Effect of high fat-diet and obesity on gastrointestinal motility
Introduction
Obesity is defined as the accumulation of excess body fat leading to a high body mass index (>30) and is a growing epidemic in most countries around the world. It is the leading preventable cause of death, and can lead to heart disease, type 2 diabetes, certain types of cancer, and other disorders (1). Approximately 32% of adults and 17% of children and adolescents were obese in the United States in a study assessing data from 2009-2010 (2). Although the prevalence of obesity has stabilized during the last decade (3), we are still far away from the goal of less than 15% of adults and 5% of children being obese, set by The Healthy People 2010 program (2).
Obesity has been shown to be associated with changes in gastrointestinal motility (4). Altering gastric motility can have a big impact on appetite and satiety. The gastrointestinal tract regulates the rate of digestion and appetite to induce or inhibit hunger (5).
In this review, we describe the relationship between obesity and gastrointestinal motility. Using the key words: motility, obesity, esophagus, gastric emptying, gastric accommodation, small and large intestinal transit, and gastric electric stimulation, in PubMed, we reviewed the current literature in this field. We discuss the potential pathophysiological mechanisms for the changes in gastrointestinal motility and possible treatment measures. We outline the changes in the gastrointestinal tract focusing on the esophagus, stomach, and the small and large intestines.
Obesity, esophageal motility and GERD
In obese patients, epidemiological studies indicate a higher prevalence of gastroesophageal reflux disease (GERD) (6,7). There are several studies that describe the relationship between obesity and GERD (8). According to a public health survey in Norway, the odds ratio of having GERD are 3.1 (95% CI, 2.6-3.6) and 3.9 (95% CI, 3.3-4.7) for obese men and women, respectively (7). There is also positive correlation between obesity, BMI, and waist to hip ratio. Even in women with BMI under 25, a rise in BMI of 3.5 correlates with increased reflux episodes (6). Abdominal fat and waist to hip ratio has been shown to have an association with GERD independent of BMI, particularly in Caucasians (9). To ascertain the pathophysiology behind this fact, some researchers performed manometry and 24 hour pH studies in obese patients with GERD and found that these patients tend to have reduced lower esophageal sphincter (LES) pressure, higher incidence of transient LES relaxation, increased esophageal acid exposure and higher intragastric pressure (10-13). Consequently, GERD complications like Barrett’s esophagus and esophageal and gastroesophageal adenocarcinoma are more common in obese patients (14,15).
Another contributing factor to GERD in obese patients is the higher incidence of hiatal hernia (16,17). Patients with reflux who are obese also have a higher incidence of erosive esophagitis (18). In addition to GERD, there are some other esophageal motility disorders associated with obesity. A study done on morbidly obese patients during the evaluation for bariatric surgery found that of 61 obese individuals, 33 patients had abnormal manometric finding; 10 had defective LES, 11 with a hypertensive LES, 2 with diffuse esophageal spasm (DES), 2 with nutcracker esophagus and 14 with a nonspecific motility disorder (19). These results replicate older similar studies on morbidly obese patients (20). Contraction amplitude in the middle esophagus has shown to be increased among obese patients (10,21). Weight loss was found to be beneficial in reducing GERD symptoms (8) and intermediate levels of physical activity has been shown to relieve reflux symptoms among obese people (22).
In summary, several esophageal disorders were found to be more prevalent in obese patients. Amongst them, GERD is the most common, with the underlying mechanisms including loss of LES tone, presence of hiatal hernia, and increased intragastric pressure (23).
Obesity and gastric motility
The stomach acts as a reservoir for ingested food and the site for its mechanical and chemical breakdown into chyme. Gastric capacity and accommodation play an important role in the functions of the stomach including gastric emptying and digestion. When food enters the stomach, the proximal part of the stomach relaxes to act as a reservoir for the meal. Following this the gastric muscles begin to push the food against a closed pylorus to begin the process of mechanical digestion. The pylorus then opens to gradually allow the digested chyme through. This process, known as gastric emptying, varies between solid and liquid foods and is under neurohormonal regulation (24). Gastric fundic tone is maintained by vagal innervation. The presence of food stimulates the vagal sensory afferents, and transmits the information to the brainstem, which sends inhibitory signals to the gastric circular muscles to allow accommodation. This reflex can occur even in the presence of a balloon blocking gastric outflow, suggesting that reflexes within the stomach alone can initiate accommodation without the need for food to reach duodenum and cause hormonal release. However, a relatively stronger stimulus for gastric accommodation is the presence of nutrients in the duodenum (25).
There are several well-established techniques to measure gastric emptying including scintigraphy, nonradioactive isotope 13C breath test, ultrasound, water and nutrient drink test, electrogastrography and Magnetic resonance imaging (MRI). Scintigraphy is still the gold standard to accurately evaluate gastric emptying (26). Accommodation is measured by an inflated balloon and barostat, single-photon emission computed tomography (SPECT), MRI and sonography (27). Some studies argue that using the barostat to measure gastric accommodation is non-physiological however it is still the gold standard (27,28).
Gastric capacity and accommodation in obesity
Gastric capacity and gastric accommodation have been studied to evaluate any possible change in obese patients. Kim et al. in 2001 studied 13 obese and 19 normal weight people and measured the fasting and post prandial gastric size using SPECT and found no significant difference in the fasting and postprandial gastric size in obese patients compared to normal individuals suggesting no change in capacity and accommodation (29). One study that used a gastric balloon filled with water to compare gastric capacity between lean, obese and bulimic women found that obese women had intermediate gastric capacity and bulimic women has the highest capacity. After dividing obese women between binge and normal eating, they found that obese women with binge eating had a stomach capacity similar to bulimic women while normal eating women had a similar capacity to lean women (30). A study in Mexico used water load test to measure gastric capacity in 32 patients with high BMI and 12 volunteers found positive correlation between BMI and gastric capacity (31). On the other hand, a large study was performed in 433 participants to assess the reliability of SPECT in measuring gastric size and the effect of aging and BMI on accommodation. The results showed SPECT as a reliable method with acceptable intra and inter-individual coefficients of variation and no significant effect of age and BMI on accommodation (32). In summary, some studies suggest a moderate increase in gastric capacity in obese patients with no changes in accommodation.
Gastric emptying and obesity
The rate of gastric emptying is known to have an influence in the feeling of fullness and satiety, and therefore it has been suggested to be linked with obesity.
One important point is the difference between gastric emptying of liquid and solid foods. For liquid meals, emptying occurs in an exponential fashion. On the other hand, solid meal emptying is characterized by a lag phase for a period about 30 minutes followed by a linear emptying phase (24).
Several studies have assessed gastric emptying in obesity and found that it is increased in obese patients (33-35) with few exceptions in studies with small samples where gastric emptying is reduced (36). In 2006, Vazquez et al. performed a more detailed study by measuring gastric emptying, postprandial volume, and hormones in relation to body weight. Their results suggest that faster gastric emptying and lower postprandial volume are typical of obese patients (37). Other studies compared the rate of gastric emptying in obese and lean people using the breath test and found that solid gastric emptying was faster in obese and overweight patients (38).
On the other hand, there are studies that show gastric emptying is delayed in some situations in overweight patients or diet induced obese animal models and others did not find any difference between the two groups. Di Ciaula et al. studied the gastric emptying and postprandial gall bladder volume in three age groups of normal and obese individuals by ultrasonography in newborns and pre-adolescent and liquid test meal in adults and determined that gastric emptying is delayed in obese patients. The study concluded that obese patients develop age-related decrease in gastric motility secondary to insulin resistance. However, it is important to note that the authors used only liquid meals in this study (39). Buchholz et al. studied 19 morbidly obese and 20 non-obese humans in an attempt to explain the higher incidence of aspiration in obese patients. The GE was measured by scintigraphy and no significant difference were found between the two groups (40).
Animals with diet-induced obesity (DIO) were also found to exhibit slower gastric emptying in a study by Li et al. The delayed gastric emptying was associated with reduced ghrelin levels and increased cholecystokinin (CCK) and leptin levels. The authors suggest that this result could be a method of compensation by increasing satiety and decreasing food intake to balance weight gain in DIO-rodents (41). Another study on ob/ob mice shows accelerated GE after 10-11 weeks then delayed GE after 22-27 weeks. The latter finding was associated with blood glucose intolerance (42). Additional larger human studies need to be done to further assess gastric emptying in obesity. Based on the majority of the literature, it is likely that obesity is associated with acceleration of gastric emptying.
Gastric motility and influence of diet and hormones
There are several hormones that mediate the body’s response to fat in the diet. CCK, Peptide YY (PYY) and leptin inhibit appetite and induce delay in the gastric emptying, while ghrelin is an appetite stimulator and causes faster gastric emptying (24,43). Glucagon like peptide (GLP-1) has a negative feedback on gastric emptying and enhances accommodation (44,45).
Fat in the diet stimulates hormones production like CCK, PYY and GLP-1 (46). Intraduodenal infusion of fatty acids increases basal pyloric pressures and the levels of CCK and PYY in the blood stream and free fatty acids are more potent than triglycerides in inducing appetite inhibition and hormonal release from the gastrointestinal tract (47). The length of fatty acid acyl chain is an important factor in determining the response in the duodenum as higher number of carbon atoms (>12) in the chain cause increased levels of CCK and GLP-1 in the blood stream and more inhibition of gastric emptying (48). To determine if there was an adaptation to a dietary component in changing gastric emptying, Castiglione et al. put eight males on a high fat diet (55% energy from fat) or high carbohydrate meal (62% of energy from carbohydrates) for fourteen days and tested gastric emptying at the end of this period. The authors found acceleration of gastric emptying in the patients who had consumed a high fat meal but not the patients who were on the high carbohydrate meal (49). Expression of GLP-2 receptors in the fundus of the stomach was higher in high fat diet fed mice compared with mice fed on a standard diet. The response to exogenous GLP-2 was higher as well. This could be an adaptive response of the stomach to the high fat diet and serve to increase gastric relaxation in response to food (50).
To study the effect of fat on gastric motility and the mechanisms involved, several investigators fed patients with high fat diet for a short period of time and then gastric emptying and the related hormone levels were measured. These hormones include leptin, CCK, PYY and ghrelin. A number of these studies showed changes in both secretion and sensitivity to CCK. Others showed alteration in postprandial levels of PYY and ghrelin levels. Little et al. studied ten healthy males and they found after twenty-one days on a high fat diet that obese patients have increased fasting CCK levels but the similar upper gut motility (51). Another study with twelve males on a high fat diet for fourteen days, demonstrated accelerated gastric emptying but no changes in levels of hormones. The authors suggest that high fat diet induced changes in the sensitivity to these hormones rather than changes in their secretion rates (52). Vazquez et al. also studied the association between the faster gastric emptying in obese people and leptin, PYY and ghrelin levels and found higher leptin levels (possible leptin resistance) and lower postprandial reduction in ghrelin with similar PYY levels in both groups (37).
Mechanisms of altered gastric motility in obesity
Several animal studies have been conducted to explore the reasons behind the gastric motility changes associated with obesity. De Lartigue et al. studied leptin and CCK signaling in diet-induced obese (DIO) rats. The authors demonstrate that DIO rats develop insensitivity to leptin signals after eight weeks of high fat diet. The vagal afferent neurons (VAN) were cultured and stimulated by leptin and evaluated for their responsiveness by measuring the protein pSTAT3 expression. The authors found that VAN from DIO-rats were less sensitive to leptin stimulation (53). In a second study, they injected the leptin resistant rats with CCK-8S and the results show less response in leptin resistant rats. They conclude that leptin is required for proper interaction between CCK and its receptors on VAN (54). Daly et al. also found reduced afferent vagal response to the mechanical and chemical stimulation in the high fat fed mice; CCK and serotonin effect on vagal afferents were diminished (55). In another study, the effect of CCK-8 injection in obesity prone rats showed decreased response in this group of rats compared to obese resistant rats. This study examined the response by measuring the dorsal hindbrain expression of Fos-like immunoreactivity after CCK injection. The results were consistent with the previous studies and show decreased response to CCK in obese-prone rats (56). Intraintestinal infusion of oleate has been shown to activate myenteric neurons in the duodenum and jejunum, but not the ileum (57). High fat-diet fed rats also have decreased response to oleate intestinal infusion in terms of less inhibition of gastric emptying and food intake by measuring Fos-like expression in hindbrain, which reflects the activity of the vagus (58). Further injection of CCK or infusion of oleate causes less inhibition of gastric emptying in rats on a high fat diet (59). On the other hand, obese patients put on a caloric restriction (very low calories diet) for four days showed enhanced pyloric pressures and reduced antroduodenal motility suggesting a regained sensitivity to CCK. In obese patients the suppression of ghrelin and stimulation of PYY levels by a high-fat meal is blunted. Caloric restriction reversed the changes seen in obesity, restoring the inhibition of ghrelin and the stimulation of PYY after a high-fat meal (60). Similarly, one year of diet restriction on twenty-eight obese patients regained the effect of hormones on gastric emptying while the one month diet restriction did not cause these changes (61). Overall, these studies suggest obesity and high fat diet induces decreased CCK sensitivity in vagal afferent neurons and this seems to be reversed with caloric restriction.
In a recent study, Baudry et al. evaluated the pathophysiology behind accelerating gastric emptying in obese mice. The authors studied the effect of a Western diet on the enteric nervous system (ENS) phenotype. Mice were put on either normal diet or Western diet for fourteen weeks and assessed for gastrointestinal motility, leptin as well as glial cell line derived neurotrophic factor (GDNF) expression. Mice on normal diet showed a decrease in antral nitregic neurons while these findings were not observed in mice on Western diet. The authors also found that mice on Western diet have increased level of leptin and GDNF in the antrum. These findings can explain the faster gastric emptying observed in obese patients. This study is one of the first studies in this field to explain the pathophysiology of increased gastric emptying in obese patients (62).
Recently, certain genes have been implicated in gastric motility regulation and the sensitivity to hormones in obesity. Papathanasopoulos et al. studied the genetic reason of the variation of gastric function in obese patients. A group of obese and overweight humans were tested for multiple candidate genes controlling serotinergic or adrenergic function like ADRA2A, ADR2C, ADRB3, GNB3, and SLC6A4. The patients then underwent gastric function tests, which include gastric emptying by scintigraphy, gastric volume by SPECT and maximal tolerant capacity. They found that there is an association between specific groups of genes and gastric function, for e.g., ADR2C was associated with accelerated solid gastric empting; GNB3 and ADRA2A with decreased post-prandial gastric volume. The authors conclude that polymorphisms in genes such as ADR2C, UCP-3, GNB3 and FTO are associated with an intermediate gastric phenotype in obesity (63).
Novel ways to treat obesity
The knowledge of the link between GI motility and obesity can aid physicians in the treatment of obesity. Gastric electrical stimulation is a novel method in treating obesity, which has been studied for a long time. It includes inserting electrodes either in the stomach or duodenum to provoke gastric reverse contractions which cause delayed gastric emptying and also a more rapid intestinal transit, which can lead to weight loss. Dogs, pigs, rats and humans have similar results in prolonging gastric emptying and shortening the intestinal transit time with electrical stimulation (64-66).
Obesity and small intestinal motility
Small intestinal transit time and obesity
Intestinal transit time is an important factor to determine the amount of nutrients to be absorbed throughout the intestine. Prolonged transit time will lead to more absorption and weight gain. Conversely, shorter transit time means less time allowed for absorption and consequently weight loss. There are few studies evaluating the effect of obesity and diet on intestinal transit time and motility. Small intestine transit time can be measured by breath test or scintigraphy, but food needs to be delivered past the pylorus to accurately measure small intestine transit time. Otherwise the test will measure transit time from the mouth to the cecum, which includes gastric emptying and is called the orocecal transit time. There is a new technique to measure regional intestinal transit time and gastric emptying using a wireless capsule yet it has not been used to assess changes in small intestinal motility in obese patients (67). Wisen et al. studied the absorption rate in terms of calories and transit time using multiple radio labeled markers in massively obese patients and found that obese patients had significantly more absorption in the proximal intestine yet had a shorter intestinal transit time in comparison with normal weight people. The rapid absorption in the small intestine can lead to decreased satiety signals and thereby influence small intestinal motility (68). In a study in humans, using resected segments from small intestine from obese patients during Roux- en-Y surgery or in normal people during surgeries to resect benign polyps, they found intestinal segments from obese patients tend to have increased carbachol-induced smooth muscle contractility compared to normal patients, which can explain the faster transit in the proximal intestine in obesity (69). Orocecal transit time was found to be shorter in patients on high-fat diet for 14 days compared to patients on low-fat diet. This was associated with a shorter gastric emptying time. The authors conclude that the intestine has decreased sensitivity to regulatory mechanism such as gut hormones, CCK and PYY by exposure to high-fat diet but in this study it is not possible to determine which part of GI tract has this effect since gastric emptying was also shorter. CCK is known to inhibit gastric emptying and stimulate small intestinal motility (70). Increased nutrient content in the high fat diet, leads to intestinal adaption such as cell proliferation, increased intestinal length and nutrient uptake. This leads to reduced exposure of the intestinal field of receptors that are activated by fat such as the fat stimulated ileal mechanisms leading to slower gastric empting and small intestinal transit. In the absence of these mechanisms the gastric emptying and proximal small intestinal transit are more rapid (71). Some studies suggest a longer small intestinal transit time in obesity. Rats fed on cafeteria diet have been shown to have longer overall transit time and increased length of small intestine (72). In another study, the orocecal transit time was measured using breath test and is found to be prolonged in obese patients comparing to healthy volunteers (73). However in a small study, no difference could be detected between obese and normal individuals in gastric emptying and orocecal transit (74).
Mechanism of altered small bowel motility in obesity
In a study using leptin deficient mice (Lepob) mice by Kiely et al. they found that, although the proximal small intestinal transit in these mice was increased, the overall transit was decreased. This was felt to be due to increased jejunal response to CCK. In the same study the mice were given CCK and ACh and they found to have increased jejunum motility response to CCK in Lepob mice, which reflected a higher sensitivity to CCK (75). In another study Lepob mice were injected with leptin for four weeks, jejunum and ileum were isolated and motility tested with CCK and ACh stimulation. They found increased response to CCK but not ACh in Lepob mice that were injected with leptin and they concluded the synergistic action between leptin and CCK on small intestine motility (76). There are also changes in the absorption rates of specific nutrients in obese patients; Hyland et al. studied the adaptation of small intestine to high-fat diet in diet resistant and DIO rats and the DIO rats exhibit decreased intestinal glucose absorption. Decreased secretomotor responses in the jejunum were noted in DIO rats and felt to be due to reduced submucosal nerve activity leading to decreased glucose absorption as a possible adaptive response. This could also be a result of the higher levels of leptin in these mice and serve to decrease absorption of glucose as a regulatory mechanism (77). Mizuno et al. examined the nNOS neurons in the ileum of female ob/ob mice and found a significant reduction in these neurons. In addition there was increased expression of the purinergic receptor P2X2 (78).
Small intestinal motility patterns and obesity
The small intestine has two types of motility patterns: fasting pattern which has three phases and occur in cyclical style and is responsible for clearing the upper gut and food residues, and fed pattern which has unpredictable style similar to phase II and serves to transit the food at a rate that gives enough time for proper digestion and absorption. Upon eating the gut switches to the fed pattern after receiving signals from afferent vagal and gut hormones like CCK and GLP-1. Obese patients are thought to have different fasting patterns of motility that include decreased motilin levels and reduced phase III activity (79). In a study by Hyland et al. the jejunum response to veratridine, which is a drug that increase nerve excitability, was different in diet-induced obese rats. Compared to diet resistant rats, the obese rats have increased phase I, decreased phase-II and sustained increased in phase III in jejunum motility (77). CCK injection in ob/ob mice had a higher effect on motility in this group compared to normal mice (75). Bertrand et al. studied serotonin availability in the rat intestine fed a high-fat diet and found a higher serotonin level due to increased synthesis and reduced uptake of serotonin, which can explain increased intestinal motility in obese patients (80). The cannabinoid receptor, CB1, has been identified in the enteric nervous system and its stimulation leads to reduced smooth muscle contractility (81). There are studies on DIO mice that showed decreased the levels of Anandamide, an endogenous cannabinoid neurotransmitter in the small intestine, and also decreased response to cannabinoid receptors CB1 antagonist and that can explain the increased motility in these mice (82). Another factor that can affect the motility is the different intestinal bacterial growth pattern in obese patients. A recent study showed obese patients have a higher incidence of small intestinal bacterial overgrowth and these finding were associated with increased pattern of cluster contractions but no differences were noted in the in the fasting motility pattern. Clustered contractions are more common in pathologic conditions such as irritable bowel syndrome (IBS) and partial small bowel obstruction (83). Some studies relating to improvement in intestinal motility with electrical stimulation have been suggested. Intestinal electrical stimulation was found to accelerate the intestinal transit via activating the vagus afferent nerves in pigs and rats (64,84).
In summary, the majority of studies found increased proximal gut transit, increased small intestinal contractility and decreased distal gut transit in obesity. In obesity the presence of leptin resistance can alter CCK sensitivity leading to slower distal small intestinal transit. In addition, there is an evidence of more efficient nutrient absorption in obese patients and also an adaptation response to high-fat diet by decreasing glucose transporters.
Changes in colonic motility in obesity
In an adult population the frequency of constipation was assessed in 966 obese patients and was found to be significantly higher compared to people with normal weights (85). In a study involving children there was increased prevalence of constipation in obese children; 21% in obese children comparing to 8.8% in lean children have constipation with delayed total colonic transit time in a minority of obese children (86). Bertrand et al. found less availability of serotonin in the colon of high fat diet-induced obese rats and this was due to lower number of enterochromic cells and can explain high-fat diet induced constipation (87). Postprandial colonic response was studied after a high carbohydrate or high fat meal in normal individuals and both meals caused increased colonic motility measured by sensors in the colon, however the fat induced response was stronger and induced retrograde phasic contractions that may contribute to delayed colonic transit seen with high fat diets. This is called gastrocolic reflex and seems to be based on the diet type (88).
Other studies have also shown that there is an increased prevalence of diarrhea in obese patients (89,90). A study assessing the changes in colonic sensory and motor function associated with obesity showed that obese patients tend to have higher pain threshold and decreased compliance to inflated barostat in the colon. They also have shorter transit time in colon measured by scintigraphy. Shorter transit time can be an explanation for increased diarrhea prevalence between obese patients (91).
In summary the studies on obesity and colonic motility are limited. Future studies will need to examine the relationship between high fat diet, obesity and changes in the brain-gut axis. In vitro studies suggest apoptosis of rodent enteric neurons in the presence of saturated fatty acids (Srinivasan et al., unpublished data), however the mechanisms underlying this need to be further explored. Recently enteric neurons in the murine colon have been shown to display SIRT1 immunoreactivity (92). SIRT1 may have a role in regulating gastrointestinal motility in obesity. The role of bacterial products in regulating gastrointestinal motility has been of recent interest. We recently demonstrated the role of Toll like receptor-4 in regulating gastrointestinal motility (93). More studies need to be performed to understand the role of sirtuins and toll like receptors in obesity-induced changes in gastrointestinal motility.
Obesity has a multitude of effects on the entire human body. In this review, we have outlined the current knowledge on the gastrointestinal motility disturbances in obesity. Figure 1 summarizes the changes in gastrointestinal motility seen in obesity. Some diseases such as GERD have a clear association with obesity. Enhanced gastric emptying has also been noted in obese patients and this could be both a cause and consequence of obesity. Finally studies on the changes in the intestinal transit are limited but suggest an enhanced upper intestinal motility and a delayed distal small intestine and colonic motility in obesity. The mechanisms underlying the changes in gastrointestinal motility in obesity are complex and include alteration in sensitivity to CCK, changes in receptors such as CB1 and alteration in enteric neuronal numbers. Future studies will have to explore the role of microbiota in the modulation of gastrointestinal motility in obesity.
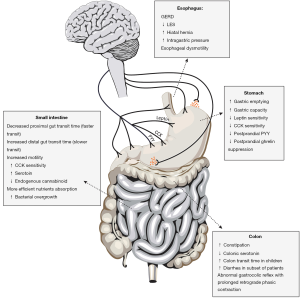
Acknowledgements
This work is supported by the NIH-RO1 (DK080684, SS) and VA-MERIT award (SS).
Disclosure: The authors declare no conflict of interest.
References
- Kopelman PG. Obesity as a medical problem. Nature 2000;404:635-43. [PubMed]
- Ogden CL, Carroll MD, Kit BK, et al. Prevalence of obesity in the United States, 2009-2010. NCHS Data Brief 2012;(82):1-8.
- Yanovski SZ, Yanovski JA. Obesity prevalence in the United States--up, down, or sideways? N Engl J Med 2011;364:987-9. [PubMed]
- Xing J, Chen JD. Alterations of gastrointestinal motility in obesity. Obes Res 2004;12:1723-32. [PubMed]
- Camilleri M, Grudell AB. Appetite and obesity: a gastroenterologist’s perspective. Neurogastroenterol Motil 2007;19:333-41. [PubMed]
- Jacobson BC, Somers SC, Fuchs CS, et al. Body-mass index and symptoms of gastroesophageal reflux in women. N Engl J Med 2006;354:2340-8. [PubMed]
- Nilsson M, Johnsen R, Ye W, et al. Obesity and estrogen as risk factors for gastroesophageal reflux symptoms. JAMA 2003;290:66-72. [PubMed]
- Friedenberg FK, Xanthopoulos M, Foster GD, et al. The association between gastroesophageal reflux disease and obesity. Am J Gastroenterol 2008;103:2111-22. [PubMed]
- Corley DA, Kubo A, Zhao W. Abdominal obesity, ethnicity and gastro-oesophageal reflux symptoms. Gut 2007;56:756-62. [PubMed]
- Schneider JM, Brücher BL, Küper M, et al. Multichannel intraluminal impedance measurement of gastroesophageal reflux in patients with different stages of morbid obesity. Obes Surg 2009;19:1522-9. [PubMed]
- Wu JC, Mui LM, Cheung CM, et al. Obesity is associated with increased transient lower esophageal sphincter relaxation. Gastroenterology 2007;132:883-9. [PubMed]
- Kouklakis G, Moschos J, Kountouras J, et al. Relationship between obesity and gastroesophageal reflux disease as recorded by 3-hour esophageal pH monitoring. Rom J Gastroenterol 2005;14:117-21. [PubMed]
- Pandolfino JE, El-Serag HB, Zhang Q, et al. Obesity: a challenge to esophagogastric junction integrity. Gastroenterology 2006;130:639-49. [PubMed]
- Chak A, Falk G, Grady WM, et al. Assessment of familiality, obesity, and other risk factors for early age of cancer diagnosis in adenocarcinomas of the esophagus and gastroesophageal junction. Am J Gastroenterol 2009;104:1913-21. [PubMed]
- Lagergren J, Bergström R, Nyrén O. Association between body mass and adenocarcinoma of the esophagus and gastric cardia. Ann Intern Med 1999;130:883-90. [PubMed]
- Wu AH, Tseng CC, Bernstein L. Hiatal hernia, reflux symptoms, body size, and risk of esophageal and gastric adenocarcinoma. Cancer 2003;98:940-8. [PubMed]
- Wilson LJ, Ma W, Hirschowitz BI. Association of obesity with hiatal hernia and esophagitis. Am J Gastroenterol 1999;94:2840-4. [PubMed]
- Biccas BN, Lemme EM, Abrahão LJ Jr, et al. Higher prevalence of obesity in erosive gastroesophageal reflux disease. Arq Gastroenterol 2009;46:15-9. [PubMed]
- Hong D, Khajanchee YS, Pereira N, et al. Manometric abnormalities and gastroesophageal reflux disease in the morbidly obese. Obes Surg 2004;14:744-9. [PubMed]
- Jaffin BW, Knoepflmacher P, Greenstein R. High prevalence of asymptomatic esophageal motility disorders among morbidly obese patients. Obes Surg 1999;9:390-5. [PubMed]
- Fornari F, Callegari-Jacques SM, Dantas RO, et al. Obese patients have stronger peristalsis and increased acid exposure in the esophagus. Dig Dis Sci 2011;56:1420-6. [PubMed]
- Djärv T, Wikman A, Nordenstedt H, et al. Physical activity, obesity and gastroesophageal reflux disease in the general population. World J Gastroenterol 2012;18:3710-4. [PubMed]
- Fass R. The pathophysiological mechanisms of GERD in the obese patient. Dig Dis Sci 2008;53:2300-6. [PubMed]
- Hellström PM, Grybäck P, Jacobsson H. The physiology of gastric emptying. Best Pract Res Clin Anaesthesiol 2006;20:397-407. [PubMed]
- Vanden Berghe P, Janssen P, Kindt S, et al. Contribution of different triggers to the gastric accommodation reflex in humans. Am J Physiol Gastrointest Liver Physiol 2009;297:G902-6. [PubMed]
- Bratten J, Jones MP. New directions in the assessment of gastric function: clinical applications of physiologic measurements. Dig Dis 2006;24:252-9. [PubMed]
- Ang D. Measurement of gastric accommodation: a reappraisal of conventional and emerging modalities. Neurogastroenterol Motil 2011;23:287-91. [PubMed]
- de Zwart IM, Haans JJ, Verbeek P, et al. Gastric accommodation and motility are influenced by the barostat device: Assessment with magnetic resonance imaging. Am J Physiol Gastrointest Liver Physiol 2007;292:G208-14. [PubMed]
- Kim DY, Camilleri M, Murray JA, et al. Is there a role for gastric accommodation and satiety in asymptomatic obese people? Obes Res 2001;9:655-61. [PubMed]
- Geliebter A, Hashim SA. Gastric capacity in normal, obese, and bulimic women. Physiol Behav 2001;74:743-6. [PubMed]
- Mejía-Rivas M, Remes-Troche J, Montaño-Loza A, et al. Gastric capacity is relatedto body mass index in obese patients.A study using the water load test. Rev Gastroenterol Mex 2009;74:71-73. [PubMed]
- Breen M, Camilleri M, Burton D, et al. Performance characteristics of the measurement of gastric volume using single photon emission computed tomography. Neurogastroenterol Motil 2011;23:308-15. [PubMed]
- Zahorska-Markiewicz B, Jonderko K, Lelek A, et al. Gastric emptying in obesity. Hum Nutr Clin Nutr 1986;40:309-13. [PubMed]
- Wright RA, Krinsky S, Fleeman C, et al. Gastric emptying and obesity. Gastroenterology 1983;84:747-51. [PubMed]
- Tosetti C, Corinaldesi R, Stanghellini V, et al. Gastric emptying of solids in morbid obesity. Int J Obes Relat Metab Disord 1996;20:200-5. [PubMed]
- Maddox A, Horowitz M, Wishart J, et al. Gastric and oesophageal emptying in obesity. Scand J Gastroenterol 1989;24:593-8. [PubMed]
- Vazquez Roque MI, Camilleri M, Stephens DA, et al. Gastric sensorimotor functions and hormone profile in normal weight, overweight, and obese people. Gastroenterology 2006;131:1717-24. [PubMed]
- Cardoso-Júnior A, Coelho LG, Savassi-Rocha PR, et al. Gastric emptying of solids and semi-solids in morbidly obese and non-obese subjects: an assessment using the 13C-octanoic acid and 13C-acetic acid breath tests. Obes Surg 2007;17:236-41. [PubMed]
- Di Ciaula A, Wang DQ, Portincasa P. Gallbladder and gastric motility in obese newborns, pre-adolescents and adults. J Gastroenterol Hepatol 2012;27:1298-305. [PubMed]
- Buchholz V, Berkenstadt H, Goitein D, et al. Gastric emptying is not prolonged in obese patients. Surg Obes Relat Dis 2012. [Epub ahead of print]. [PubMed]
- Li J, Ma W, Wang S. Slower gastric emptying in high-fat diet induced obese rats is associated with attenuated plasma ghrelin and elevated plasma leptin and cholecystokinin concentrations. Regul Pept 2011;171:53-7. [PubMed]
- Asakawa A, Inui A, Ueno N, et al. Ob/ob mice as a model of delayed gastric emptying. J Diabetes Complications 2003;17:27-8. [PubMed]
- Camilleri M. Peripheral mechanisms in the control of appetite and related experimental therapies in obesity. Regul Pept 2009;156:24-7. [PubMed]
- Delgado-Aros S, Kim DY, Burton DD, et al. Effect of GLP-1 on gastric volume, emptying, maximum volume ingested, and postprandial symptoms in humans. Am J Physiol Gastrointest Liver Physiol 2002;282:G424-31. [PubMed]
- Schirra J, Wank U, Arnold R, et al. Effects of glucagon-like peptide-1(7-36)amide on motility and sensation of the proximal stomach in humans. Gut 2002;50:341-8. [PubMed]
- Stewart JE, Feinle-Bisset C, Keast RS. Fatty acid detection during food consumption and digestion: Associations with ingestive behavior and obesity. Prog Lipid Res 2011;50:225-33. [PubMed]
- Little TJ, Russo A, Meyer JH, et al. Free fatty acids have more potent effects on gastric emptying, gut hormones, and appetite than triacylglycerides. Gastroenterology 2007;133:1124-31. [PubMed]
- Feltrin KL, Little TJ, Meyer JH, et al. Effects of intraduodenal fatty acids on appetite, antropyloroduodenal motility, and plasma CCK and GLP-1 in humans vary with their chain length. Am J Physiol Regul Integr Comp Physiol 2004;287:R524-33. [PubMed]
- Castiglione KE, Read NW, French SJ. Adaptation to high-fat diet accelerates emptying of fat but not carbohydrate test meals in humans. Am J Physiol Regul Integr Comp Physiol 2002;282:R366-71. [PubMed]
- Rotondo A, Amato A, Baldassano S, et al. Gastric relaxation induced by glucagon-like peptide-2 in mice fed a high-fat diet or fasted. Peptides 2011;32:1587-92. [PubMed]
- Little TJ, Feltrin KL, Horowitz M, et al. A high-fat diet raises fasting plasma CCK but does not affect upper gut motility, PYY, and ghrelin, or energy intake during CCK-8 infusion in lean men. Am J Physiol Regul Integr Comp Physiol 2008;294:R45-51. [PubMed]
- Boyd KA, O’Donovan DG, Doran S, et al. High-fat diet effects on gut motility, hormone, and appetite responses to duodenal lipid in healthy men. Am J Physiol Gastrointest Liver Physiol 2003;284:G188-96. [PubMed]
- de Lartigue G, Barbier de la Serre C, Espero E, et al. Diet-induced obesity leads to the development of leptin resistance in vagal afferent neurons. Am J Physiol Endocrinol Metab 2011;301:E187-95. [PubMed]
- de Lartigue G, Barbier de la Serre C, Espero E, et al. Leptin resistance in vagal afferent neurons inhibits cholecystokinin signaling and satiation in diet induced obese rats. PLoS One 2012;7:e32967. [PubMed]
- Daly DM, Park SJ, Valinsky WC, et al. Impaired intestinal afferent nerve satiety signalling and vagal afferent excitability in diet induced obesity in the mouse. J Physiol 2011;589:2857-70. [PubMed]
- Swartz TD, Duca FA, Covasa M. Differential feeding behavior and neuronal responses to CCK in obesity-prone and -resistant rats. Brain Res 2010;1308:79-86. [PubMed]
- Sayegh AI, Covasa M, Ritter RC. Intestinal infusions of oleate and glucose activate distinct enteric neurons in the rat. Auton Neurosci 2004;115:54-63. [PubMed]
- Covasa M, Grahn J, Ritter RC. Reduced hindbrain and enteric neuronal response to intestinal oleate in rats maintained on high-fat diet. Auton Neurosci 2000;84:8-18. [PubMed]
- Covasa M, Ritter RC. Adaptation to high-fat diet reduces inhibition of gastric emptying by CCK and intestinal oleate. Am J Physiol Regul Integr Comp Physiol 2000;278:R166-70. [PubMed]
- Brennan IM, Seimon RV, Luscombe-Marsh ND, et al. Effects of acute dietary restriction on gut motor, hormone and energy intake responses to duodenal fat in obese men. Int J Obes (Lond) 2011;35:448-56. [PubMed]
- Mathus-Vliegen EM, van Ierland-van Leeuwen ML, Bennink RJ. Influences of fat restriction and lipase inhibition on gastric emptying in obesity. Int J Obes (Lond) 2006;30:1203-10. [PubMed]
- Baudry C, Reichardt F, Marchix J, et al. Diet-induced obesity has neuroprotective effects in murine gastric enteric nervous system: involvement of leptin and glial cell line-derived neurotrophic factor. J Physiol 2012;590:533-44. [PubMed]
- Papathanasopoulos A, Camilleri M, Carlson PJ, et al. A preliminary candidate genotype-intermediate phenotype study of satiation and gastric motor function in obesity. Obesity (Silver Spring) 2010;18:1201-11. [PubMed]
- Xu X, Lei Y, Chen JD. Duodenum electrical stimulation delays gastric emptying, reduces food intake and accelerates small bowel transit in pigs. Obesity (Silver Spring) 2011;19:442-8. [PubMed]
- Zhang J, Xu X, Chen JD. Chronic tachygastrial electrical stimulation reduces food intake in dogs. Obesity (Silver Spring) 2007;15:330-9. [PubMed]
- Yin J, Zhang J, Chen JD. Inhibitory effects of intestinal electrical stimulation on food intake, weight loss and gastric emptying in rats. Am J Physiol Regul Integr Comp Physiol 2007;293:R78-82. [PubMed]
- Sarosiek I, Selover KH, Katz LA, et al. The assessment of regional gut transit times in healthy controls and patients with gastroparesis using wireless motility technology. Aliment Pharmacol Ther 2010;31:313-22. [PubMed]
- Wisén O, Johansson C. Gastrointestinal function in obesity: motility, secretion, and absorption following a liquid test meal. Metabolism 1992;41:390-5. [PubMed]
- Gallagher TK, Baird AW, Winter DC. Constitutive basal and stimulated human small bowel contractility is enhanced in obesity. Ann Surg Innov Res 2009;3:4. [PubMed]
- Wang X, Soltesz V, Axelson J, et al. Cholecystokinin increases small intestinal motility and reduces enteric bacterial overgrowth and translocation in rats with surgically induced acute liver failure. Digestion 1996;57:67-72. [PubMed]
- Cunningham KM, Daly J, Horowitz M, et al. Gastrointestinal adaptation to diets of differing fat composition in human volunteers. Gut 1991;32:483-6. [PubMed]
- Dameto MC, Rayó JM, Esteban S, et al. Effect of cafeteria diet on the gastrointestinal transit and emptying in the rat. Comp Biochem Physiol A Comp Physiol 1991;99:651-5.
- Basilisco G, Camboni G, Bozzani A, et al. Orocecal transit delay in obese patients. Dig Dis Sci 1989;34:509-12. [PubMed]
- French SJ, Murray B, Rumsey RD, et al. Preliminary studies on the gastrointestinal responses to fatty meals in obese people. Int J Obes Relat Metab Disord 1993;17:295-300. [PubMed]
- Kiely JM, Noh JH, Graewin SJ, et al. Altered intestinal motility in leptin-deficient obese mice. J Surg Res 2005;124:98-103. [PubMed]
- Kiely JM, Graewin SJ, Pitt HA, et al. Leptin increases small intestinal response to cholecystokinin in leptin-deficient obese mice. J Surg Res 2005;124:146-50. [PubMed]
- Hyland NP, Rybicka JM, Ho W, et al. Adaptation of intestinal secretomotor function and nutrient absorption in response to diet-induced obesity. Neurogastroenterol Motil 2010;22:602-e171. [PubMed]
- Mizuno MS, Crisma AR, Borelli P, et al. Expression of the P2X(2) receptor in different classes of ileum myenteric neurons in the female obese ob/ob mouse. World J Gastroenterol 2012;18:4693-703. [PubMed]
- Pieramico O, Malfertheiner P, Nelson DK, et al. Interdigestive gastroduodenal motility and cycling of putative regulatory hormones in severe obesity. Scand J Gastroenterol 1992;27:538-44. [PubMed]
- Bertrand RL, Senadheera S, Markus I, et al. A Western diet increases serotonin availability in rat small intestine. Endocrinology 2011;152:36-47. [PubMed]
- Boesmans W, Ameloot K, van den Abbeel V, et al. Cannabinoid receptor 1 signalling dampens activity and mitochondrial transport in networks of enteric neurones. Neurogastroenterol Motil 2009;21:958-e77. [PubMed]
- Izzo AA, Piscitelli F, Capasso R, et al. Peripheral endocannabinoid dysregulation in obesity: relation to intestinal motility and energy processing induced by food deprivation and re-feeding. Br J Pharmacol 2009;158:451-61. [PubMed]
- Madrid AM, Poniachik J, Quera R, et al. Small intestinal clustered contractions and bacterial overgrowth: a frequent finding in obese patients. Dig Dis Sci 2011;56:155-60. [PubMed]
- Sun Y, Chen JD. Intestinal electric stimulation accelerates whole gut transit and promotes fat excrement in conscious rats. Int J Obes (Lond) 2009;33:817-23. [PubMed]
- Pecora P, Suraci C, Antonelli M, et al. Constipation and obesity: a statistical analysis. Boll Soc Ital Biol Sper 1981;57:2384-8. [PubMed]
- vd Baan-Slootweg OH, Liem O, Bekkali N, et al. Constipation and colonic transit times in children with morbid obesity. J Pediatr Gastroenterol Nutr 2011;52:442-5.
- Bertrand RL, Senadheera S, Tanoto A, et al. Serotonin availability in rat colon is reduced during a Western diet model of obesity. Am J Physiol Gastrointest Liver Physiol 2012;303:G424-34. [PubMed]
- Rao SS, Kavelock R, Beaty J, et al. Effects of fat and carbohydrate meals on colonic motor response. Gut 2000;46:205-11. [PubMed]
- You S, Zhang Q, Anitha M, et al. Effects of hepatic insulin gene therapy on enteric neuropathy in STZ-diabetic mice. Zhong Nan Da Xue Xue Bao Yi Xue Ban 2011;36:546-53. [PubMed]
- Delgado-Aros S, Locke GR 3rd, Camilleri M, et al. Obesity is associated with increased risk of gastrointestinal symptoms: a population-based study. Am J Gastroenterol 2004;99:1801-6. [PubMed]
- Delgado-Aros S, Camilleri M, Garcia MA, et al. High body mass alters colonic sensory-motor function and transit in humans. Am J Physiol Gastrointest Liver Physiol 2008;295:G382-8. [PubMed]
- Lakhan SE, Kirchgessner A. Gut microbiota and sirtuins in obesity-related inflammation and bowel dysfunction. J Transl Med 2011;9:202. [PubMed]
- Anitha M, Vijay-Kumar M, Sitaraman SV, et al. Gut Microbial Products Regulate Murine Gastrointestinal Motility via Toll-Like Receptor 4 Signaling. Gastroenterology 2012;143:1006-16. [PubMed]