Treatment for hepatitis B in patients with drug resistance
Introduction
The hepatitis B virus (HBV) is a small, partially double stranded DNA virus that causes acute and chronic hepatitis in humans. About 240 million people are persistently infected with HBV, making HBV one of the most hazardous viral pathogens for humans and a global public health concern. Despite tremendous advances with universal vaccination programs, highly effective antiviral therapies and guidelines for screening of HBV infected patients for liver cancer (1), the number of HBV-related deaths due to cirrhosis or hepatocellular carcinoma increased between 1990 and 2013 by 33% to >686,000 cases in 2013 worldwide (2). The main treatment goal for chronic hepatitis B is to suppress viral replication and consequently to prevent progression to liver fibrosis and cirrhosis, liver failure and development of hepatocellular carcinoma (3,4). In most cases, this is achieved by the orally available nucleotide/nucleoside analogues lamivudine (LMV), telbivudine (LdT), adefovir dipivoxil (ADV), entecavir (ETV) or tenofovir (TDF), while only a minor proportion of HBV-monoinfected patients is treated with (pegylated) interferon-alpha (5). Nucleotide and nucleoside analogues act as competitive inhibitors of the HBV reverse transcriptase, as their incorporation into the DNA strand provokes chain termination (6).
The HBV polymerase lacks proof-reading function, which results in a high number of mutant viral genomes in infected individuals. It has been estimated that the daily rate of de novo HBV production can reach 1011 virions in chronically infected patients, with an estimated mutation frequency of 1.4×10−5–3.2×10−5 nucleotides (7). Particular selection pressures, both endogenous (host immune clearance) and exogenous (vaccines and antivirals), readily select escape mutants and strongly influence the predominant HBV quasispecies in an infected individual. It is therefore of utmost importance to regularly monitor HBV-infected patients undergoing antiviral therapy for the HBV viral load as well as for signs of liver injury such as elevated alanine aminotransferase (ALT) activity (3).
Clinical relevance and detection of drug resistance
The clinical relevance of drug resistance became dramatically clear after the introduction of the first nucleoside (deoxy-cytidine) analogue LMV that has a low barrier to resistance. LMV-resistant mutations arise in about 23% of patients after 12 months of therapy and in up to 80% after 5 years of treatment (6). Patients with LMV-resistant mutations have a higher risk of deteriorating liver function (e.g., increase in Child-Pugh score), increasing signs of liver injury (e.g., ALT levels) as well as developing cirrhosis and hepatocellular carcinoma, all in comparison to patients with wildtype virus under antiviral therapy (3,8-10). Viral rebound and hepatic decompensation is also observed with other drug-resistant HBV mutants (11). The risk of selecting antiviral therapy-resistant mutants is related to the pretreatment HBV DNA level, the choice of the antiviral (low/high barrier), the duration of treatment, the rapidity of viral response/viral suppression as well as to the previous exposure to nucleotide/nucleoside analogues (12). In order to reduce the risk of drug resistance, all guidelines now recommend the use of newer, highly potent antivirals with a high barrier to resistance such as ETV or TDF (3,4).
Due to the relevance of continued viral suppression for preventing disease progression and subsequent complications, it is essential to regularly monitor patients undergoing antiviral therapy. As a rule of thumb, HBV-DNA testing should be ideally <200 IU/mL after 6 months of therapy and negative (or close to negative, i.e., below 10–15 IU/mL) after 12 months (13). If HBV-DNA remains detectable after 48 weeks of treatment, this has been traditionally called “persistent viremia”. However, with the current preferred therapies of highly potent drugs like ETV and TDF, persistent viremia is defined as a plateau in the decline of HBV DNA and/or failure to achieve undetectable HBV DNA level after 96 weeks of therapy (4).
Most guidelines recommend testing HBV-DNA serum levels every 3 months during the first year of treatment and at least every 6 months thereafter (3,4,13). There are different definitions about “drug resistance”, but the failure of reducing viral load by one log within three months of therapy is suspicious for drug resistance (3). The most recent AASLD guidelines define viral breakthrough as an increase in HBV DNA by >1 log compared to nadir or HBV DNA ≥100 IU/mL in persons on nucleoside/nucleotide analogue therapy with previously undetectable levels (<10 IU/mL) (4). From a practical point of view, it is important to confirm “viral breakthrough” by a second measurement before changing the therapy, and non-compliance needs to be ruled out. If the latter is excluded, drug resistance is likely, and HBV resistance testing can be performed, as this may help in deciding about the subsequent therapy (4). Figure 1 shows the clinical algorithm for testing and managing HBV-infected patients undergoing nucleoside/nucleotide analogue therapy.
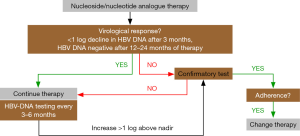
Molecular mechanisms of drug resistance
Different types of mutations are associated with drug resistance and can emerge during antiviral therapy with nucleoside or nucleotide analogues. Primary mutations typically affect the reverse transcriptase domain of the HBV polymerase, thereby causing steric changes of the polymerase protein that escape the inhibitory effects of the nucleos(t)ide analogues (7,12,14). The most relevant hot-spot mutations in the HBV polymerase are displayed in Table 1. However, the polymerase mutants have a dramatically reduced viral replication efficacy in most cases (14). Secondary compensatory mutations occur in order to restore the viral replication fitness, thereby overcoming deleterious effects of the primary drug-resistant mutations (12). These mutations are not necessarily located within the enzymatically active sites of the polymerase, but oftentimes stabilize secondary or tertiary viral structures. The eight different HBV genotypes A–H partly differ with respect to the position of secondary compensatory mutations and the rate of drug resistance development (15).
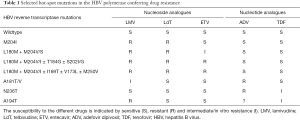
Full table
LMV
As mentioned above, LMV is the approved antiviral drug with the highest resistance rate. The most common mutations conferring LMV resistance affect the tyrosine-methionine-aspartate-aspartate (YMDD) motif at the active site of the HBV polymerase, where the methionine (M) residue at amino acid 204 is replaced by isoleucine (rtM204I) or valine (rtM204V). The rtM204V mutation is usually accompanied by a leucine (L) to M or occasionally serine (S) change at position 180 (rtL180M/S) (6,12). HBV viral strains with the LMV-resistant mutations display drug resistance against LMV, but have a decreased replication capacity in vitro (14). Interestingly, the double point mutants L180M/M204V replicate better than the single M204I mutants, possibly due to the stabilizing effect of the L180M mutation (B domain) on the YMDD loop (C domain) in a conformational model of the HBV reverse transcriptase (7). Another compensatory secondary mutation is the rtV173L that improves the impaired replication of rtM204V/I mutants (6).
ADV
The nucleotide analogue ADV is effective in LMV-resistant variants, but also has a substantial 30% resistance rate after 5 years of treatment (16). The two major resistance mutations are an asparagine to threonine substitution at rt236 in the domain D (rtN236T) and the rtA181V/T mutation affecting the B domain of the HBV polymerase (7). Although there is no absolute cross-resistance, TDF shows a reduced activity against ADV-resistant HBV strains in vitro (17). Moreover, it is also possible that dual resistant mutations against LMV and ADV can occur, if ADV treatment follows LMV treatment (11).
LdT
Resistance rates against LdT are lower compared to LMV, but significantly higher compared to ETV or TDF, reaching about 17% after 2 years (3). Very similar to LMV, the drug selects for YMDD mutations (mostly M204I). In addition, rtA181T/V and rtL229W/V were described as mutations conferring LdT resistance (12).
ETV
If ETV is used as first-line therapy, the rate of resistance is very low, approximately or below 1% after 5 years of therapy (18,19). However, the rate of ETV resistance is much higher in patients that are already LMV resistant, because ETV resistance requires the “YMDD mutation(s)” (rtM204V/I ± L180M) plus an additional ETV ‘signature’ substitution in the B domain (rtI169T or rtS184G), C domain (rtS202G/I), or E domain (rtM250V) (12). The presence of any ETV resistance drastically reduces viral replication when compared to wildtype HBV in vitro (20).
TDF
At present, no typical TDF-resistant mutations have been described, even after 7 years of antiviral treatment (21). However, TDF shares some structural similarities to ADV, raising the concern of potential cross-resistance between both drugs. In fact, TDF is less effective against mutant HBV strains containing the primary mutations associated with ADV resistance (rtA181T/V and/or rtN236T) in vitro (17). Patients with ADV-resistant HBV show an impaired response to TDF compared to patients without ADV resistance (22). More specifically, the rtA194T polymerase mutation has been found in HBV/HIV coinfected patients during TDF treatment, suggesting an association with TDF resistance (23). In vitro, this rtA194T polymerase mutation is associated with a partial drug resistance against TDF. On the other hand, the rtA194T mutation impairs replication competence of HBV constructs in vitro, possibly explaining the low occurrence of this mutant in clinical practice (24).
Multidrug resistance
There are several reports of complex patient histories involving the sequential use of different antivirals. Such patients are at risk of developing “multi-drug resistant” HBV (12). The occurrence of such complex genomic HBV variants might be exceptionally high in conditions of immune suppression such as following liver transplantation (25).
Notably, mutations in the HBV polymerase that are related to drug resistance might affect the structure of the HBV envelope (S protein) as well, because the open reading frames of these genes partially overlap (7). For instance, the rtV191I polymerase mutation that has been observed in an HBV/HIV coinfected patient during TDF-containing therapy creates a stop-codon in the overlapping surface antigen (sW182stop). This results in the deletion of the last 44 amino acids of the HBsAg, which then escapes detection (“HBsAg-negativity”) in routine diagnostic serum tests (26).
Practical management of drug resistance
In cases of a confirmed virological breakthrough and exclusion of non-adherence (Figure 1), the patient should be either switched to another antiviral monotherapy with a high genetic barrier to resistance (ETV or TDF) or a second antiviral drug with a complementary resistance profile should be added (4). Although it appears reasonable from a virological point to reduce resistance by combination therapy, there are no clear long-term data favoring the “add-on” over the “switch” concept (4,12). Table 2 summarizes current recommendations for the management of treatment failure.
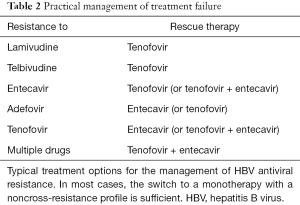
Full table
LMV/LdT resistance
Patients with resistance to LMV or LdT should be immediately switched to TDF monotherapy. A recent meta-analysis revealed that TDF monotherapy is as effective as TDF-based combination therapy in maintaining viral suppression in LMV-resistant patients with prior failure of either LMV or LMV/ADF therapy (27). ETV should not be used, due to the higher risk of selecting ETV-resistant mutations with pre-existing LMV-resistance (Table 1) (6).
ETV resistance
In case of ETV resistance, one can either add TDF or switch to TDF monotherapy, as no cross-resistance can be expected. A randomized study including 90 patients with ETV resistance suggested that both strategies (TDF alone vs. ETV-TDF combination) are similarly effective in suppression of viral replication (28). However, both highly effective drugs can be safely combined and represent an efficient rescue in patients with complex resistance patterns (29).
ADV resistance
ETV has no cross-resistance with ADV (17) and is therefore effective in patients with ADV-resistant HBV mutants (4). There are some data from observational cohort studies suggesting that the efficacy of TDF is diminished in patients with ADV resistance (22,30). However, in a more recent randomized study comparing TDF or TDF + ETV in 102 patients with ADV-resistant chronic hepatitis B, both strategies were similarly effective (28). Thus, either ETV or TDF monotherapy or TDF-ETV combination may be considered in ADV resistance (3,4,28).
TDF resistance and multi-drug resistance
Although there is no signature mutation pattern conferring TDF resistance, cases of insufficient responses to TDF have been reported (23). Considering the noncross-resistance between ETV and TDF, the use of ETV should be effective in these cases, either as mono- or combination therapy (Table 2). In vitro data indeed demonstrated that ETV is effective against TDF-resistant strains (24,26). The situation might be more complex in patients with multiple drug-resistant mutations due to an extended treatment history. A retrospective European multicenter study revealed that TDF monotherapy induced a potent and long-lasting antiviral response in LMV- and/or ADV-experienced patients with previous treatment failure (22). Moreover, the combination of ETV and TDF is a highly effective rescue therapy in patients with treatment failure after exposure to multiple drugs (29).
Outlook
Inspired by the great success in eliminating hepatitis C virus from infected individuals by novel direct acting antiviral drugs (31), many current research efforts aim at developing new concepts to achieve a cure for HBV infections as well. On the one hand, this involves the development of new antivirals that target different parts of the viral replication cycle and/or the host’s immune response (32). For instance, HBV entry inhibitors, siRNA against viral transcripts, inhibitors of the HBV capsid formation or drugs targeting the cccDNA are currently being tested in preclinical and early clinical studies (33). Different attempts to strengthen either innate (e.g., by activating TLR pathways or providing cytokines) or adaptive immune responses (e.g., by immune checkpoint inhibitors, therapeutic vaccination or T-cell transfer) might be needed to eradicate the virus or stably control HBV (32,33).
A different approach is the termination of nucleoside/nucleotide analogue therapy. This was supported by an observational study from Greece, demonstrating that HBeAg-negative patients that stopped ADV therapy after 4–5 years of sustained viral suppression may to a large proportion either endogenously control viral replication (about 55%) or even lose HBsAg (39%) (34). Several controlled trials are ongoing to test this approach and identify the ideal candidates of patients for discontinuation of therapy. A recent meta-analysis summarizing the data from available, yet heterogeneous studies reported a virological remission after nucleoside/nucleotide discontinuation in about 38% of the patients (35). From the perspective of drug resistance, this approach is certainly challenging (4). After cessation of antiviral therapy, almost all patients relapse with increased viral load and oftentimes biochemical signs of liver injury (34). Although this might induce immune responses ultimately controlling or clearing HBV, this poses the risk of selecting and expanding drug-resistant mutants (4).
Conclusions
Current antiviral therapies for hepatitis B aim at stably suppressing viral replication in order to prevent HBV-related complications such as hepatic decompensation, liver cirrhosis and hepatocellular carcinoma. The currently recommended nucleoside and nucleotide analogues ETV and TDF have a very low risk of drug resistance. However, drug resistance can occur in patients during antiviral therapy. Regular testing for HBV DNA (every 3–6 months) is essential to early detect treatment failure. Different nucleoside and nucleotide analogues select distinct drug-resistant HBV mutants. In cases of treatment failure and drug resistance, switching to a noncross-resistant antiviral or adding a second highly effective antiviral drug represent recommended rescue strategies.
Acknowledgements
Funding: This work was supported by the German Research Foundation (DFG Ta434/3-1 & SFB/TRR57).
Footnote
Conflicts of Interest: The authors have no conflicts of interest to declare.
References
- Locarnini S, Hatzakis A, Chen DS, et al. Strategies to control hepatitis B: Public policy, epidemiology, vaccine and drugs. J Hepatol 2015;62:S76-86. [Crossref] [PubMed]
- Stanaway JD, Flaxman AD, Naghavi M, et al. The global burden of viral hepatitis from 1990 to 2013: findings from the Global Burden of Disease Study 2013. Lancet 2016;388:1081-8. [Crossref] [PubMed]
- European Association For The Study Of The Liver. EASL clinical practice guidelines: Management of chronic hepatitis B virus infection. J Hepatol 2012;57:167-85. [Crossref] [PubMed]
- Terrault NA, Bzowej NH, Chang KM, et al. AASLD guidelines for treatment of chronic hepatitis B. Hepatology 2016;63:261-83. [Crossref] [PubMed]
- Aghemo A, Lampertico P, Colombo M. Assessing long-term treatment efficacy in chronic hepatitis B and C: between evidence and common sense. J Hepatol 2012;57:1326-35. [Crossref] [PubMed]
- Tong S, Revill P. Overview of hepatitis B viral replication and genetic variability. J Hepatol 2016;64:S4-16. [Crossref] [PubMed]
- Tacke F, Manns MP, Trautwein C. Influence of mutations in the hepatitis B virus genome on virus replication and drug resistance--implications for novel antiviral strategies. Curr Med Chem 2004;11:2667-77. [Crossref] [PubMed]
- Lai CL, Dienstag J, Schiff E, et al. Prevalence and clinical correlates of YMDD variants during lamivudine therapy for patients with chronic hepatitis B. Clin Infect Dis 2003;36:687-96. [Crossref] [PubMed]
- Yuen MF, Seto WK, Chow DH, et al. Long-term lamivudine therapy reduces the risk of long-term complications of chronic hepatitis B infection even in patients without advanced disease. Antivir Ther 2007;12:1295-303. [PubMed]
- Liaw YF, Sung JJ, Chow WC, et al. Lamivudine for patients with chronic hepatitis B and advanced liver disease. N Engl J Med 2004;351:1521-31. [Crossref] [PubMed]
- Fung SK, Andreone P, Han SH, et al. Adefovir-resistant hepatitis B can be associated with viral rebound and hepatic decompensation. J Hepatol 2005;43:937-43. [Crossref] [PubMed]
- Zoulim F, Locarnini S. Optimal management of chronic hepatitis B patients with treatment failure and antiviral drug resistance. Liver Int 2013;33 Suppl 1:116-24. [Crossref] [PubMed]
- Cornberg M, Protzer U, Petersen J, et al. Prophylaxis, diagnosis and therapy of hepatitis B virus infection - the German guideline. Z Gastroenterol 2011;49:871-930. [Crossref] [PubMed]
- Tacke F, Gehrke C, Luedde T, et al. Basal core promoter and precore mutations in the hepatitis B virus genome enhance replication efficacy of Lamivudine-resistant mutants. J Virol 2004;78:8524-35. [Crossref] [PubMed]
- Pourkarim MR, Amini-Bavil-Olyaee S, Kurbanov F, et al. Molecular identification of hepatitis B virus genotypes/subgenotypes: revised classification hurdles and updated resolutions. World J Gastroenterol 2014;20:7152-68. [Crossref] [PubMed]
- Salpini R, Alteri C, Cento V, et al. Snapshot on drug-resistance rate and profiles in patients with chronic hepatitis B receiving nucleos(t)ide analogues in clinical practice. J Med Virol 2013;85:996-1004. [Crossref] [PubMed]
- Herbers U, Amini-Bavil-Olyaee S, Mueller A, et al. Hepatitis B e antigen-suppressing mutations enhance the replication efficiency of adefovir-resistant hepatitis B virus strains. J Viral Hepat 2013;20:141-8. [Crossref] [PubMed]
- Chang TT, Lai CL, Kew Yoon S, et al. Entecavir treatment for up to 5 years in patients with hepatitis B e antigen-positive chronic hepatitis B. Hepatology 2010;51:422-30. [Crossref] [PubMed]
- Tenney DJ, Rose RE, Baldick CJ, et al. Long-term monitoring shows hepatitis B virus resistance to entecavir in nucleoside-naïve patients is rare through 5 years of therapy. Hepatology 2009;49:1503-14. [Crossref] [PubMed]
- Amini-Bavil-Olyaee S, Herbers U, Luedde T, et al. Impact of hepatitis B e antigen-suppressing mutations on the replication efficiency of entecavir-resistant hepatitis B virus strains. J Viral Hepat 2011;18:804-14. [Crossref] [PubMed]
- Buti M, Tsai N, Petersen J, et al. Seven-year efficacy and safety of treatment with tenofovir disoproxil fumarate for chronic hepatitis B virus infection. Dig Dis Sci 2015;60:1457-64. [Crossref] [PubMed]
- van Bömmel F, de Man RA, Wedemeyer H, et al. Long-term efficacy of tenofovir monotherapy for hepatitis B virus-monoinfected patients after failure of nucleoside/nucleotide analogues. Hepatology 2010;51:73-80. [Crossref] [PubMed]
- Sheldon J, Camino N, Rodés B, et al. Selection of hepatitis B virus polymerase mutations in HIV-coinfected patients treated with tenofovir. Antivir Ther 2005;10:727-34. [PubMed]
- Amini-Bavil-Olyaee S, Herbers U, Sheldon J, et al. The rtA194T polymerase mutation impacts viral replication and susceptibility to tenofovir in hepatitis B e antigen-positive and hepatitis B e antigen-negative hepatitis B virus strains. Hepatology 2009;49:1158-65. [Crossref] [PubMed]
- Betz-Stablein BD, Töpfer A, Littlejohn M, et al. Single-Molecule Sequencing Reveals Complex Genome Variation of Hepatitis B Virus during 15 Years of Chronic Infection following Liver Transplantation. J Virol 2016;90:7171-83. [Crossref] [PubMed]
- Amini-Bavil-Olyaee S, Sheldon J, Lutz T, et al. Molecular analysis of an HBsAg-negative hepatitis B virus mutant selected in a tenofovir-treated HIV-hepatitis B virus co-infected patient. AIDS 2009;23:268-72. [Crossref] [PubMed]
- Wang HL, Lu X, Yang X, et al. Efficacy of tenofovir-based rescue therapy in patients with lamivudine-resistant hepatitis B virus: A systematic review and meta-analysis. Clin Res Hepatol Gastroenterol 2016;40:447-56. [Crossref] [PubMed]
- Lim YS, Byun KS, Yoo BC, et al. Tenofovir monotherapy versus tenofovir and entecavir combination therapy in patients with entecavir-resistant chronic hepatitis B with multiple drug failure: results of a randomised trial. Gut 2016;65:852-60. [Crossref] [PubMed]
- Petersen J, Ratziu V, Buti M, et al. Entecavir plus tenofovir combination as rescue therapy in pre-treated chronic hepatitis B patients: an international multicenter cohort study. J Hepatol 2012;56:520-6. [Crossref] [PubMed]
- Patterson SJ, George J, Strasser SI, et al. Tenofovir disoproxil fumarate rescue therapy following failure of both lamivudine and adefovir dipivoxil in chronic hepatitis B. Gut 2011;60:247-54. [Crossref] [PubMed]
- Pawlotsky JM, Feld JJ, Zeuzem S, et al. From non-A, non-B hepatitis to hepatitis C virus cure. J Hepatol 2015;62:S87-99. [Crossref] [PubMed]
- Fung J, Lai CL, Seto WK, et al. Emerging drugs for the treatment of hepatitis B. Expert Opin Emerg Drugs 2016;21:183-93. [Crossref] [PubMed]
- Durantel D, Zoulim F. New antiviral targets for innovative treatment concepts for hepatitis B virus and hepatitis delta virus. J Hepatol 2016;64:S117-31. [Crossref] [PubMed]
- Hadziyannis SJ, Sevastianos V, Rapti I, et al. Sustained responses and loss of HBsAg in HBeAg-negative patients with chronic hepatitis B who stop long-term treatment with adefovir. Gastroenterology 2012;143:629-36.e1. [Crossref] [PubMed]
- Papatheodoridis G, Vlachogiannakos I, Cholongitas E, et al. Discontinuation of oral antivirals in chronic hepatitis B: A systematic review. Hepatology 2016;63:1481-92. [Crossref] [PubMed]