Int 2019;40:615-21. [Crossref] [PubMed]
- Gross CE, Adams SB, Easley M, et al. Surgical Treatment of Bony and Soft-Tissue Impingement in Total Ankle Arthroplasty. Foot Ankle Spec 2017;10:37-42. [Crossref] [PubMed]
- Schuberth JM, Babu NS, Richey JM, et al. Gutter impingement after total ankle arthroplasty. Foot Ankle Int 2013;34:329-37. [Crossref] [PubMed]
- Undén A, Jehpsson L, Kamrad I, et al. Better implant survival with modern ankle prosthetic designs: 1,226 total ankle prostheses followed for up to 20 years in the Swedish Ankle Registry. Acta Orthop 2020;91:191-6. [Crossref] [PubMed]
- Rushing CJ, Zulauf E, Hyer CF, et al. Risk Factors for Early Failure of Fourth Generation Total Ankle Arthroplasty Prostheses. J Foot Ankle Surg 2021;60:312-7. [Crossref] [PubMed]
- Younger A, Veljkovic A. Current Update in Total Ankle Arthroplasty: Salvage of the Failed Total Ankle Arthroplasty with Anterior Translation of the Talus. Foot Ankle Clin 2017;22:301-9. [Crossref] [PubMed]
- Espinosa N, Walti M, Favre P, et al. Misalignment of total ankle components can induce high joint contact pressures. J Bone Joint Surg Am 2010;92:1179-87. [Crossref] [PubMed]
- Sopher RS, Amis AA, Calder JD, et al. Total ankle replacement design and positioning affect implant-bone micromotion and bone strains. Med Eng Phys 2017;42:80-90. [Crossref] [PubMed]
- Sundfeldt M, Carlsson LV, Johansson CB, et al. Aseptic loosening, not only a question of wear: a review of different theories. Acta Orthop 2006;77:177-97. [Crossref] [PubMed]
- Conditt MA, Thompson MT, Usrey MM, et al. Backside wear of polyethylene tibial inserts: mechanism and magnitude of material loss. J Bone Joint Surg Am 2005;87:326-31. [PubMed]
- Espinosa N, Klammer G, Wirth SH. Osteolysis in Total Ankle Replacement: How Does It Work? Foot Ankle Clin 2017;22:267-75. [Crossref] [PubMed]
- Goodman SB, Gallo J. Periprosthetic Osteolysis: Mechanisms, Prevention and Treatment. J Clin Med 2019;8:2091. [Crossref] [PubMed]
- Goodman SB. The effects of micromotion and particulate materials on tissue differentiation. Bone chamber studies in rabbits. Acta Orthop Scand Suppl 1994;258:1-43. [Crossref] [PubMed]
- Lee GW, Lee KB. Periprosthetic Osteolysis as a Risk Factor for Revision After Total Ankle Arthroplasty: A Single-Center Experience of 250 Consecutive Cases. J Bone Joint Surg Am 2022;104:1334-40. [Crossref] [PubMed]
- Yoon HS, Lee J, Choi WJ, et al. Periprosthetic osteolysis after total ankle arthroplasty. Foot Ankle Int 2014;35:14-21. [Crossref] [PubMed]
- Mehta N, Serino J, Hur ES, et al. Pathogenesis, Evaluation, and Management of Osteolysis Following Total Ankle Arthroplasty. Foot Ankle Int 2021;42:230-42. [Crossref] [PubMed]
- Hur ES, Mehta N, Lee S, et al. Management of Periprosthetic Bone Cysts After Total Ankle Arthroplasty. Orthop Clin North Am 2023;54:109-19. [Crossref] [PubMed]
- Gross CE, Huh J, Green C, et al. Outcomes of Bone Grafting of Bone Cysts After Total Ankle Arthroplasty. Foot Ankle Int 2016;37:157-64. [Crossref] [PubMed]
- Usuelli FG, Maccario C, Pantalone A, et al. Identifying the learning curve for total ankle replacement using a mobile bearing prosthesis. Foot Ankle Surg 2017;23:76-83. [Crossref] [PubMed]
- Escudero MI, Le V, Bemenderfer TB, et al. Total Ankle Arthroplasty Radiographic Alignment Comparison Between Patient-Specific Instrumentation and Standard Instrumentation. Foot Ankle Int 2021;42:851-8. [Crossref] [PubMed]
- Saito GH, Sanders AE, O'Malley MJ, et al. Accuracy of patient-specific instrumentation in total ankle arthroplasty: A comparative study. Foot Ankle Surg 2019;25:383-9. [Crossref] [PubMed]
- Daigre J, Berlet G, Van Dyke B, et al. Accuracy and Reproducibility Using Patient-Specific Instrumentation in Total Ankle Arthroplasty. Foot Ankle Int 2017;38:412-8. [Crossref] [PubMed]
- Kadakia RJ, Akoh CC, Chen J, et al. 3D Printed Total Talus Replacement for Avascular Necrosis of the Talus. Foot Ankle Int 2020;41:1529-36. [Crossref] [PubMed]
- Harnroongroj T, Vanadurongwan V. The Talar Body Prosthesis. JBJS 1997;79:1313-22. [Crossref] [PubMed]
- Johnson LG, Anastasio AT, Fletcher AN, et al. Outcomes following total talus replacement: A systematic review. Foot Ankle Surg 2022;28:1194-201. [Crossref] [PubMed]
- Iwamoto K, Yamamoto N, Saiga K, et al. Prosthetic joint infection after total talar replacement: An implant-retained case treated with combined continuous local antibiotic perfusion (CLAP). J Orthop Sci 2024;29:349-53. [Crossref] [PubMed]