Gut microbiota alterations in acute myocardial infarction: (diabetic) context is everything
Heart attack [myocardial infarction (MI)] prevalence is increasing worldwide but very little is known about the roles gut microbiota play in its pathogenesis and epidemiology (1,2). Similarly, the role of gut microbiota alterations in type 2 diabetes mellitus (T2DM), a significant co-morbidity in many patients with MI, is still under intense investigation (3). The recently published study by Lei et al. (4) hypothesized that the gut microbiota composition might differ between acute MI patients with T2DM (AMIDM) and acute MI patients without T2DM (AMINDM), potentially conferring poorer prognosis in AMIDM patients, and it set out to investigate this hypothesis by examining the gut microbiota profiles of patients belonging to these two groups.
Using 16S rDNA sequencing to examine the different compositions and functions of the gut microbiota from 15 AMIDM and 15 AMINDM patients, the study found their postulated hypothesis to hold true: gut microbiota compositions do differ between the two groups and also predict their functions (4). Importantly, significant correlations between certain bacterial species and clinical parameters of AMI patients were uncovered, as well (4). Microbial shaping and colonization of the gut begins perinatally and changes in its content are influenced by various factors, including genetic, dietary, and environmental factors (5). Dietary habits, medications, and genetics are all known to precipitate dysbiosis of the gut microbiota, contributing to T2DM pathogenesis (5,6). The differences observed in the study by Lei et al. (4) most likely stemmed from variations in diet and genetic background of the study’s patients.
Regarding specific findings of the study, Firmicutes and Bacteroidetes were found to be the most prevalent phyla (4). Indeed, alterations in these two phyla have already been strongly associated with cardiovascular and metabolic disease progression in previous studies (7,8). Of note, most of the bacteria species belonging to these two phyla are short chain fatty acid (SCFA)-producing species, i.e., butyrate, propionate, or acetate metabolite-producing microbes, which means that these alterations in the Firmicutes/Bacteroidetes levels or ratios may promote heart disease through SCFA-dependent mechanisms (8). Consistent with other studies suggesting an elevated Firmicutes/Bacteroidetes ratio might accompany various diseases, such as T2DM, obesity, and atherosclerosis/coronary artery disease (9-11), Lei et al. also found an increase in Firmicutes abundance but a decrease in Bacteroidetes levels in AMIDM patients (4). This indicates that a higher Firmicutes/Bacteroidetes ratio might be a feature of acute MI with T2DM, as well. Most bacteria belonging to the Firmicutes are gram-positive and associated with unhealthy lifestyles, such as a high-fat diet (12), energy imbalance (13), and high body mass index (overweight) (14). In contrast, Bacteroidetes consist of gram-negative bacteria, which can be induced by consumption of fiber-rich or carbohydrate-rich diets, and whose population seems to decrease as atherosclerosis progresses (15-17). Of note, several studies investigating specific gut microbial profiles of coronary artery disease patients seem to agree that a common feature of gut microbiota alterations in these patients is an increased Firmicutes/Bacteroidetes ratio, i.e., higher Firmicutes and lower Bacteroidetes species levels (9,18,19). The study by Lei et al. adds patients with AMIDM to the list of heart disease patients presenting this altered gut microbiota signature (4). Whether the presence of T2DM complicating MI in these patients alters this ratio itself to promote inflammation and insulin resistance, or the higher Firmicutes-to-Bacteroidetes ratio is the result of the increased systemic and cardiovascular chronic inflammation and/or the metabolic syndrome caused by the diabetes, remains an open but very important question awaiting answer in future studies. It is also plausible that both possibilities could be in play: the higher Firmicutes/Bacteroidetes ratio could both be involved in the pathogenesis of T2DM-induced cardiovascular inflammation and metabolic derangements that can precipitate an MI and, at the same time, be the result of T2DM-induced gut microbiota dysbiosis that accompanies coronary artery disease and acute MI.
Interestingly, the higher colonic Firmicutes/Bacteroidetes ratio has been consistently linked with elevated SCFA metabolite production/levels (20-22). However, it remains to be determined whether the increased production of SCFAs is beneficial or detrimental for the patient, i.e., whether increased SCFA metabolite production confers protection against, or further aggravates the risk of metabolic disorders, obesity, and cardiovascular disease, including MI. Some studies claim it is beneficial/protective (20) while some others provide evidence for its association with increased disease risk (21,22).
The reason behind these discrepancies may lie in the fact that these gut SCFA metabolites work through multi-functional receptors which exert a variety of tissue-specific effects, some of which are beneficial and some toxic, depending on the cell/tissue type and disease context. Indeed, SCFAs are not only energy fuel sources for the cells but also act as hormone signals on target tissues/cells by activating two of the four known free fatty acid receptors (FFARs), FFAR2 and FFAR3 (23). All FFARs are G protein-coupled receptors (GPCRs) and FFAR2 and 3 are expressed in the gut, adipose tissue, bone marrow, liver, muscles, lungs, brain, heart, and in sympathetic neurons (23). This broad tissue expression underlies the versatile and important roles FFAR2 and FFAR3 play in several pathologies, such as diabetes, obesity, metabolic syndrome, inflammatory bowel diseases, asthma, gout/arthritis, and cardiovascular diseases, including MI (23). Like all GPCRs, when FFARs are activated by ligands, they couple to heterotrimeric G proteins, inducing dissociation of the Ga from the Gbg subunits, both of which are then free to elicit intracellular signaling cascades by activating or inhibiting effectors (23). FFAR2 couples primarily to Gi/o and Gq/11 proteins (Figure 1) (23). Therefore, via Gi/o protein activation, it inhibits adenylyl cyclase (AC) and lowers intracellular cAMP levels but also activates ERK1/2 (Figure 1). On the other hand, via Gq/11 protein activation, FFAR2 increases intracellular [Ca2+] and, again, promotes activation of ERKs and other mitogen-activated protein kinases (MAPKs) (Figure 1). FFAR2 plays important roles in a variety of pathologies, including diabetes mellitus as it elevates glucagon-like peptide (GLP)-1 and peptide YY (peptide tyrosine-tyrosine, PYY) levels (Figure 1) (23). One of the most important functions of FFAR2 is regulation of energy storage in fat cells and of adipogenesis, which puts this receptor on front stage in metabolic syndrome pathogenesis. Indeed, FFAR2 has been shown to increase adipogenesis and acetate or propionate upregulate FFAR2 in murine adipose tissues, leading to lower plasma fatty acid levels and decreased lipolysis (Figure 1) (23). The role of FFAR2 in adipogenesis has been documented in mice lacking this receptor and fed a high fat diet (HFD) These animals have leaner body mass improved blood glucose levels and better lipidemic profile and energy utilization profiles, including higher brown adipose tissue (BAT) density (“browning” of adipose tissue) and suppressed white adipose tissue (WAT) inflammation (23).
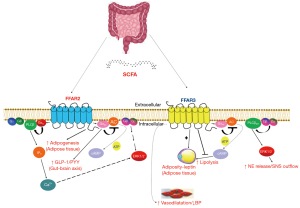
On the other hand, FFAR3 (also known as GPR41) is minimally activated by acetic acid (the shortest of the SCFAs) and, instead, shows preference for the longest-chain SCFAs, such as valeric acid (with 5 C atoms) or caproic acid (6 C atoms) for activation (23). Additionally, FFAR3 signaling seems to proceed exclusively via the pertussis toxin-sensitive Gi/o proteins (Figure 1), unlike FFAR2 which couple to Gq/11 proteins, as well. Indeed, FFAR3 stimulation with SCFAs inhibits AC and lowers intracellular cAMP synthesis via Gai subunit activation but also promotes ERK1/2 phosphorylation and activation via Gi/o-derived free Gbg subunits (Figure 1). FFAR3 also regulates cardiac function via stimulation of the sympathetic nervous system (Figure 1) (24). FFAR3 is abundant in murine peripheral sympathetic neurons, including cardiac sympathetic nerve terminals, wherein it regulates intermediary metabolism and sympathetic neuronal activity via facilitation of norepinephrine release (Figure 1) (24). The signaling pathway underlying the sympatho-stimulatory effect of FFAR3 is Gi/o-derived free Gβγ subunit activation of phospholipase C (PLC)-β2/3 (Figure 1) (24). Gβγ-activated PLCβ2/3 activates, in turn, ERK1/2, which phosphorylate synapsin-2β at Ser426 to induce vesicle fusion with the neuronal plasma membrane and norepinephrine exocytosis/synaptic release from sympathetic nerve endings (24). Notably, regulator of G protein signaling (RGS)-4 binds Gi/o-derived free Gβγ subunits and PLCβ and blocks PLCβ activation independently of its GTPase-activating (GAP) function (24). Indeed, RGS4 was recently shown to oppose FFAR3 signaling to PLCβ via Gi/o-derived free Gβγ subunits and to reduce Ca2+ signaling from FFAR3, translating into less inflammation in the heart and lower norepinephrine release and firing activity in cardiac sympathetic neurons (24). This suggests that FFAR3 antagonism, which can be achieved with a ketone body such as 3-hydroxybuyrate, might be of therapeutic value in ischemic cardiomyopathies or cardiac metabolic dysfunction.
FFAR3-dependent AC inhibition and cAMP lowering also reduces lipolysis by inhibiting peripheral triglyceride lipases, accompanied by hepatic lipogenesis and fat cell proliferation (Figure 1) (23). In addition, an interesting role for FFAR3 in vascular smooth muscle cells in regulation of blood pressure and vascular tone has been reported, which implicates this receptor in hypertension development, as well (Figure 1) (23). More specifically, FFAR3 is expressed in the smooth muscle cells of small resistance blood vessels and mice lacking this receptor develop hypertension upon antibiotic treatment (which usually reduces gut microbial SCFA production/fermentation). Indeed, propionic acid has been reported to induce vasodilation ex vivo, producing acute hypotensive responses and conferring protection against hypertension (Figure 1) (23). However, FFAR3-induced norepinephrine release from sympathetic neurons, including those that innervate the renal juxtaglomerular apparatus of the kidney that produces renin, could result in elevation, rather than reduction, of blood pressure (via renin-angiotensin-aldosterone system activation), so the net effect of FFAR3 activation on blood pressure regulation is probably much more complicated. Thus, the precise role of FFAR3 in hypertension awaits elucidation. Nevertheless, it becomes clear from all these studies above that the picture of the effects of either FFAR2 or FFAR3 on cardiovascular and endocrine functions is quite complex, with the same FFAR sometimes mediating opposite effects in different tissue types (e.g., FFAR3 in hypertension). Therefore, it should not come as a surprise that, especially, the cardiovascular effects of the gut microbial SCFA metabolites are not yet fully delineated. In all probability, whether the effect of a SCFA metabolite is beneficial or toxic is dependent on the particular tissue/cell type in which it is examined.
Lei et al. also investigated correlations of specific clinical parameters with the gut microbiota of AMI patients (4). Among the salient findings was that Dorea longicatena positively, but Phascolarctobacterium faecium negatively, correlated with HbA1c (glycated hemoglobin), a potential predictor of post-MI clinical outcomes in diabetes mellitus patients. This strongly implies that the Phascolarctobacterium faecium species is a mitigating factor, whereas the Dorea longicatena species is an aggravating factor in determining MI risk of AMIDM patients. Higher propensity for metabolic dysregulation among patients with AMIDM may underlie, at least in part, worse outcomes and the higher heart attack risk of AMIDM patients compared to the AMINDM population (25). Overall, the study by Lei et al. (4) provides yet more strong evidence for the association of commensal bacteria composition changes with glucose and lipid metabolism disorders, and for their potential value as biomarkers of poor outcomes in diabetic MI patients.
Of course, the study was hampered by several limitations, such as the fact it was an observational study with a relatively small sample size and that it only studied patients of Chinese ethnicity, which prohibits extrapolation of its findings to other racial or ethnic groups (4). Another limitation was the single time point used for sample collection, which prevented follow up or examination of potential gut microbiota composition changes over time. Nevertheless, the study presents for the first time the gut microbiota profiles of an AMIDM population using the powerful and reliable 16S rDNA sequencing method which enabled associations at the microbial species level. With the advent of better, more powerful integrated multi-omics analyses, the roles of gut microbiota in the pathophysiological processes of AMIDM will be even more amenable to molecular investigations in the future. Coupled with studies in animal models to delineate specific molecular pathophysiological mechanisms, these studies will have the potential of establishing associations of individual gut microbiota composition changes with specific clinical outcomes in diabetic heart attack patients.
In conclusion, the presence of the type 2 diabetes co-morbidity brings about important changes in the composition and predictive function of the gut microbiota in heart attack patients; thus, gut microbiota might be a useful diagnostic and/or prognostic marker of acute MI in diabetics. Future studies on the differential composition of the metabolites produced by these microbiotas, including alterations in relative concentrations of individual SCFA metabolites, in the presence or absence of diabetes mellitus may help establish these gut microbial profile differences as targets for cardiovascular therapies, as well.
Acknowledgments
Funding: The author is supported by a grant from
Footnote
Provenance and Peer Review: This article was commissioned by the editorial office, Annals of Translational Medicine. The article did not undergo external peer review.
Conflicts of Interest: The author has completed the ICMJE uniform disclosure form (available at https://atm.amegroups.com/article/view/10.21037/atm-23-1741/coif). The author has no conflicts of interest to declare.
Ethical Statement: The author is accountable for all aspects of the work in ensuring that questions related to the accuracy or integrity of any part of the work are appropriately investigated and resolved.
Open Access Statement: This is an Open Access article distributed in accordance with the Creative Commons Attribution-NonCommercial-NoDerivs 4.0 International License (CC BY-NC-ND 4.0), which permits the non-commercial replication and distribution of the article with the strict proviso that no changes or edits are made and the original work is properly cited (including links to both the formal publication through the relevant DOI and the license). See: https://creativecommons.org/licenses/by-nc-nd/4.0/.
References
- Han Y, Gong Z, Sun G, et al. Dysbiosis of Gut Microbiota in Patients With Acute Myocardial Infarction. Front Microbiol 2021;12:680101. [Crossref] [PubMed]
- Wu ZX, Li SF, Chen H, et al. The changes of gut microbiota after acute myocardial infarction in rats. PLoS One 2017;12:e0180717. [Crossref] [PubMed]
- Craciun CI, Neag MA, Catinean A, et al. The Relationships between Gut Microbiota and Diabetes Mellitus, and Treatments for Diabetes Mellitus. Biomedicines 2022;10:308. [Crossref] [PubMed]
- Lei C, Zhang X, Chen E, et al. Compositional alterations of the gut microbiota in acute myocardial infarction patients with type 2 diabetes mellitus. Ann Transl Med 2023;11:317. [Crossref] [PubMed]
- Gurung M, Li Z, You H, et al. Role of gut microbiota in type 2 diabetes pathophysiology. EBioMedicine 2020;51:102590. [Crossref] [PubMed]
- Scheithauer TPM, Rampanelli E, Nieuwdorp M, et al. Gut Microbiota as a Trigger for Metabolic Inflammation in Obesity and Type 2 Diabetes. Front Immunol 2020;11:571731. [Crossref] [PubMed]
- Da Silva CC, Monteil MA, Davis EM. Overweight and Obesity in Children Are Associated with an Abundance of Firmicutes and Reduction of Bifidobacterium in Their Gastrointestinal Microbiota. Child Obes 2020;16:204-10. [Crossref] [PubMed]
- Robles-Vera I, Toral M, de la Visitación N, et al. Probiotics Prevent Dysbiosis and the Rise in Blood Pressure in Genetic Hypertension: Role of Short-Chain Fatty Acids. Mol Nutr Food Res 2020;64:e1900616. [Crossref] [PubMed]
- Sawicka-Smiarowska E, Bondarczuk K, Bauer W, et al. Gut Microbiome in Chronic Coronary Syndrome Patients. J Clin Med 2021;10:5074. [Crossref] [PubMed]
- Huang L, Thonusin C, Chattipakorn N, et al. Impacts of gut microbiota on gestational diabetes mellitus: a comprehensive review. Eur J Nutr 2021;60:2343-60. [Crossref] [PubMed]
- Stojanov S, Berlec A, Štrukelj B. The Influence of Probiotics on the Firmicutes/Bacteroidetes Ratio in the Treatment of Obesity and Inflammatory Bowel disease. Microorganisms 2020;8:1715. [Crossref] [PubMed]
- Bisanz JE, Upadhyay V, Turnbaugh JA, et al. Meta-Analysis Reveals Reproducible Gut Microbiome Alterations in Response to a High-Fat Diet. Cell Host Microbe 2019;26:265-272.e4. [Crossref] [PubMed]
- Schwiertz A, Taras D, Schäfer K, et al. Microbiota and SCFA in lean and overweight healthy subjects. Obesity (Silver Spring) 2010;18:190-5. [Crossref] [PubMed]
- Gomes AC, Hoffmann C, Mota JF. The human gut microbiota: Metabolism and perspective in obesity. Gut Microbes 2018;9:308-25. [Crossref] [PubMed]
- Faith JJ, McNulty NP, Rey FE, et al. Predicting a human gut microbiota's response to diet in gnotobiotic mice. Science 2011;333:101-4. [Crossref] [PubMed]
- Lozupone CA, Stombaugh JI, Gordon JI, et al. Diversity, stability and resilience of the human gut microbiota. Nature 2012;489:220-30. [Crossref] [PubMed]
- Jie Z, Xia H, Zhong SL, et al. The gut microbiome in atherosclerotic cardiovascular disease. Nat Commun 2017;8:845. [Crossref] [PubMed]
- Emoto T, Yamashita T, Sasaki N, et al. Analysis of Gut Microbiota in Coronary Artery Disease Patients: a Possible Link between Gut Microbiota and Coronary Artery Disease. J Atheroscler Thromb 2016;23:908-21. [Crossref] [PubMed]
- Cui L, Zhao T, Hu H, et al. Association Study of Gut Flora in Coronary Heart Disease through High-Throughput Sequencing. Biomed Res Int 2017;2017:3796359. [Crossref] [PubMed]
- Santos-Marcos JA, Perez-Jimenez F, Camargo A. The role of diet and intestinal microbiota in the development of metabolic syndrome. J Nutr Biochem 2019;70:1-27. [Crossref] [PubMed]
- Popli S, Badgujar PC, Agarwal T, et al. Persistent organic pollutants in foods, their interplay with gut microbiota and resultant toxicity. Sci Total Environ 2022;832:155084. [Crossref] [PubMed]
- Rahat-Rozenbloom S, Fernandes J, Gloor GB, et al. Evidence for greater production of colonic short-chain fatty acids in overweight than lean humans. Int J Obes (Lond) 2014;38:1525-31. [Crossref] [PubMed]
- Lymperopoulos A, Suster MS, Borges JI. Short-Chain Fatty Acid Receptors and Cardiovascular Function. Int J Mol Sci 2022;23:3303. [Crossref] [PubMed]
- Carbone AM, Borges JI, Suster MS, et al. Regulator of G-Protein Signaling-4 Attenuates Cardiac Adverse Remodeling and Neuronal Norepinephrine Release-Promoting Free Fatty Acid Receptor FFAR3 Signaling. Int J Mol Sci 2022;23:5803. [Crossref] [PubMed]
- Shiyovich A, Gilutz H, Plakht Y. Serum electrolyte/metabolite abnormalities among patients with acute myocardial infarction: comparison between patients with and without diabetes mellitus. Postgrad Med 2021;133:395-403. [Crossref] [PubMed]