Mesenchymal stem cells as future treatment for cardiovascular regeneration and its challenges
Introduction
Cardiovascular diseases (CVDs), a type of chronic diseases principally ischemic heart disease (IHD), for instance stroke and myocardial infarction (MI) have high morbidity and mortality rate which contributed to the disability-adjusted life, death and global disease burden (1,2). It is an ever-growing healthcare problem accounting for a total of 32% of global deaths and taking approximately 17.9 million of lives annually (3). The global death figure is projected to be 22.2 million deaths annually by the year of 2030 (4). The overall healthcare burden caused by CVDs is estimated to increase from 318 million US dollars (USD) to 1.1 trillion USD by the year of 2035, which underscored the healthcare burden today (5).
CVD is an umbrella term that encompasses a broad range of pathologies including cerebrovascular disease, MI, stroke, cardiomyopathy, peripheral heart diseases, coronary heart disease, rheumatic heart diseases as well as congenital heart diseases (1,6,7). The underlying predominant pathogenesis associated with most of the CVDs is due to atherosclerotic origin caused by blood clots and fat accumulation on the lining of blood vessels (1,8). The development of atherosclerotic plaques caused the narrowing of arteries, thereby stopping the blood flow to other parts of the heart that resulted in the damage and death of heart muscle (9). Hence, hyperlipidaemia, hypertension, diabetes, smoking and obesity are well recognized aetiological factors leading to the onset of CVDs (7).
Current available treatment approaches in pharmacological aspects include antithrombotic agents, antiplatelet agents, and lipid-lowering agents (10). Most of the time surgeries are required, for instances: coronary angioplasty with the insertion of a stent to normalize the blood flow or implanting of mechanical ventricular assist devices (11,12). Nevertheless, these approaches are associated with high medical expenses and other complications such as bleeding and infection (11). The improvements in managing CVDs through pharmacological and surgical approaches have lowered the mortality rate of CVDs, but they merely served as symptomatic treatments. However, the progression of CVDs with significant morbidities is still inevitable (13). CVDs particularly MI, due to the irreversibly loss of function of myocardial cells that lead to heart failure (14), which cannot save by pharmacological and surgical approaches. To date, heart transplantation is still the standard curative treatment for heart failure, the terminal-stage of CVDs. The scarcity of donor organs, high operation costs and requirement of lifelong immunosuppression of post-transplantation have limited the development of this approach (5). Hence, stem cell approaches have gained a great attention to emerge as promising and alternative future treatment since a decade ago (15).
The therapeutic approach of stem cell therapy emerged over two decades ago in animal studies (16) and further progressed to clinical studies 10 years later (17). Stem cells could be classified as adult stem cells (ASCs) or embryonic stem cells (ESCs) (18). ESCs can differentiate into an embryonic structure which is also known as pluripotent in nature. On the other hand, ASCs showed its multipotency in which they only differentiate within a limited range of cell types (19) as a regeneration of damaged tissue. Mesenchymal stem cells (MSCs) categorized as a type of ASCs which were discovered by Friedenstein et al. in 1970 (20) have drawn a great consideration in clinical studies due to MSCs free from ethical concerns as well as teratoma formation (18). Bone marrow, adipose tissues, and umbilical cord are the source of MSCs. Furthermore, MSCs are able to differentiate into multiple lineages which include osteocytes, myocytes, chondrocytes, adipocytes, and other lineages (21).
The capacity of heart tissue to undergo self-regeneration is limited as adult cardiac cells have less tendency to divide (13). Hence, this made the use of MSCs to repair the ischemic areas of the heart or assist in myocardial self-repair and restore the cardiac functions become feasible (22). MSCs particularly attractive for cardiovascular regeneration due to their multilineage potential, immunomodulatory properties, able to secrete cardio-protective cytokine, angiogenesis stimulation and extracellular matrix reorganization in ischemia scar areas (11,23). However, the application of MSCs had faced several challenges despite their attractive properties. The challenges included complexity and versatility of MSCs, low in vivo survival rate, efficiency of administration route as well as the induction of tumorigenicity (24,25). This article will focus on the therapeutic properties of MSCs, the use of MSCs for cardiovascular regeneration as well as tissue engineering of MSCs and the challenges in the use of MSCs.
Properties and characterization of MSCs
MSCs are mostly located in perivascular space or vascularized tissues that could be ubiquitously found throughout the human body (26). In general, MSCs represent the nonhematopoietic subpopulation of cells with the potential of multilineage differentiation which differentiate into different types of mesodermal tissues (25,27). The first identification of MSCs was back 40 years ago, a plastic-adherent bone marrow stromal cell which was isolated from bone marrow (20). However, the sources of human MSCs are not only restricted to bone marrow, adipose tissue (28), umbilical cord (29), cartilage (30), and peripheral blood (31), but also from other organs or tissues such as brain, spleen, liver and lung (1). However, the most widely utilized MSCs in clinical studies are isolated from bone marrow (15) as MSCs from bone marrow accounted for about 0.001% to 0.1% of plastic-adherent cells that are nucleated that along with easy isolation and in vitro amplification (6). MSCs are widely regarded as culture-adherent cells with the capacity of self-renewal to differentiate into multiple lineages such as adipogenic, chondrogenic, and osteogenic (32). It is also being reported that mesodermal cell types such as cardiomyocytes can be transdifferentiated by MSCs (33).
However, MSCs isolated from different sources would exhibit different characteristics, transcriptomic profile, and differentiation potential (34). Thus, the equivalence of biological activities of different sources of MSCs is worthwhile to be determined (15). Hence, due to heterogenous cell population of MSCs, characterization and isolation by varied among the researchers, the Mesenchymal and Tissue Stem Cells Committee of the International Society of Cellular Therapy (ISCT) had established the minimal criteria to fulfill the definition of MSCs to make the compare and contrast of study outcomes easier (35). Cells which are referred as MSCs must show the characteristics of plastic-adherent under the condition of standard culture. Secondly, the surface markers including CD73, CD105, and CD90 must be expressed by MSCs but not CD45, CD14, CD34, CD79α, CD19, CD11b, or HLA-DR surface markers when assessed by fluorescence-activated cell sorting (FACS) analysis. Thirdly, MSCs must possess the ability to retain the capacity of in vitro multilineage differentiation into adipocytes, chondrocytes as well as osteocytes (35,36). However, none of the cell surface markers could disputably describe MSCs. Furthermore, loss of cell surface marker expression may also be observed in the MSCs during the isolation or expansion process (37). Isolation of MSCs according to ISCT properties had resulted in stromal cells that are heterogenous and nonclonal cultural that contained various multipotent stem cells, committed as progenitors or differentiated stem cells (38). To reflect the heterogeneity of the cells, the nomenclature of ‘mesenchymal stromal cells’ had deviated out from ‘mesenchymal stem cells’. However, both of these nomenclatures are still being applied interchangeably (39).
MSCs are also being well recognized for its capacity of migration and homing efficiency which are regulated by the expression of adhesion molecules, integrin, chemokines receptors: CCR1, CCR4, CCR7, CCR10, and CXCR5 (27). The expression of these molecules had made MSCs play an important role in tissue regeneration by reaching the damaging tissues through endothelial cell layers (40). The physiological role of MSCs in maintaining tissue homeostasis had underscored a significant potential for the clinical uses as regenerative medicine such as heart regeneration, neuronal regeneration as well as skin wound healing repair (26). Thus, MSCs had gained a great attention as future treatment of chronic diseases especially CVDs due to its multilineage differentiation and homing efficiency. In addition, MSCs with the properties of cardiomyogenesis, immunomodulation, antifibrotic and neovasculogenesis had played a significant role other than differentiation ability in treating CVDs as described in Table 1.
Table 1
Properties | Findings | References |
---|---|---|
Cardiomyogenesis | • By exposing MSCs in vitro with DNA methyltransferase 5-Aza lead to the induction of cardiomyogenic differentiation of MSCs | (13,41-44) |
• Growth factors such as BMP-2, FGF-4, antioxidant supplements, insulin induce cardiomyocyte-like cells from MSCs | ||
• miRNA-1–2 can regulate the differentiation of MSCs to cardiomyocyte-like cells via Wnt signaling pathway | ||
• MSCs showed the differentiation ability cardiac muscle-like cells in vivo after transplantation of MSCs into damaged site of myocardial tissue | ||
• Animal studies have shown MSCs exhibit cardiomyogenesis and engraftment up to 3 years, showing the ability to contribute to cardiac tissue over extended period | ||
Anti-inflammatory and immunomodulatory properties | • MSCs regulate the inflammatory response by suppressing leukocytes, both innate and adaptive immunity anti-inflammation | (45-47) |
• The number of proinflammatory monocytes decreased with the transplantation of MSCs and reduce myocarditis severity after MI | ||
• MSCs can inhibit proliferation of T cell which suitable in treating graft-versus-host diseases | ||
• MSCs transplantation on MI mice show reduction in expression of TNF-α, IL-1, and IL-6 of myocardial cells and resulted in cardiac function improvement | ||
Antifibrotic effects | • MSCs can regulate MMP to inhibit the proliferation of cardiac fibroblast collagen type I and type III are decreased in expression | (48-50) |
• Excess deposition of collagen interrupted the interaction of between myocytes in myocardium and leading to myocardium | ||
• Adipose-derived MSCs showed the ability to produce fibrosis regulator such as TGF-β1 to impact the fibrosis evolution | ||
• Paracrine effect of MSCs that caused antifibrotic effect resulted in reduced left-ventricular remodeling and improved cardiac function | ||
Neovascularization capacity | • Paracrine mechanism of MSCs showed angiogenic potential by secreting pro-angiogenic molecules, VEGFs | (6,51-53) |
• Wide array of cytokine secreted by MSCs including PDGF, thrombopoietin and angiogenin to exert angiogenesis | ||
• Studies also showed that under hypoxia-preconditioned MSCs stimulate the expression of VEFG, bFGF, IGF-1, and SDF-1 which served as an important factor for neovascularization process. The process included mobilizing, migrating, homing, adhering and differentiating to endothelial cells |
MSC, mesenchymal stem cell; CVD, cardiovascular disease; 5-Aza, 5-azacytidine; BMP-2, bone morphogenetic protein-2; FGF-4, fibroblast growth factor-4; miRNA, microRNA; MI, myocardial infarction; TNF-α, tumor necrosis factor-alpha; IL, interleukin; MMP, matrix metalloproteinase; TGF-β1, transforming growth factor-β1; VEGF, vascular endothelial growth factor; PDGF, platelet-derived growth factor; bFGF, basic fibroblast growth factor; IGF-1, insulin-like growth factor-1; SDF-1, stromal cell-derived factor-1.
MSCs transplantation for ischemic cardiomyopathy
Ischemic cardiomyopathy is commonly referred to the decreased ability of the heart to pump blood efficiently due to myocardial damage caused upon the ischemia (54). Coronary artery diseases (CADs) are always addressed along with atherosclerosis as these are the prevalent cause of ischemic cardiomyopathy (55). Characterization of CAD is due to plaque formation in the coronary blood vessels, which resulted in the cardiac muscle cells experiencing an imbalance of oxygen supply and demand. This eventually contributed to the irreversible damage of the cardiac muscle cells due to prolonged ischemia and resulted in the cardiac remodeling (54,56). Despite the significant advanced surgery treatment including coronary artery bypass grafting and stenting, there are still some existing issues such as a sharp reduced cardiac ejection fraction (EF) (<25%) after the surgery (57). Hence, MSCs had been proposed as one of the potential future treatments in a form of cellular therapy as cellular cardiomyoplasty (58). Cellular cardiomyoplasty is a cardiac regenerative medicine field, which uses stem cells or other biological substances that are grafted into the myocardium to improve the contractile ability as well as to hinder the reversing effect of scar formation (59). The application of MSCs as cellular cardiomyoplasty is due to the transdifferention of MSCs, paracrine functions, microvesicles, and exosomes as well as mitochondria transfer.
Transdifferentiation of MSCs under hypoxia condition
The past two decades had established a lot of studies regarding the application of MSCs to treat acute or chronic ischemic heart injury in rodents, murine or other larger animal models (25). The underlying mechanisms of the therapeutic effects of MSCs related to its properties including trans differentiation into cardiomyocytes or endothelial cell (60), paracrine mechanisms in secreting broad spectrum of cytokine (61), enhanced angiogenesis and myogenesis (62) as well as stimulating local cardiac stem cell (CSC) differentiation (63). According to the study done by Xie et al., in vitro differentiation of bone marrow-derived MSCs (BM-MSCs) was induced by myocardium medium under the condition of hypoxia. As the differentiation of MSCs might be associated with presence of soluble signaling molecules in the conditioned medium containing hypoxia-induced cardiomyocytes (64). This suggested that the implantation of MSCs to myocardium can differentiate under a milieu-dependent manner and express the cardiomyogenic phenotypes in vivo (65). According to the studies done by Nagaya et al., the administration of MSCs intravenously into rats model with acute MI demonstrated cardiac function improvement as a result of angiogenesis and myogenesis that occurred in ischemic myocardium (62). MSCs have the ability to stimulate endogenous CSCs in vivo and in vitro to proliferate (13). Furthermore, according to the study done by Williams et al. had reported the therapeutic effect of combining human MSCs and CSCs would reduce the infarct size after MI and restore the cardiac function (23).
Paracrine effect of MSCs
Although MSCs have cardiogenic transdifferentiation ability, the paracrine function of MSCs also predominantly accounts for the therapeutic effects of cellular cardiomyoplasty (66). The mounting evidence showed that the paracrine effect of MSCs by producing a plethora of factors such as growth factors, cytokine, surface molecules, messenger RNA (mRNA), and microRNA (miRNA) (61). All these factors are contributing to the recovery of cardiac function by assisting the endogenous repair mechanism as well as support antifibrosis, activity of antiapoptotic and inhibition of proinflammatory (13,25,61) as shown in Figure 1. These factors are vascular endothelial growth factor (VEGF), stromal cell-derived factor (SDF)-1, insulin-like growth factor (IGF)-1, angiopoietin 1, hepatocyte growth factor (HGF), periostin, and thymosin b4 (15,67,68). Studies also showed that hypoxia-induced MSCs which overexpress the prosurvival gene, Akt upregulated the gene encoded for IGF-1, HGF, fibroblast growth factor (FGF)-2, and VEGF. With this property, the in vitro studies of conditioned-medium from hypoxia-induced MSCs showed the inhibition of hypoxia caused apoptosis but stimulated the contraction of cardiomyocytes of rats. On the other hand, the in vivo study of conditioned medium injected to the myocardium of MI rat showed the improvement of cardiac function (69). MSCs cardioprotective effects are due to the ability of MSCs to promote cardiomyocytes survival and anti-apoptosis via the activation of protein kinase C (PKC), PI3K/protein kinase B (Akt), nuclear factor (NF)-κB, and STAT3 signaling (70). In terms of cardiovascular tissue repair, it is significant to minimize the fibrotic scarring to prevent cardiac tissue from stiffening to maintain its cardiac function (71). MSCs-secreting factors including matrix metallopeptidase (MMP)-9 and interleukin (IL)-6 are upregulated in MI patients which are responsible for the pathological cause of remodeling and proinflammatory responses which should be maintained at minimum level (70-72). However, MSCs are also able to produce the antagonists to inhibit these negative factors including tissue inhibitor of metalloproteinases (TIMP)-1 and IL-10 to alleviate the effects. Hence, this showed that MSCs have the ability to achieve an appropriate balance in terms of the stimulatory and inhibitory factors that were produced (73).
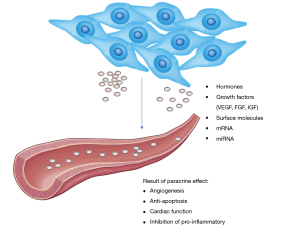
Microvesicles and exosomes
Recent studies also found that MSCs are able to secrete microvesicles known as exosomes, which is the membrane vesicle with the diameter of 30–100 nm (74). Lai et al. were the first to study MSCs-derived exosomes in the year 2010 in reducing myocardial ischemia injury. The findings found that a novel role of exosomes had brought a different perspective of the mediation of intercellular tissue repairing (75). The content presence in the exosomes was found to be proteins, lipids, cytokines, mRNA, miRNA, and ribosomal RNAs (76). Different content of the exosomes would lead to different therapeutic effects in treating CVDs as shown in Figure 2. The common surface markers are CD29, CD9, CD89, and CD44 which shown on both MSCs and exosomes (75). In the animal studies, the myocardial ischemia and reperfusion injury porcine model that treated with MSC-conditioned medium intravenously and intracoronary had shown the reduction of infarct size by 50% upon administration (76). This is due to the cardioprotective effect of MSCs which exert the paracrine effect by secreting exosomes to neutralize the injury (77). These exosomes had played an important role in treating CVDs with the ability of anti-apoptosis, anti-inflammatory, anti-fibrotic, immunomodulatory that lead to cardiac regeneration (74). Study reported that the ameliorative effects of MSCs on ischemic cardiomyocytes was due to the transferring of miR-22-containing exosomes which targeted on the methyl CpG binding protein 2 (Mecp2) and thereby reduce the activity of apoptosis (78). The anti-apoptotic effect is due to p53-upregulator modulator of apoptosis (PUMA) inhibition (79). In addition, a study done by Arslan et al. demonstrated exosomes derived from MSCS showed the anti-inflammatory and anti-cardiac remodeling effect. MSCs-secreting exosomes would lower the white blood cell count including neutrophils and macrophages and thereby alleviate the inflammation responses along with decrease in size of infarction and prevent cardiac remodeling after ischemia-reperfusion injury (IRI) (80). miRNA-223 MSCs-derived exosomes showed the reduction of inflammation response induced by macrophages and thereby result in cardioprotective effects (81). On the other hand, other content such as miR-133 will lead to anti-fibrotic effect (82), VEGF that stimulates angiogenesis (83), and IL-10 for immunomodulation (42).
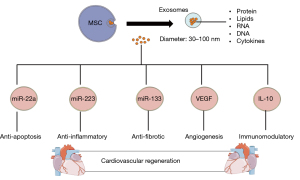
Mitochondria transfer
Mitochondria transfer is another method of the MSCs communicating intercellularly. It was being reported that the human MSCs can transfer their mitochondria to the damaged cells that lack of functional mitochondria that are incapable of carrying out aerobic respiration due to the deleted or defective mitochondrial DNA (84). The mechanism of intercellular transfer of mitochondria remains unknown and yet to be fully understood. However, it has been proposed that MSCs can actively transfer their mitochondria to damaged cell with severe compromised mitochondrial function via a tunnelling nanotube (TNT), a nanotubular structure in which comprised of F-actin and partial membrane fusion (85) as shown in Figure 3. According to the studies done by Lin et al., mitochondria transfer from MSCs to mitochondria-defective cells would benefit the damaged cells by restoring their mitochondrial function via the cellular bioenergetics rescue as well as restoration of impaired oxidative phosphorylation and (86). Studies also showed that the interplay between MSCs and cardiomyocytes to convey the functional mitochondria in providing a new therapeutics insight in CVDs (13). In a coculture system between human MSCs and adult rat cardiomyocytes, a heterologous cell fusion and mitochondria transfer were observed to promote the reprogramming or dedifferentiation of cardiomyocytes to a progenitor-like state. Hence, this has provided a better insight of stem-cell mediated regenerative mechanisms to develop a more efficient therapy on myocardium repair (87). There was also a study that showed mitochondria transfer can also happen between MSCs and vascular smooth muscle cells to result in upregulating MSCs proliferation that led to a great promise in treating ischemic cardiomyopathy (88).
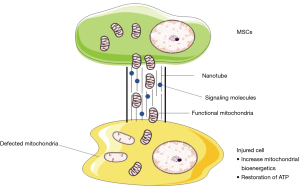
Despite the underlying mechanisms of MSCs remained to fully elucidated, the cardioprotective effect had been well confirmed by the established preclinical and clinical studies as summarized in Table 2.
Table 2
Studies | Findings | References |
---|---|---|
Preclinical studies | ||
Transplantation of magnetically labelled MSCs in a MI induced swine model | The studies demonstrated the restorative effect of transplanted magnetically labelled MSCs with the regeneration of new myocardium and prevented the remodeling in MI swine model under a 2-month follow-up | (89) |
Autologous MSCs showed reverse remodeling effect in swine model of chronic ischemic cardiomyopathy | BM-MSCs were safely delivered to the heart failure model. The size of myocardial infarct scar was reduced and thereby enhance the ventricular function by producing substantial structures and functional reverse remodeling | (90) |
Investigation of route of administration of mesenchymal cells in canine model with acute MI: IC and trans endocardial delivery | The safety profile of TE electromechanical-guided delivery of BM-MSCs was investigated by comparing the IC delivery with TE. This result demonstrated that acute MI’s canine model showed an increase of vascularity and cardiac function. There was a greater functional improvement in treating MI via TE delivery of MSCs | (91) |
Application of autologous mesenchymal stromal cells showed the improvement of myocardial function in porcine model via direct injection | The study showed the safety and efficacy of autologous MSCs in chronic ischemia-induced large animal models with no side effects or abnormal cell proliferation. In addition, it showed the improvement of cardiac function, formation of new vessels (angiogenesis) also detected | (92) |
Clinical studies | ||
A phase I study of human MSCs delivered by a novel device to treat heart attack patients | The phase I study primarily focused on the assessment of the safety and feasibility of MSCs delivery using a novel device with patient with recent MI. The study used autologous MSCs with delivery devices such as specialized catheter system to deliver MSCs to damaged site of cardiac tissue | (93) |
Effect of autologous MSCs on MI | The study is designed in the manner of randomized, double-blinded, placebo-controlled design. The status is completed. Patients with acute MI were assigned randomly to receive autologous MSCs or placebo via IC infusion which was directly delivered into coronary arteries. The results showed that there are some improvements in cardiac function and reduction in scar tissue, but overall results are not consistent | (94) |
Study of injection of ex vivo-expanded autologous BM-MSCs in acute patients via intramyocardial injection | The study was a completed phase I clinical studies. The study was done by injecting MSCs to patients with MI by primary PCI. It has been shown that the injection of MSCs in acute MI patients is associated with the enhancement of neovascularization and myogenesis. The study also presented the safety and feasibility of using intramyocardial injection for MSCs transplantation as route of administration in patients shortly after acute MI had shown the safety profile up to 5-year follow up | (95) |
Transplantation of MSCs to patients with ischemic cardiomyopathy via intramyocardial injection | This study was an early-phase clinical trial. A total of eight patients that suffer from LV dysfunction related to MI were injected intramyocardially with autologous BM-MSCs. The study showed that the patients have no observable side effects and improved regional contractility of a myocardial scar and reversing the remodeling effect | (96) |
The precise trial of adipose-derived regenerative cells in patients with ischemic cardiomyopathy | The clinical trial was conducted with the first randomized, placebo-controlled, double-blind trial to investigate the transplantation of adipose-derived regenerative cells via TE injection in patients with ischemic cardiomyopathy. The results suggested that the adipose-derived regenerative cells may have the ability to preserve ventricular function, myocardial perfusion in the patients. The injection in patients was safe and feasible | (97) |
MPCs served as adjunctive therapy in patients receiving the treatment of contemporary LVADs | This is a multicenter, double-blind, sham-procedure controlled preliminary trial that involved 30 patients. The study explores the strategy of injecting allogeneic MPCs along with the implantation of LVADs may lead to the myocardial recovery. The results showed that the administration of MPCs appeared to be safe with the potential signal of efficacy | (98) |
MSC, mesenchymal stem cell; MI, myocardial infarction; BM-MSCs, bone marrow-derived MSCs; IC, intracoronary; TE, transendocardial; PCI, percutaneous coronary intervention; LV, left ventricular; MPC, mesenchymal precursor cell; LVAD, left ventricular assist device.
Tissue engineering as a promising strategy for the application of MSCs
The tipping point of CVDs is due to the damage to the heart muscle regardless of acute or chronic that caused the heart failure progression (99). The heart cannot repair and replace its damaged cells by its native processes because of the low cell turnover rate which accounts for 0.3–1% annually (100) and lead to scar tissue development over the damaged myocardium region. The scar tissue caused the heart organ becomes intact but not contractible (99). However, based on the earlier research, most of the studies are focusing on the transplantation of organ in treating CVDs (1). The ideal intervention that could apply clinically could be either scar-forming prevention or replacement of formed scar tissue with functional cardiac muscle tissue by using MSCs due to its properties of tissue regeneration as mentioned in Table 1 (1,99). This approach had faced some of the limitations towards its success in medical use due to death of the MSCs upon injection, MSCs exit from heart or weak cellular integration with the receiving tissue (101-103). Thus, in the aspect of regenerative medicine for CVDs, many studies were performed for cardiovascular regeneration through tissue engineering (104). Up to now, cardiac tissue engineering is still not mature and ready for a routine clinical application but the autologous and allogeneic ASCs like MSCs have found to be successfully used in cardiac therapy in the clinical trials (105). Therefore, tissue engineering innovations hold a promising strategy to shape the research direction to result in a more reliable treatment (106).
The purpose of tissue engineering is to fulfill the cell requirement for their survival in any surroundings and create the suitable cellular microenvironment through the use of acceptable materials (99). It has brought the life science toward the development of biological substitutes for the purpose of restoring, maintaining and improving tissue function (107). The construct of tissue engineering of MSCs is underpinned by three significant components including relevant selection of the cells, a biomaterial scaffolds for three-dimensional (3D) culture and presence of the appropriate signals to coordinate the tissue regeneration via biophysical cues and signaling molecules like chemical mediators (108). Hence, in this review, the molecular and biomaterial engineering for cardiac tissue engineering, which cover the fundamentals and applications aspects will be discussed and introduced.
Use of genome-editing tools [clustered regularly interspaced short palindromic repeats/caspase 9 (CRISPR/Cas9) system]
Although MSCs possess various attractive properties that could be served as a regenerative medicine for CVDs as mentioned in Table 1, gene modification of MSCs can further improve its natural function in the aspects of tissue regeneration, organ-repairing and immunomodulation properties (109). Reprogramming the genome of MSCs could serve to augment the function of MSCs by upregulating or downregulating their desired genes to result in desired products production including pro- or anti-inflammatory chemokine or mediators in a controlled manner (110). In addition, foreign genes could also be introduced into the MSCs to modulate the therapeutic effects for specific applications by expressing non-native products (111). One of the limitations of the use of MSCs in treating CVDs is due to the low in vivo survival rate and integration rate after transplantation (25). In the physiological aspect, the use of therapeutic MSCs is to deliver to the site of injury under the condition of hypoxia which is sensitive to the MSCs due to the harsh local condition (112). Hence, by engineering the MSCs genetically could help to improve the survival as the survival rate of MSCs is especially essential in CVDs associated with damaged tissue under the condition of hypoxia including MI and stroke. The pro-survival strategy primarily targets the process of apoptosis by downregulating the elements that involved in apoptotic cascade or induction of pro-survival genes such as SDF-1β and hypoxia-inducible factor-1α (HIF-1α) (113,114). The beneficial effects of HIF-1α-engineered MSCs could be seen by the studies in the trials of mouse myocardial ischemia model (115).
Genetic modification or engineering of MSCs could be facilitated by the technology of CRISPR/Cas9. CRISPR is associated with a Cas9 nuclease complex along with a small guide RNA to produce a specific target break in the DNA sequence. Subsequently, a new sequence insertion could be done via homology-directed repair which served as correcting, inserting or silencing a specific gene (116). Hence, CRISPR/Cas9 was used for gene-editing of MSCs in order to result in desired therapeutic effects by enhancing its native gene or inserting a new gene (117).
Cell delivery and retention
There are a number of preclinical studies along with several clinical studies that evidenced the safety and feasibility of MSCs transplantation as the treatment of CVDs with various routes of delivery (118). Nevertheless, the low retention rate of MSCs is the common hurdle faced by all the existing routes of administration and delivery including systemic intravenous injection, intramyocardial injection, intracoronary injection as well as retrograde coronary venous injection (118,119). Cell retention is the fraction of MSCs retained in the damaged myocardium after transplantation in the time period ranging from minutes to days and played an important role in cardiac repair (120). As the advancement of tissue engineering application, the delivery of MSCs into the myocardium can be aided by using a suitable encapsulation biomaterials, microcapsule which consist of agarose, collagen, fibrin and dextran sulphate to provide long-term survival support (121). The microcapsule allows the mass transportation oxygen, growth factor and nutrients exchange optimally but the size is small enough to be delivered to the myocardium by offering a strong mechanical stability to protect the MSCs from constant tissue movement (121,122). According to Park et al., the study proposed that graphene oxide (GO) flakes could be used to serve as a protection of the MSCs from death mediated by reactive oxygen stress (ROS) and result in improving the therapeutic efficacy. The study also found that adherence of GO flakes to MSCs prior to the implantation facilitated the paracrine effects of MSCs which resulted in promoting restorative effect and cardiac tissue repair (123). Cell delivery vehicles such as fibrin glues (FG) would also enhance the attachment, growth and differentiation of MSCs and ultimately result in tissue formation within the injured tissues (124).
Biomaterial-based and biomimetic scaffold
As the possible attractive therapeutic effect of MSCs, cardiac tissue engineering is found to be able to facilitate the efficacy of MSCs by constructing bioartificial tissue that can be implanted into the patient body to provide support to the damaged heart tissue and restore function as shown in Figure 4 (125). A broad range of biomaterial strategies could be used for cardiac graft integration, remodeling, endogenous tissue regeneration by incorporating natural or synthetic polymer to mimic the tissue including cardiac patches, heart valves and vascular grafts (125,126). The biomaterials include chitosan, silk and alginate. The use of biodegradable polymeric scaffolds is always supplemented with biomolecules or protein to biomimics the cardiac extracellular matrix architecture as well as the biochemical composition to promote the integration and regeneration of the tissue. Besides the biocompatibility and biodegradability, the scaffold provided mechanical support to the regeneration with interconnected porous structures that allow nutrient and oxygen diffusion, cell migration and removal of waste (127).
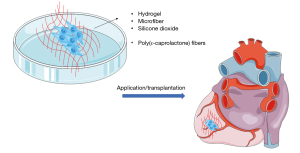
According to a study by Kim et al., the scaffolds containing silicon dioxide associated with nanoparticles as tissue engineering may facilitate the MSCs growth by activating the extracellular regulated kinase 1/2 (ERK1/2) (128). Recently, cardiac patch-based therapeutics had drawn great attention as a strategy for cardiac regeneration. A study showed that MSCs seeded with poly(ε-caprolactone) (PCL)/gelatin (PG) nanofibrous patches were found to have a strong and sufficient mechanical support effect to induce angiogenesis which resulted in speeding up the cardiac repair meanwhile reducing the fibrosis in an MI-induced rat model after 4 weeks of implantation (129). In addition, other study also demonstrated that radio-frequency plasma surface functionalized electrospun PCL fibers could serve as an appropriate matrix for BM-MSCs cardiac implantation in chronic MI rat model (130). The result showed that MSC-patch animal models only experienced 6% of decreased EF after 4-week implantation compared to the decrease of 13% of EF in sham-treated animal models (130).
3D bio-printing
Despite the rapid advancement of technology, there are still challenges existing in terms of developing the tissue construct that mimics their native microenvironment. Hence, 3D bioprinting had emerged as an enabling technology to construct engineered tissue to allow the efficient exchange of oxygen and nutrients (131). The technique of 3D printing refers to the use of materials that are combined into 3D shape through a computer-controlled process (132).
Angiogenesis or microcirculatory vessels are the main factors that directly influenced cardiac tissue engineering as the vessels are essentially needed for nutrient demand and gas exchange. Hence, 3D-bioprinting requires the mixture of biomaterials, angiogenic factors such as bioink and cells along with multidisciplinary approaches. The combination of stem cell engineering and tissue fabrication for autologous blood vessels synergistically recapitulate the biological functions and mechanical properties of native vessels (133). Thick vascularized tissue could be produced by bioprinting using 3D cell-laden ink. Cell-laden ink comprises thrombin, fibrinogen, gelatin, transglutaminase and cell (1). The study had demonstrated that the integration of parenchyma, endothelium, and stroma into a single thick tissue to bio-print simultaneously with multiple inks that composed of MSCs and human neonatal dermal fibroblasts (hNDFs) subsequently lined with human umbilical vein endothelial cells (HUVECs) (134). The study showed that the constructed thick vascularized tissue demonstrated an active perfusion with growth factors which required for the differentiation of MSCs (134). This approach that used cell-laden ink compared to the study done by Kolesky et al. which used multiple-cell laden, fugitive (vasculature) and ECM matrix ink to create vascularized tissue (thickness 1–2 mm) showed an improvement in physiological relevance with the thickness of more than 1 cm (135).
Challenges of using MSCs for cardiovascular regeneration
MSCs had offered the world a new hope in treating CVDs which had been proven by much of the research in terms of their feasibility, safety as well as efficacy. However, there are still some challenges that remain to be resolved (6). Some of the challenges discussed in this review article included the complexity and versatility of the sources of MSCs, low survival and retention rate of MSCs, route of administration as well as ethical and safety issues of MSCs.
Complexity and versatility of the source of MSCs
The variabilities in cell sources presented the problem of the use of MSCs has yet to be resolved (136). Different sources of MSCs showed different ability of differentiation and discrete immunoregulation effects in CVDs. According to the study, umbilical cord lining-derived MSCs (CL-MSCs), and adult BM-MSCs showed difference in the immunogenic profile and the capacity to respond to inflammatory stimuli as well as the suppression of allogeneic immune activation. CL-MSCs demonstrated a more beneficial immunogenic profile as well as immunosuppressive potential compared to BM-MSCs (137). Other comparative study also showed that CL-MSCs demonstrated a high proliferation and migration rates to prolong the survival of immunodeficient mice. It has the best characteristics to use as stem cell-based therapy due to its hypo-immunogenic effect (138). In addition, there is no consensus in culturing MSCs which resulted in different therapeutic effects in CVDs (6). The culture condition would also affect the MSCs immunomodulatory (139). Hagmann et al. investigated that the choice of expansion medium showed an impact on the potential of differentiation ability, growth and expression of surface markers of MSCs (140). Culture conditioned with growth factors such as FGF-2 showed an increase in the proliferation of MSCs (141).
Low retention and survival rate of MSCs and in vitro expansion efficacy
The successfulness of transplantation of the MSCs in the graft site as well as the ability of MSCs to survive contribute to the exertion of MSCs’ therapeutic effects to treat CVDs. However, there are researches reported the retention rate of stem cell is low as aforementioned and the hostile ischemic environment would affect the immunogenicity of transplanted MSCs (121). Even though there is a lot of literature supporting that the use of MSCs in animal studies that have shown cardiac function improvement and cardiac recovery, the problems and challenges arose when translated into clinical application (142,143). Some of the clinical studies also reported some convincing data that there is no significant improvement following the MSCs treatment. This might be contributed by the weak retention and survival rate of MSCs after implantation (121). It has been known that there will be a massive cell loss upon the implantation due to wash out of the small cells during microcirculation into the punctured microvascular network as a result of tissue’s contractile nature (102). The continuous movement of the tissues in our body would also impair the cell attachment and integration to the host tissue which eventually lead to the progressively cell death (144). Hence, microencapsulation of the MSCs with alginate-poly-L-lysine-alginate (APA) or other biomaterial such as neuregulin-1 could improve the survival and retention rate of the MSCs in therapeutic use (145,146).
In vitro expansion efficacy played a role in using MSCs as therapeutic agent for CVDs to obtain enough cells to serve as cellular therapy. However, spontaneous senescence of the MSCs limited the death of cells and had become one of the problems in the expansion efficacy (6). To date, the understanding of the cellular senescence of MSCs is still lacking due to the fact that senescence of MSCs is so heterogeneous with different phenotypic markers. Hence, for the use of MSCs in therapy, a well-established method to generate a large population of the MSCs is needed (147). Studies showed that by introducing of human telomerase reverse transcriptase (hTERT) gene into MSCs would result in substantial multiplication of the lifespan of MSCs meanwhile preserving the normal karyotype and elongation of telomere without affecting the ability of MSCs to differentiate (148).
Route of administration
The availability of the route of administration of MSCs including intravenous, intracoronary, intramyocardial (149). Direct intramyocardial injection has been commonly used as a route of cell administration for cellular cardiomyopathy. However, the study demonstrated that this route could cause rapid loss of the implanted cell from the contracting of the myocardium as well as the washout (102). Intravenous administration of MSCs was known to be the least invasive method among the others. However, the feasibility of this method relies on the homing signals of MSCs to the infarcted area after acute MI (149). Intravenous method is not preferable for treating CVDs as the preclinical studies had reported the low cell retention of MSCs within the heart after the implantation and the lungs would trap most of the injected cells. On the other hand, direct left ventricular cavity infusion could facilitate the migration and colonization of the MSCs to the ischemic myocardium. Intracoronary administration is a preferred technique that has been used extensively due to it has the potential to distribute the cells homogeneously to the targeted myocardium (150). However, this method is unsuitable when there is an occlusion of inflow arteries as the occlusion would decrease the blood flow that caused ischemia leading to arrhythmia (91,151). Hence, more extensive research is needed to optimize the best approach of administration of candidate MSCs to ensure the therapeutic effects of MSCs.
Ethical and safety issue of MSCs
MSCs are not really bound to ethical issues as compared to ESCs as the isolation does not involve any sacrifice of any life form (152,153). Nevertheless, the ethical issues will still arise if it involves the genetic modification of MSCs and causes a greater challenge towards the clinical setting (154). Unmodified MSCs confronted with the issue of standardization deficiency that caused discrepancy of therapeutic effects which lead to the modification of MSCs. Nevertheless, careful monitoring of random genomic integration in hosts is still needed as random integration would result in critical genomic dysfunction and potential to mutate the gene that caused malignancies (155). Therefore, this would affect the social acceptance towards the genetically modified MSCs.
Additionally, the safety issue of MSCs is yet to be elucidated completely. Despite a lot of the clinical applications had demonstrated that the MSCs shown the benefit in therapy for CVDs, but the ability of the MSCs to promote the tumor growth and metastasis due to their potential to suppress anti-tumor response and angiogenesis raised the concerns in the aspect of regenerative medicine (156). MSCs that undergo unwanted differentiation may also intensify the malignant disease by bridging the gap between neo-angiogenesis and anti-tumor response to modulate the tumor growth (156,157). There are studies showing that MSCs could increase tumor growth. According to a study, the findings suggested that MSCs could favor the growth of tumor in vivo when the mice is injected together with the tumor cell and MSCs (158). The immunomodulatory characteristics of MSCs cause the MSCs to migrate to the primary tumor and promote tumor growth (156). According to the study done by Ljujic et al., human MSCs could home themselves to the tumor site and promote tumor growth in metastatic breast cancer mice. It is due to human MSCs being able to stimulate the production of T helper (Th)2 cytokine which resulted in developing metastatic tumor by increasing CD4+Foxp3+ regulatory T (Treg) cells (159). Study also suggested that co-culturing of T cells with MSCs could result in the decrease production of cytokine including IFN-γ, IL-1α, and tumor necrosis factor-alpha (TNF-α) but increase Th2 cytokines such as IL-10, IL-5, and IL-13. This had confirmed the fact the MSCs could promote tumor growth by creating an immunosuppressive microenvironment (160).
In addition, recent reports questioned the transdifferentiation of MSCs after injected into myocardium rather than its proposed therapeutic benefits (161). MSCs have the potential of unwanted differentiation into undesired tissues including cartilage and bone. The study reported that encapsulated structures were observed in the infarcted area of the damaged heart in mice models that were injected with MSCs associated with calcification or ossifications (162). However, some of the studies also demonstrated that there is no evidence for arrhythmogenic and tumorigenic risk after extensive preclinical and clinical studies. Hence, more research should be carried out to validate the safety of MSCs (163).
Possible solutions to overcome the challenges
It has been known that the outcome of MSCs therapeutic intervention is strongly relied on the delivery of MSCs to the desired site. However, the current available routes of administration of the cell products including the most preferred method, intramyocardial injection, gave a very inefficient results due to poor engraftment of the cells (164,165). Thus, the use of MSCs as a promising treatment must be accompanied by developing suitable approaches to locate the cell products to desired site and survive after transplantation. In order to address the issues, the area of nanomedicine could be applied to maximize the efficacy of MSCs treatment by directing the cell products to injured area. A promising study by Cheng et al. used iron nanoparticles conjugated with two types of antibodies which further developed into magnetic bifunctional cell engager (MagBICE) to accurately direct the cell products to the injured site to treat acute MI mouse model. The technology applied was highly generalizable for regenerative medicine which include molecular targeting and non-invasive imaging all in just one small particles (166). Another animal studies by Chang et al. demonstrated the use of ultrasound-mediated microbubble destruction combined with intracoronary transplantation showed an increase in the homing efficiency of BM-MSCs with the promising outcome of having more BM-MSCs to differentiate into cardiomyocytes and thereby improved the cardiac function in (71). Both studies mentioned above required further extensive investigation to proceed the preclinical studies to clinical studies.
Another major challenge for the survival of MSCs transplantation is the harsh microenvironment of the damaged host tissue such as oxidative stress and scarcity of nutrients that lead to the cell death (167). The success outcome of transplanted MSCs primarily depends on the hypoxia-induced proapoptotic signaling mediated by Toll-like receptor (TLR4) and pro-survival pathways such as Akt (168,169). Hence, these signaling pathways and molecules can be used as targets for genetic modification of MSCs to increase its survival and proliferation rate. A recent study by Cai et al. has designed a novel delivery system for MSCs transplantation self-assembling peptide (SAP) modified with pro-survival peptide, glutamine-histidine-arginine-glutamic acid-aspartic acid-glycine-serine (QHREDGS). The study was tested on the MSCs cultured with the modified SAP (SAP-MSCs) and was found out that the modified SAP increased the proliferation of MSCs and markedly decreased apoptosis when exposed to oxygen and glucose deprivation meanwhile increase the level of phosphorylated Akt. The results were very promising in rat models that injected with SAP-MSCs which significantly improved cardiac function and reduced infarct size (170).
Genetic modification of MSCs is also one of the approaches to improve the survival of MSCs. Study by Brewster et al. reported a promising result by knocking out TLR4 gene in MSCs. The study showed that the survival of TLR4-knockout MSCs was greatly improved after hypoxic injury due to the Akt pathway signal was increased and thereby resulted in cardioprotection (171). Research has shown that the miRNA, miRNA-133a, which abundantly expressed in cardiac muscle, is down regulated in the patients that suffered with MI (172). Hence, miRNA-133a could be the novel therapeutic target as research also found that it plays a role in attenuating fibrosis, cardiac remodeling and preventing cardiac hypertrophy (173,174). Study by Dakhlallah et al. reprogrammed MSCs using double-stranded oligonucleuotides, miRNA-mimics via transfection. miRNA-133a transfected MSCs were transplanted into rat models subjected to MI was found to markedly improve cell engraftment and reduce fibrosis. In terms of molecular level, the quantitative reverse transcriptase polymerase chain reaction (qRT-PCR) data had shown the reduction in the proapoptotic gene, Apaf-1, Cas3, and Cas9 which significantly enhance the survival of transplanted MSCs (175). Hence, genetically modified MSCs with certain miRNAs may elicit multiple promising therapeutic effects and it is worthwhile to study extensively to enhance the efficacy of MSCs transplantation.
Numerous studies have also explored the in vitro exposure of MSCs to pharmacological agents, cytokines, and growth factors. A pharmacological agent, trimetazidine, has been extensively studied. An in vitro study by Gong et al. found that MSCs cultured with trimetazidine had shown a reduction in apoptosis via Akt pathway activation (176). Another preclinical study had also proven the therapeutic effect of MSCs cultured with trimetazidine found to improve the cardiac function and reduce infarct size when treated in rat model via intramyocardial administration (177). Other pharmacological agents such as nicorandil, melatonin and angiotensin II are also found to increase the survival of MSCs and thereby improve the efficacy of stem cell treatment (178-180). Growth factors such as IGF-1 is one of the essential paracrine factors for MSCs and contributing to the role of MSCs to exert its therapeutic effects (181). IGF-1 is also known to suppress the expression of inflammation cytokines TNF-α, IL-1β, and IL-6 (182). A preclinical study by Guo et al. reported the transplantation of MSCs that pretreated with IGF-1 found to decrease the production of inflammatory cytokines as mentioned above in peri-MI area and increased the survival rate of engrafted cells in ischemic heart (183). Hence, preconditioning of MSCs with growth factors or cytokine is another solution that is worthwhile to explore to make cell therapy feasible in the future.
Future directions
To date, there are large number of studies from preclinical and early clinical phase trials that elucidate the potential therapeutic effects of MSCs therapy in CVDs. However, given that the low retention and survival rate of MSCs had greatly affected the long-term efficacy of the treatment. Genetic modification via bioengineering and pretreatment of the MSCs might be the future of MSCs therapy that hold a greater promising result to address the lifespan of MSCs in host body and contribute to the result of permanent regenerative medicine. The research paradigm has shifted toward to enhance the MSCs therapy via pretreatment with various types of factors and genetic engineering despite there is still lack of clinical translation to date. Hence, more extensive research is needed to bring forward the study into a clinical translation setting in the near future. As for genetic modification of MSCs, the choice of vectors used, and the technique of transfection must be studied extensively to minimize the destruction of MSCs. Furthermore, the data also showed that there are only approximately 1–10% stem cells injected either in myocardium or coronary arteries that remain in the heart after 2 hours due to the movement of MSCs through myocardial veins and lymphatic vessels (184). Thus, research in the near future should not just focus on the survival of MSCs but meanwhile techniques such as nanoparticles and cardiac patches should also be applied to facilitate the cell engraftment in the site of damaged heart (185). A more sophisticated and sensitive imaging techniques such as computed tomography (CT) and magnetic resonance imaging (MRI) could be employed to perform analysis on the clinical efficacy of MSCs therapy in CVD patients to measure the changes in terms of the size of infarcted area and left ventricular EF (LVEF) (154). Additionally, both imaging techniques are essentially needed to detect the target area for MSCs therapy and thereby potentially to serve as a reference to design a more appropriate cell delivery method. The versality of MSCs needs to be addressed by standardizing and optimizing the cell selection based on easy harvesting and ready to be expanded ex vivo in large scale. In-quality control such as selection of donors, handling medicine, use of cell banks and cryopreservation are needed to ensure a homogeneous manufacturing of MSCs and the safety and efficacy of the use of cell products (186). The effect of different sources of MSCs, autologous or allogenic, must be investigated to make sure the source of MSCs does not affect the beneficial effects of MSCs therapy in ischemic cardiomyopathy patients. As of now, there are only two early phase clinical trials, POSEIDON (163) and POSEIDON DCM (187), were found to compare the effectiveness of autologous and allogenic sources of MSCs in ischemic heart failure and dilated cardiomyopathy. More supporting data and third phase clinical results are needed to fully elucidate the source of MSCs. Lastly, more clinical data and long-term research are needed to evident the safety of MSCs, addressing the appropriate dosage of cell products to elicit the maximum therapeutic effects in patients with the least adverse effect. The future of MSCs treatment is summarized in Table 3 in the manner of SWOT analysis (strengths, weaknesses, opportunities, and threats).
Table 3
Strengths | Weaknesses | Opportunities | Threats |
---|---|---|---|
(I) Regenerative potential: the multipotency nature of MSCs which gives the ability to differentiate into variety range of cell lineage including cardiac cell (188) | (I) Limited differentiation: MSCs lose their differentiation potential and exhibit changes in terms of their morphology if culture in long-term (189) | (I) AI in MSCs treatment: use of AI to aid in the understanding of molecular characteristics of MSCs such as protein sequences, molecular structure of the proteins, binding stability between cell receptors and ligands (190) | (I) Regulatory hurdles: the regulatory path is the key factor that affects the speed of translation MSCs treatment into clinical settings. Successful translation requires a comprehensive understanding of the hurdles at the early stages of development such as cell culture, manipulation, processed cells or cells used in a heterologous manner (191) |
(II) Minimal ethical concern: lack of ethical issues and have great advantages over ESCs (192) | (II) Engraftment and survival: minimal long-term engraftment which persists for limited time due to immune clearance from host (193) | (II) Personalized medicine: by targeting and genetically engineered cellular therapy particular specific to disease state of the individuals which applied to different pathologies (194) | (II) Clinical efficacy and long-term safety: lack of efficacy data for long-term follow up. MSCs may promote metastasis through angiogenesis due to their differentiation capacity into endothelial cells (156) |
(III) Relatively non-invasive: the application of MSCs as therapeutic treatment with minimal invasive procedure such as intradermal injection (192) | (III) Combined therapies: the therapeutic effect of MSCs could be further enhanced by bioactive molecules such as tamoxifen or chloroquine to achieve better immunoregulatory properties (195) |
SWOT, strengths, weaknesses, opportunities, and threats; MSC, mesenchymal stem cell; AI, artificial intelligence; ESC, embryonic stem cell.
Conclusions and prospects
MSCs therapy is an exciting, emerging and dynamic area in regenerative medicine with full potential in recovering CVDs which is the primary factor of death worldwide. MSCs have gained a great attention and consideration due to its advantages of wide range of sources, can be isolated easily as well as variety properties including cardiomyogenesis, immunomodulatory and anti-inflammatory, anti-fibrosis and neovascularization. Furthermore, there are also promising results from the preclinical and clinical studies reported MSCs could improve the cardiac function and recovery, reverse the remodeling effect which contributed by the migration of MSCs to infarcted area, lowering the response of inflammation, differentiate into cardiomyocytes, reduce fibrosis and new blood vessels formation.
Even though there is a large number of supporting data from preclinical and clinical studies showing the effectiveness, therapeutic benefits and safety of the use of MSCs, the exact mechanism of action of the application of MSCs in treating CVDs is yet to be fully elucidated. More research on studying the mechanism of action is needed. However, with the advancement of science and technology, MSCs-based tissue engineering had been further developed to develop the therapeutic effects, survival as well homing efficiency of MSCs by modulating the microstructure of biomaterials to allow MSCs to be applied in medicine practically.
Tissue engineering technologies such as tissue scaffold and 3D bioprinting allowed the researcher to engineer the heart tissue which showed encouraging findings, however the translation into clinical setting is still required. Despite the advantages, there are still limitations and challenges faced using MSCs in terms of identification of MSCs due to its complexity and versatility, the low survival and retention rate of the MSCs, the effectiveness in the route of administration as well as the ethical and safety issues of MSCs.
Considering that MSCs had been frequently used as human remedy but the concern of MSCs may promote tumor growth and metastasis, more studies should focus on long-term monitoring of MSCs-treated animals to determine other detrimental effects of long-term use of MSCs. Besides that, the optimization of the route of administration approach is needed to result in the best therapeutic effects of MSCs and cause minimal invasiveness to the patients. Furthermore, improving the understanding of the biology and the properties of different types of MSCs derived from different sources could help in refining the capacity of MSCs to promote cardiac repair. Further research could be done on the investigation of the synergy effects of combining therapeutic MSCs with other cell types like macrophages or CSCs to improve the therapeutic effects (196,197). Although there are limitations and challenges in using MSCs, it is still a future promising therapy in treating CVDs with continuously optimizing the approaches to make it feasible.
Acknowledgments
Funding: None.
Footnote
Peer Review File: Available at https://atm.amegroups.com/article/view/10.21037/atm-23-1936/prf
Conflicts of Interest: Both authors have completed the ICMJE uniform disclosure form (available at https://atm.amegroups.com/article/view/10.21037/atm-23-1936/coif). The authors have no conflicts of interest to declare.
Ethical Statement: The authors are accountable for all aspects of the work in ensuring that questions related to the accuracy or integrity of any part of the work are appropriately investigated and resolved.
Open Access Statement: This is an Open Access article distributed in accordance with the Creative Commons Attribution-NonCommercial-NoDerivs 4.0 International License (CC BY-NC-ND 4.0), which permits the non-commercial replication and distribution of the article with the strict proviso that no changes or edits are made and the original work is properly cited (including links to both the formal publication through the relevant DOI and the license). See: https://creativecommons.org/licenses/by-nc-nd/4.0/.
References
- Ji ST, Kim H, Yun J, et al. Promising Therapeutic Strategies for Mesenchymal Stem Cell-Based Cardiovascular Regeneration: From Cell Priming to Tissue Engineering. Stem Cells Int 2017;2017:3945403. [Crossref] [PubMed]
- Roth GA, Mensah GA, Johnson CO, et al. Global Burden of Cardiovascular Diseases and Risk Factors, 1990-2019: Update From the GBD 2019 Study. J Am Coll Cardiol 2020;76:2982-3021. [Crossref] [PubMed]
- Virani SS, Alonso A, Aparicio HJ, et al. Heart Disease and Stroke Statistics-2021 Update: A Report From the American Heart Association. Circulation 2021;143:e254-e743. [Crossref] [PubMed]
- Ruan Y, Guo Y, Zheng Y, et al. Cardiovascular disease (CVD) and associated risk factors among older adults in six low-and middle-income countries: results from SAGE Wave 1. BMC Public Health 2018;18:778. [Crossref] [PubMed]
- Raziyeva K, Smagulova A, Kim Y, et al. Preconditioned and Genetically Modified Stem Cells for Myocardial Infarction Treatment. Int J Mol Sci 2020;21:7301. [Crossref] [PubMed]
- Guo Y, Yu Y, Hu S, et al. The therapeutic potential of mesenchymal stem cells for cardiovascular diseases. Cell Death Dis 2020;11:349. [Crossref] [PubMed]
- Stewart J, Manmathan G, Wilkinson P. Primary prevention of cardiovascular disease: A review of contemporary guidance and literature. JRSM Cardiovasc Dis 2017;6:2048004016687211. [Crossref] [PubMed]
- Flora GD, Nayak MK. A Brief Review of Cardiovascular Diseases, Associated Risk Factors and Current Treatment Regimes. Curr Pharm Des 2019;25:4063-84. [Crossref] [PubMed]
- Heitor Reis A. On the etiology of cardiovascular diseases: A new framework for understanding literature results. Med Hypotheses 2016;92:94-9. [Crossref] [PubMed]
- Huffman MD, Bhatnagar D. Novel treatments for cardiovascular disease prevention. Cardiovasc Ther 2012;30:257-63. [Crossref] [PubMed]
- Chang D, Fan T, Gao S, et al. Application of mesenchymal stem cell sheet to treatment of ischemic heart disease. Stem Cell Res Ther 2021;12:384. [Crossref] [PubMed]
- Meads C, Cummins C, Jolly K, et al. Coronary artery stents in the treatment of ischaemic heart disease: a rapid and systematic review. Health Technol Assess 2000;4:1-153. [Crossref] [PubMed]
- Golpanian S, Wolf A, Hatzistergos KE, et al. Rebuilding the Damaged Heart: Mesenchymal Stem Cells, Cell-Based Therapy, and Engineered Heart Tissue. Physiol Rev 2016;96:1127-68. [Crossref] [PubMed]
- Gabriel-Costa D. The pathophysiology of myocardial infarction-induced heart failure. Pathophysiology 2018;25:277-84. [Crossref] [PubMed]
- Karantalis V, Balkan W, Schulman IH, et al. Cell-based therapy for prevention and reversal of myocardial remodeling. Am J Physiol Heart Circ Physiol 2012;303:H256-70. [Crossref] [PubMed]
- Marelli D, Desrosiers C, el-Alfy M, et al. Cell transplantation for myocardial repair: an experimental approach. Cell Transplant 1992;1:383-90. [Crossref] [PubMed]
- Hare JM, Traverse JH, Henry TD, et al. A randomized, double-blind, placebo-controlled, dose-escalation study of intravenous adult human mesenchymal stem cells (prochymal) after acute myocardial infarction. J Am Coll Cardiol 2009;54:2277-86. [Crossref] [PubMed]
- Wei X, Yang X, Han ZP, et al. Mesenchymal stem cells: a new trend for cell therapy. Acta Pharmacol Sin 2013;34:747-54. [Crossref] [PubMed]
- Harvey A, Caretti G, Moresi V, et al. Interplay between Metabolites and the Epigenome in Regulating Embryonic and Adult Stem Cell Potency and Maintenance. Stem Cell Reports 2019;13:573-89. [Crossref] [PubMed]
- Friedenstein AJ, Chailakhjan RK, Lalykina KS. The development of fibroblast colonies in monolayer cultures of guinea-pig bone marrow and spleen cells. Cell Tissue Kinet 1970;3:393-403. [Crossref] [PubMed]
- Nombela-Arrieta C, Ritz J, Silberstein LE. The elusive nature and function of mesenchymal stem cells. Nat Rev Mol Cell Biol 2011;12:126-31. [Crossref] [PubMed]
- Colicchia M, Jones DA, Beirne AM, et al. Umbilical cord-derived mesenchymal stromal cells in cardiovascular disease: review of preclinical and clinical data. Cytotherapy 2019;21:1007-18. [Crossref] [PubMed]
- Williams AR, Hatzistergos KE, Addicott B, et al. Enhanced effect of combining human cardiac stem cells and bone marrow mesenchymal stem cells to reduce infarct size and to restore cardiac function after myocardial infarction. Circulation 2013;127:213-23. [Crossref] [PubMed]
- Karp JM, Leng Teo GS. Mesenchymal stem cell homing: the devil is in the details. Cell Stem Cell 2009;4:206-16. [Crossref] [PubMed]
- Li L, Chen X, Wang WE, et al. How to Improve the Survival of Transplanted Mesenchymal Stem Cell in Ischemic Heart? Stem Cells Int 2016;2016:9682757. [Crossref] [PubMed]
- Hass R, Kasper C, Böhm S, et al. Different populations and sources of human mesenchymal stem cells (MSC): A comparison of adult and neonatal tissue-derived MSC. Cell Commun Signal 2011;9:12. [Crossref] [PubMed]
- Farini A, Sitzia C, Erratico S, et al. Clinical applications of mesenchymal stem cells in chronic diseases. Stem Cells Int 2014;2014:306573. [Crossref] [PubMed]
- Jang HR, Park JH, Kwon GY, et al. Effect of preemptive treatment with human umbilical cord blood-derived mesenchymal stem cells on the development of renal ischemia-reperfusion injury in mice. Am J Physiol Renal Physiol 2014;307:F1149-61. [Crossref] [PubMed]
- Secco M, Moreira YB, Zucconi E, et al. Gene expression profile of mesenchymal stem cells from paired umbilical cord units: cord is different from blood. Stem Cell Rev Rep 2009;5:387-401. [Crossref] [PubMed]
- Alsalameh S, Amin R, Gemba T, et al. Identification of mesenchymal progenitor cells in normal and osteoarthritic human articular cartilage. Arthritis Rheum 2004;50:1522-32. [Crossref] [PubMed]
- Zvaifler NJ, Marinova-Mutafchieva L, Adams G, et al. Mesenchymal precursor cells in the blood of normal individuals. Arthritis Res 2000;2:477-88. [Crossref] [PubMed]
- Li Z, Hu X, Zhong JF. Mesenchymal Stem Cells: Characteristics, Function, and Application. Stem Cells Int 2019;2019:8106818. [Crossref] [PubMed]
- Toma C, Pittenger MF, Cahill KS, et al. Human mesenchymal stem cells differentiate to a cardiomyocyte phenotype in the adult murine heart. Circulation 2002;105:93-8. [Crossref] [PubMed]
- Via AG, Frizziero A, Oliva F. Biological properties of mesenchymal Stem Cells from different sources. Muscles Ligaments Tendons J 2012;2:154-62. [PubMed]
- Dominici M, Le Blanc K, Mueller I, et al. Minimal criteria for defining multipotent mesenchymal stromal cells. The International Society for Cellular Therapy position statement. Cytotherapy 2006;8:315-7. [Crossref] [PubMed]
- Horwitz EM, Le Blanc K, Dominici M, et al. Clarification of the nomenclature for MSC: The International Society for Cellular Therapy position statement. Cytotherapy 2005;7:393-5. [Crossref] [PubMed]
- Jones EA, Kinsey SE, English A, et al. Isolation and characterization of bone marrow multipotential mesenchymal progenitor cells. Arthritis Rheum 2002;46:3349-60. [Crossref] [PubMed]
- Squillaro T, Peluso G, Galderisi U. Clinical Trials With Mesenchymal Stem Cells: An Update. Cell Transplant 2016;25:829-48. [Crossref] [PubMed]
- Ullah M, Liu DD, Thakor AS. Mesenchymal Stromal Cell Homing: Mechanisms and Strategies for Improvement. iScience 2019;15:421-38. [Crossref] [PubMed]
- Liesveld JL, Sharma N, Aljitawi OS. Stem cell homing: From physiology to therapeutics. Stem Cells 2020;38:1241-53. [Crossref] [PubMed]
- Chong JJ, Chandrakanthan V, Xaymardan M, et al. Adult cardiac-resident MSC-like stem cells with a proepicardial origin. Cell Stem Cell 2011;9:527-40. [Crossref] [PubMed]
- Shen Z, Huang W, Liu J, et al. Effects of Mesenchymal Stem Cell-Derived Exosomes on Autoimmune Diseases. Front Immunol 2021;12:749192. [Crossref] [PubMed]
- Szaraz P, Gratch YS, Iqbal F, et al. In Vitro Differentiation of Human Mesenchymal Stem Cells into Functional Cardiomyocyte-like Cells. J Vis Exp 2017;55757. [PubMed]
- Wang LD, Wagers AJ. Dynamic niches in the origination and differentiation of haematopoietic stem cells. Nat Rev Mol Cell Biol 2011;12:643-55. [Crossref] [PubMed]
- Miteva K, Pappritz K, El-Shafeey M, et al. Mesenchymal Stromal Cells Modulate Monocytes Trafficking in Coxsackievirus B3-Induced Myocarditis. Stem Cells Transl Med 2017;6:1249-61. [Crossref] [PubMed]
- Najar M, Raicevic G, Fayyad-Kazan H, et al. Mesenchymal stromal cells and immunomodulation: A gathering of regulatory immune cells. Cytotherapy 2016;18:160-71. [Crossref] [PubMed]
- Ringdén O, Uzunel M, Rasmusson I, et al. Mesenchymal stem cells for treatment of therapy-resistant graft-versus-host disease. Transplantation 2006;81:1390-7. [Crossref] [PubMed]
- Fan D, Takawale A, Lee J, et al. Cardiac fibroblasts, fibrosis and extracellular matrix remodeling in heart disease. Fibrogenesis Tissue Repair 2012;5:15. [Crossref] [PubMed]
- Gubert F, da Silva JS, Vasques JF, et al. Mesenchymal Stem Cells Therapies on Fibrotic Heart Diseases. Int J Mol Sci 2021;22:7447. [Crossref] [PubMed]
- Mazo M, Araña M, Pelacho B, et al. Mesenchymal stem cells and cardiovascular disease: a bench to bedside roadmap. Stem Cells Int 2012;2012:175979. [Crossref] [PubMed]
- Danieli P, Malpasso G, Ciuffreda MC, et al. Conditioned medium from human amniotic mesenchymal stromal cells limits infarct size and enhances angiogenesis. Stem Cells Transl Med 2015;4:448-58. [Crossref] [PubMed]
- Shi W, Xin Q, Yuan R, et al. Neovascularization: The Main Mechanism of MSCs in Ischemic Heart Disease Therapy. Front Cardiovasc Med 2021;8:633300. [Crossref] [PubMed]
- Uemura R, Xu M, Ahmad N, et al. Bone marrow stem cells prevent left ventricular remodeling of ischemic heart through paracrine signaling. Circ Res 2006;98:1414-21. [Crossref] [PubMed]
- Bacaner M, Brietenbucher J, LaBree J. Prevention of ventricular fibrillation, acute myocardial infarction (myocardial necrosis), heart failure, and mortality by bretylium: is ischemic heart disease primarily adrenergic cardiovascular disease? Am J Ther 2004;11:366-411. [Crossref] [PubMed]
- Sekulic M, Zacharias M, Medalion B. Ischemic Cardiomyopathy and Heart Failure. Circ Heart Fail 2019;12:e006006. [Crossref] [PubMed]
- Malakar AK, Choudhury D, Halder B, et al. A review on coronary artery disease, its risk factors, and therapeutics. J Cell Physiol 2019;234:16812-23. [Crossref] [PubMed]
- Mykhaylichenko VY, Kostyamin YD, Samarin SA. Options for using cellular cardiomyoplasty for coronary heart disease. Journal of Clinical Practice 2020;11:13-22. [Crossref]
- Okpala A, Joykutty L. Mesenchymal Stromal Cells Used for Cellular Cardiomyoplasty As a Way To Treat Myocardial Infarction and Heart Failure. Journal of Student Research 2021. doi:
10.47611/jsrhs.v10i3.1917 .10.47611/jsrhs.v10i3.1917 - Nursalim A, Katili PA, Santoso T. Cellular cardiomyoplasty for myocardial infarction: a 2014 evidence-based update. Acta Med Indones 2014;46:150-62. [PubMed]
- Wakitani S, Saito T, Caplan AI. Myogenic cells derived from rat bone marrow mesenchymal stem cells exposed to 5-azacytidine. Muscle Nerve 1995;18:1417-26. [Crossref] [PubMed]
- Ratajczak MZ, Kucia M, Jadczyk T, et al. Pivotal role of paracrine effects in stem cell therapies in regenerative medicine: can we translate stem cell-secreted paracrine factors and microvesicles into better therapeutic strategies? Leukemia 2012;26:1166-73. [Crossref] [PubMed]
- Nagaya N, Fujii T, Iwase T, et al. Intravenous administration of mesenchymal stem cells improves cardiac function in rats with acute myocardial infarction through angiogenesis and myogenesis. Am J Physiol Heart Circ Physiol 2004;287:H2670-6. [Crossref] [PubMed]
- Hatzistergos KE, Quevedo H, Oskouei BN, et al. Bone marrow mesenchymal stem cells stimulate cardiac stem cell proliferation and differentiation. Circ Res 2010;107:913-22. [Crossref] [PubMed]
- Xie XJ, Wang JA, Cao J, et al. Differentiation of bone marrow mesenchymal stem cells induced by myocardial medium under hypoxic conditions. Acta Pharmacol Sin 2006;27:1153-8. [Crossref] [PubMed]
- Wang JS, Shum-Tim D, Galipeau J, et al. Marrow stromal cells for cellular cardiomyoplasty: feasibility and potential clinical advantages. J Thorac Cardiovasc Surg 2000;120:999-1005. [Crossref] [PubMed]
- Reinecke H, Minami E, Zhu WZ, et al. Cardiogenic differentiation and transdifferentiation of progenitor cells. Circ Res 2008;103:1058-71. [Crossref] [PubMed]
- Ho JH, Ma WH, Su Y, et al. Thymosin beta-4 directs cell fate determination of human mesenchymal stem cells through biophysical effects. J Orthop Res 2010;28:131-8. [Crossref] [PubMed]
- Khoury M, Drake A, Chen Q, et al. Mesenchymal stem cells secreting angiopoietin-like-5 support efficient expansion of human hematopoietic stem cells without compromising their repopulating potential. Stem Cells Dev 2011;20:1371-81. [Crossref] [PubMed]
- Gnecchi M, He H, Noiseux N, et al. Evidence supporting paracrine hypothesis for Akt-modified mesenchymal stem cell-mediated cardiac protection and functional improvement. FASEB J 2006;20:661-9. [Crossref] [PubMed]
- Ranganath SH, Levy O, Inamdar MS, et al. Harnessing the mesenchymal stem cell secretome for the treatment of cardiovascular disease. Cell Stem Cell 2012;10:244-58. [Crossref] [PubMed]
- Chang X, Liu J, Liao X, et al. Ultrasound-mediated microbubble destruction enhances the therapeutic effect of intracoronary transplantation of bone marrow stem cells on myocardial infarction. Int J Clin Exp Pathol 2015;8:2221-34. [PubMed]
- Liu CP, Yeh JL, Wu BN, et al. KMUP-3 attenuates ventricular remodelling after myocardial infarction through eNOS enhancement and restoration of MMP-9/TIMP-1 balance. Br J Pharmacol 2011;162:126-35. [Crossref] [PubMed]
- Sarkar D, Ankrum JA, Teo GS, et al. Cellular and extracellular programming of cell fate through engineered intracrine-, paracrine-, and endocrine-like mechanisms. Biomaterials 2011;32:3053-61. [Crossref] [PubMed]
- Huang L, Ma W, Ma Y, et al. Exosomes in mesenchymal stem cells, a new therapeutic strategy for cardiovascular diseases? Int J Biol Sci 2015;11:238-45. [Crossref] [PubMed]
- Lai RC, Arslan F, Lee MM, et al. Exosome secreted by MSC reduces myocardial ischemia/reperfusion injury. Stem Cell Res 2010;4:214-22. [Crossref] [PubMed]
- Lai RC, Chen TS, Lim SK. Mesenchymal stem cell exosome: a novel stem cell-based therapy for cardiovascular disease. Regen Med 2011;6:481-92. [Crossref] [PubMed]
- Tsao CR, Liao MF, Wang MH, et al. Mesenchymal Stem Cell Derived Exosomes: A New Hope for the Treatment of Cardiovascular Disease? Acta Cardiol Sin 2014;30:395-400. [PubMed]
- Feng Y, Huang W, Wani M, et al. Ischemic preconditioning potentiates the protective effect of stem cells through secretion of exosomes by targeting Mecp2 via miR-22. PLoS One 2014;9:e88685. [Crossref] [PubMed]
- Yu B, Gong M, Wang Y, et al. Cardiomyocyte protection by GATA-4 gene engineered mesenchymal stem cells is partially mediated by translocation of miR-221 in microvesicles. PLoS One 2013;8:e73304. [Crossref] [PubMed]
- Arslan F, Lai RC, Smeets MB, et al. Mesenchymal stem cell-derived exosomes increase ATP levels, decrease oxidative stress and activate PI3K/Akt pathway to enhance myocardial viability and prevent adverse remodeling after myocardial ischemia/reperfusion injury. Stem Cell Res 2013;10:301-12. [Crossref] [PubMed]
- Wang X, Gu H, Qin D, et al. Exosomal miR-223 Contributes to Mesenchymal Stem Cell-Elicited Cardioprotection in Polymicrobial Sepsis. Sci Rep 2015;5:13721. [Crossref] [PubMed]
- Chen Y, Zhao Y, Chen W, et al. MicroRNA-133 overexpression promotes the therapeutic efficacy of mesenchymal stem cells on acute myocardial infarction. Stem Cell Res Ther 2017;8:268. [Crossref] [PubMed]
- Qu Q, Pang Y, Zhang C, et al. Exosomes derived from human umbilical cord mesenchymal stem cells inhibit vein graft intimal hyperplasia and accelerate reendothelialization by enhancing endothelial function. Stem Cell Res Ther 2020;11:133. [Crossref] [PubMed]
- Cho YM, Kim JH, Kim M, et al. Mesenchymal stem cells transfer mitochondria to the cells with virtually no mitochondrial function but not with pathogenic mtDNA mutations. PLoS One 2012;7:e32778. [Crossref] [PubMed]
- Spees JL, Olson SD, Whitney MJ, et al. Mitochondrial transfer between cells can rescue aerobic respiration. Proc Natl Acad Sci U S A 2006;103:1283-8. [Crossref] [PubMed]
- Lin HY, Liou CW, Chen SD, et al. Mitochondrial transfer from Wharton's jelly-derived mesenchymal stem cells to mitochondria-defective cells recaptures impaired mitochondrial function. Mitochondrion 2015;22:31-44. [Crossref] [PubMed]
- Acquistapace A, Bru T, Lesault PF, et al. Human mesenchymal stem cells reprogram adult cardiomyocytes toward a progenitor-like state through partial cell fusion and mitochondria transfer. Stem Cells 2011;29:812-24. [Crossref] [PubMed]
- Vallabhaneni KC, Haller H, Dumler I. Vascular smooth muscle cells initiate proliferation of mesenchymal stem cells by mitochondrial transfer via tunneling nanotubes. Stem Cells Dev 2012;21:3104-13. [Crossref] [PubMed]
- Qi CM, Ma GS, Liu NF, et al. Transplantation of magnetically labeled mesenchymal stem cells improves cardiac function in a swine myocardial infarction model. Chin Med J (Engl) 2008;121:544-50. [Crossref] [PubMed]
- Schuleri KH, Feigenbaum GS, Centola M, et al. Autologous mesenchymal stem cells produce reverse remodelling in chronic ischaemic cardiomyopathy. Eur Heart J 2009;30:2722-32. [Crossref] [PubMed]
- Perin EC, Silva GV, Assad JA, et al. Comparison of intracoronary and transendocardial delivery of allogeneic mesenchymal cells in a canine model of acute myocardial infarction. J Mol Cell Cardiol 2008;44:486-95. [Crossref] [PubMed]
- Zhou Y, Wang S, Yu Z, et al. Direct injection of autologous mesenchymal stromal cells improves myocardial function. Biochem Biophys Res Commun 2009;390:902-7. [Crossref] [PubMed]
- Bolli R, Hare JM, Henry TD, et al. Rationale and Design of the SENECA (StEm cell iNjECtion in cAncer survivors) Trial. Am Heart J 2018;201:54-62. [Crossref] [PubMed]
- ClinicalTrials.gov. "ESTIMATION Study" for Endocardial Mesenchymal Stem Cells Implantation in Patients After Acute Myocardial Infarction (ESTIMATION). Retrieved August 19, 2023. Available online: https://classic.clinicaltrials.gov/ct2/show/record/NCT01394432?view=record
- Rodrigo SF, van Ramshorst J, Hoogslag GE, et al. Intramyocardial injection of autologous bone marrow-derived ex vivo expanded mesenchymal stem cells in acute myocardial infarction patients is feasible and safe up to 5 years of follow-up. J Cardiovasc Transl Res 2013;6:816-25. [Crossref] [PubMed]
- Williams AR, Trachtenberg B, Velazquez DL, et al. Intramyocardial stem cell injection in patients with ischemic cardiomyopathy: functional recovery and reverse remodeling. Circ Res 2011;108:792-6. [Crossref] [PubMed]
- Perin EC, Sanz-Ruiz R, Sánchez PL, et al. Adipose-derived regenerative cells in patients with ischemic cardiomyopathy: The PRECISE Trial. Am Heart J 2014;168:88-95.e2. [Crossref] [PubMed]
- Ascheim DD, Gelijns AC, Goldstein D, et al. Mesenchymal precursor cells as adjunctive therapy in recipients of contemporary left ventricular assist devices. Circulation 2014;129:2287-96. [Crossref] [PubMed]
- Curtis MW, Russell B. Cardiac tissue engineering. J Cardiovasc Nurs 2009;24:87-92. [Crossref] [PubMed]
- Tzahor E, Poss KD. Cardiac regeneration strategies: Staying young at heart. Science 2017;356:1035-9. [Crossref] [PubMed]
- Murry CE, Soonpaa MH, Reinecke H, et al. Haematopoietic stem cells do not transdifferentiate into cardiac myocytes in myocardial infarcts. Nature 2004;428:664-8. [Crossref] [PubMed]
- Teng CJ, Luo J, Chiu RC, et al. Massive mechanical loss of microspheres with direct intramyocardial injection in the beating heart: implications for cellular cardiomyoplasty. J Thorac Cardiovasc Surg 2006;132:628-32. [Crossref] [PubMed]
- Zenovich AG, Davis BH, Taylor DA. Comparison of intracardiac cell transplantation: autologous skeletal myoblasts versus bone marrow cells. Handb Exp Pharmacol 2007;117-65. [Crossref] [PubMed]
- Rodrigues ICP, Kaasi A, Maciel Filho R, et al. Cardiac tissue engineering: current state-of-the-art materials, cells and tissue formation. Einstein (Sao Paulo) 2018;16:eRB4538. [Crossref] [PubMed]
- Barker RA, Carpenter MK, Forbes S, et al. The Challenges of First-in-Human Stem Cell Clinical Trials: What Does This Mean for Ethics and Institutional Review Boards? Stem Cell Reports 2018;10:1429-31. [Crossref] [PubMed]
- Nguyen AH, Marsh P, Schmiess-Heine L, et al. Cardiac tissue engineering: state-of-the-art methods and outlook. J Biol Eng 2019;13:57. [Crossref] [PubMed]
- Almouemen N, Kelly HM, O'Leary C. Tissue Engineering: Understanding the Role of Biomaterials and Biophysical Forces on Cell Functionality Through Computational and Structural Biotechnology Analytical Methods. Comput Struct Biotechnol J 2019;17:591-8. [Crossref] [PubMed]
- Ahadian S, Rahal R, Ramón‐Azcón J, et al. Biomaterials in tissue engineering. In: Hasan A. editor. Tissue engineering for artificial organs: regenerative medicine, smart diagnostics and personalized medicine. Hoboken: John Wiley & Sons; 2017:35-83.
- Park JS, Suryaprakash S, Lao YH, et al. Engineering mesenchymal stem cells for regenerative medicine and drug delivery. Methods 2015;84:3-16. [Crossref] [PubMed]
- Varkouhi AK, Monteiro APT, Tsoporis JN, et al. Genetically Modified Mesenchymal Stromal/Stem Cells: Application in Critical Illness. Stem Cell Rev Rep 2020;16:812-27. [Crossref] [PubMed]
- Nowakowski A, Walczak P, Lukomska B, et al. Genetic Engineering of Mesenchymal Stem Cells to Induce Their Migration and Survival. Stem Cells Int 2016;2016:4956063. [Crossref] [PubMed]
- Brandl A, Meyer M, Bechmann V, et al. Oxidative stress induces senescence in human mesenchymal stem cells. Exp Cell Res 2011;317:1541-7. [Crossref] [PubMed]
- Herberg S, Shi X, Johnson MH, et al. Stromal cell-derived factor-1β mediates cell survival through enhancing autophagy in bone marrow-derived mesenchymal stem cells. PLoS One 2013;8:e58207. [Crossref] [PubMed]
- Majmundar AJ, Wong WJ, Simon MC. Hypoxia-inducible factors and the response to hypoxic stress. Mol Cell 2010;40:294-309. [Crossref] [PubMed]
- Huang B, Qian J, Ma J, et al. Myocardial transfection of hypoxia-inducible factor-1α and co-transplantation of mesenchymal stem cells enhance cardiac repair in rats with experimental myocardial infarction. Stem Cell Res Ther 2014;5:22. [Crossref] [PubMed]
- Zhang JH, Adikaram P, Pandey M, et al. Optimization of genome editing through CRISPR-Cas9 engineering. Bioengineered 2016;7:166-74. [Crossref] [PubMed]
- Gerace D, Martiniello-Wilks R, Nassif NT, et al. CRISPR-targeted genome editing of mesenchymal stem cell-derived therapies for type 1 diabetes: a path to clinical success? Stem Cell Res Ther 2017;8:62. [Crossref] [PubMed]
- Li J, Hu S, Zhu D, et al. All Roads Lead to Rome (the Heart): Cell Retention and Outcomes From Various Delivery Routes of Cell Therapy Products to the Heart. J Am Heart Assoc 2021;10:e020402. [Crossref] [PubMed]
- Terrovitis JV, Smith RR, Marbán E. Assessment and optimization of cell engraftment after transplantation into the heart. Circ Res 2010;106:479-94. [Crossref] [PubMed]
- Johnston PV, Sasano T, Mills K, et al. Engraftment, differentiation, and functional benefits of autologous cardiosphere-derived cells in porcine ischemic cardiomyopathy. Circulation 2009;120:1075-83, 7 p following 1083.
- Blocki A, Beyer S, Dewavrin JY, et al. Microcapsules engineered to support mesenchymal stem cell (MSC) survival and proliferation enable long-term retention of MSCs in infarcted myocardium. Biomaterials 2015;53:12-24. [Crossref] [PubMed]
- Ross CJ, Chang PL. Development of small alginate microcapsules for recombinant gene product delivery to the rodent brain. J Biomater Sci Polym Ed 2002;13:953-62. [Crossref] [PubMed]
- Park J, Kim B, Han J, et al. Graphene oxide flakes as a cellular adhesive: prevention of reactive oxygen species mediated death of implanted cells for cardiac repair. ACS Nano 2015;9:4987-99. [Crossref] [PubMed]
- Wu X, Ren J, Li J. Fibrin glue as the cell-delivery vehicle for mesenchymal stromal cells in regenerative medicine. Cytotherapy 2012;14:555-62. [Crossref] [PubMed]
- Theus AS, Tomov ML, Cetnar A, et al. Biomaterial approaches for cardiovascular tissue engineering. Emergent Materials 2019;2:193-207. [Crossref]
- Bernhard JC, Vunjak-Novakovic G. Should we use cells, biomaterials, or tissue engineering for cartilage regeneration? Stem Cell Res Ther 2016;7:56. [Crossref] [PubMed]
- Boffito M, Sartori S, Ciardelli G. Polymeric scaffolds for cardiac tissue engineering: requirements and fabrication technologies. Polymer International 2014;63:2-11. [Crossref]
- Kim KJ, Joe YA, Kim MK, et al. Silica nanoparticles increase human adipose tissue-derived stem cell proliferation through ERK1/2 activation. Int J Nanomedicine 2015;10:2261-72. [Crossref] [PubMed]
- Kai D, Wang QL, Wang HJ, et al. Stem cell-loaded nanofibrous patch promotes the regeneration of infarcted myocardium with functional improvement in rat model. Acta Biomater 2014;10:2727-38. [Crossref] [PubMed]
- Guex AG, Frobert A, Valentin J, et al. Plasma-functionalized electrospun matrix for biograft development and cardiac function stabilization. Acta Biomater 2014;10:2996-3006. [Crossref] [PubMed]
- Jia W, Gungor-Ozkerim PS, Zhang YS, et al. Direct 3D bioprinting of perfusable vascular constructs using a blend bioink. Biomaterials 2016;106:58-68. [Crossref] [PubMed]
- Qasim M, Haq F, Kang MH, et al. 3D printing approaches for cardiac tissue engineering and role of immune modulation in tissue regeneration. Int J Nanomedicine 2019;14:1311-33. [Crossref] [PubMed]
- Song HG, Rumma RT, Ozaki CK, et al. Vascular Tissue Engineering: Progress, Challenges, and Clinical Promise. Cell Stem Cell 2018;22:340-54. [Crossref] [PubMed]
- Kolesky DB, Homan KA, Skylar-Scott MA, et al. Three-dimensional bioprinting of thick vascularized tissues. Proc Natl Acad Sci U S A 2016;113:3179-84. [Crossref] [PubMed]
- Kolesky DB, Truby RL, Gladman AS, et al. 3D bioprinting of vascularized, heterogeneous cell-laden tissue constructs. Adv Mater 2014;26:3124-30. [Crossref] [PubMed]
- Regmi S, Pathak S, Kim JO, et al. Mesenchymal stem cell therapy for the treatment of inflammatory diseases: Challenges, opportunities, and future perspectives. Eur J Cell Biol 2019;98:151041. [Crossref] [PubMed]
- Deuse T, Stubbendorff M, Tang-Quan K, et al. Immunogenicity and immunomodulatory properties of umbilical cord lining mesenchymal stem cells. Cell Transplant 2011;20:655-67. [Crossref] [PubMed]
- Stubbendorff M, Deuse T, Hua X, et al. Immunological properties of extraembryonic human mesenchymal stromal cells derived from gestational tissue. Stem Cells Dev 2013;22:2619-29. [Crossref] [PubMed]
- Nasef A, Chapel A, Fouillard L. Effect of culture environement on mesenchymal stem cell immunomodulatory ability. Stud Stem Cells Res Ther 2020;6:9-15. [Crossref]
- Hagmann S, Moradi B, Frank S, et al. Different culture media affect growth characteristics, surface marker distribution and chondrogenic differentiation of human bone marrow-derived mesenchymal stromal cells. BMC Musculoskelet Disord 2013;14:223. [Crossref] [PubMed]
- Buckley CT, Kelly DJ. Expansion in the presence of FGF-2 enhances the functional development of cartilaginous tissues engineered using infrapatellar fat pad derived MSCs. J Mech Behav Biomed Mater 2012;11:102-11. [Crossref] [PubMed]
- Przybyt E, Harmsen MC. Mesenchymal stem cells: promising for myocardial regeneration? Curr Stem Cell Res Ther 2013;8:270-7. [Crossref] [PubMed]
- Rangappa S, Makkar R, Forrester J. Review article: current status of myocardial regeneration: new cell sources and new strategies. J Cardiovasc Pharmacol Ther 2010;15:338-43. [Crossref] [PubMed]
- Paul A, Ge Y, Prakash S, et al. Microencapsulated stem cells for tissue repairing: implications in cell-based myocardial therapy. Regen Med 2009;4:733-45. [Crossref] [PubMed]
- Al Kindi AH, Asenjo JF, Ge Y, et al. Microencapsulation to reduce mechanical loss of microspheres: implications in myocardial cell therapy. Eur J Cardiothorac Surg 2011;39:241-7. [Crossref] [PubMed]
- Díaz-Herráez P, Garbayo E, Simón-Yarza T, et al. Adipose-derived stem cells combined with neuregulin-1 delivery systems for heart tissue engineering. Eur J Pharm Biopharm 2013;85:143-50. [Crossref] [PubMed]
- Li Y, Wu Q, Wang Y, et al. Senescence of mesenchymal stem cells. Int J Mol Med 2017;39:775-82. [Crossref] [PubMed]
- Takeuchi M, Takeuchi K, Kohara A, et al. Chromosomal instability in human mesenchymal stem cells immortalized with human papilloma virus E6, E7, and hTERT genes. In Vitro Cell Dev Biol Anim 2007;43:129-38. [Crossref] [PubMed]
- Dib N, Khawaja H, Varner S, et al. Cell therapy for cardiovascular disease: a comparison of methods of delivery. J Cardiovasc Transl Res 2011;4:177-81. [Crossref] [PubMed]
- Dib N, Menasche P, Bartunek JJ, et al. Recommendations for successful training on methods of delivery of biologics for cardiac regeneration: a report of the International Society for Cardiovascular Translational Research. JACC Cardiovasc Interv 2010;3:265-75. [Crossref] [PubMed]
- Bartunek J, Wijns W, Heyndrickx GR, et al. Timing of intracoronary bone-marrow-derived stem cell transplantation after ST-elevation myocardial infarction. Nat Clin Pract Cardiovasc Med 2006;3:S52-6. [Crossref] [PubMed]
- Ding DC, Shyu WC, Lin SZ. Mesenchymal stem cells. Cell Transplant 2011;20:5-14. [Crossref] [PubMed]
- Wang HS, Hung SC, Peng ST, et al. Mesenchymal stem cells in the Wharton's jelly of the human umbilical cord. Stem Cells 2004;22:1330-7. [Crossref] [PubMed]
- Karantalis V, Hare JM. Use of mesenchymal stem cells for therapy of cardiac disease. Circ Res 2015;116:1413-30. [Crossref] [PubMed]
- Ocansey DKW, Pei B, Yan Y, et al. Improved therapeutics of modified mesenchymal stem cells: an update. J Transl Med 2020;18:42. [Crossref] [PubMed]
- Volarevic V, Markovic BS, Gazdic M, et al. Ethical and Safety Issues of Stem Cell-Based Therapy. Int J Med Sci 2018;15:36-45. [Crossref] [PubMed]
- Lazennec G, Jorgensen C. Concise review: adult multipotent stromal cells and cancer: risk or benefit? Stem Cells 2008;26:1387-94. [Crossref] [PubMed]
- Zhu W, Xu W, Jiang R, et al. Mesenchymal stem cells derived from bone marrow favor tumor cell growth in vivo. Exp Mol Pathol 2006;80:267-74. [Crossref] [PubMed]
- Ljujic B, Milovanovic M, Volarevic V, et al. Human mesenchymal stem cells creating an immunosuppressive environment and promote breast cancer in mice. Sci Rep 2013;3:2298. [Crossref] [PubMed]
- Batten P, Sarathchandra P, Antoniw JW, et al. Human mesenchymal stem cells induce T cell anergy and downregulate T cell allo-responses via the TH2 pathway: relevance to tissue engineering human heart valves. Tissue Eng 2006;12:2263-73. [Crossref] [PubMed]
- Nagaya N, Kangawa K, Itoh T, et al. Transplantation of mesenchymal stem cells improves cardiac function in a rat model of dilated cardiomyopathy. Circulation 2005;112:1128-35. [Crossref] [PubMed]
- Breitbach M, Bostani T, Roell W, et al. Potential risks of bone marrow cell transplantation into infarcted hearts. Blood 2007;110:1362-9. [Crossref] [PubMed]
- Hare JM, Fishman JE, Gerstenblith G, et al. Comparison of allogeneic vs autologous bone marrow–derived mesenchymal stem cells delivered by transendocardial injection in patients with ischemic cardiomyopathy: the POSEIDON randomized trial. JAMA 2012;308:2369-79. [Crossref] [PubMed]
- van den Akker F, Feyen DA, van den Hoogen P, et al. Intramyocardial stem cell injection: go(ne) with the flow. Eur Heart J 2017;38:184-6. [PubMed]
- Huang P, Tian X, Li Q, et al. New strategies for improving stem cell therapy in ischemic heart disease. Heart Fail Rev 2016;21:737-52. [Crossref] [PubMed]
- Cheng K, Shen D, Hensley MT, et al. Magnetic antibody-linked nanomatchmakers for therapeutic cell targeting. Nat Commun 2014;5:4880. [Crossref] [PubMed]
- Karpov AA, Udalova DV, Pliss MG, et al. Can the outcomes of mesenchymal stem cell-based therapy for myocardial infarction be improved? Providing weapons and armour to cells. Cell Prolif 2017;50:e12316. [Crossref] [PubMed]
- Ahmed ESA, Ahmed NH, Medhat AM, et al. Mesenchymal stem cells targeting PI3K/AKT pathway in leukemic model. Tumour Biol 2019;41:1010428319846803. [Crossref] [PubMed]
- Giallongo C, Tibullo D, Camiolo G, et al. TLR4 signaling drives mesenchymal stromal cells commitment to promote tumor microenvironment transformation in multiple myeloma. Cell Death Dis 2019;10:704. Erratum in: Cell Death Dis 2019;10:820. [Crossref] [PubMed]
- Cai H, Wu FY, Wang QL, et al. Self-assembling peptide modified with QHREDGS as a novel delivery system for mesenchymal stem cell transplantation after myocardial infarction. FASEB J 2019;33:8306-20. [Crossref] [PubMed]
- Brewster BD, Rouch JD, Wang M, et al. Toll-like receptor 4 ablation improves stem cell survival after hypoxic injury. J Surg Res 2012;177:330-3. [Crossref] [PubMed]
- Bostjancic E, Zidar N, Stajer D, et al. MicroRNAs miR-1, miR-133a, miR-133b and miR-208 are dysregulated in human myocardial infarction. Cardiology 2010;115:163-9. [Crossref] [PubMed]
- Yu BT, Yu N, Wang Y, et al. Role of miR-133a in regulating TGF-β1 signaling pathway in myocardial fibrosis after acute myocardial infarction in rats. Eur Rev Med Pharmacol Sci 2019;23:8588-97. [PubMed]
- Zhu W, Sun L, Zhao P, et al. Macrophage migration inhibitory factor facilitates the therapeutic efficacy of mesenchymal stem cells derived exosomes in acute myocardial infarction through upregulating miR-133a-3p. J Nanobiotechnology 2021;19:61. [Crossref] [PubMed]
- Dakhlallah D, Zhang J, Yu L, et al. MicroRNA-133a engineered mesenchymal stem cells augment cardiac function and cell survival in the infarct heart. J Cardiovasc Pharmacol 2015;65:241-51. [Crossref] [PubMed]
- Gong X, Fan G, Wang W, et al. Trimetazidine protects umbilical cord mesenchymal stem cells against hypoxia and serum deprivation induced apoptosis by activation of Akt. Cell Physiol Biochem 2014;34:2245-55. [Crossref] [PubMed]
- Xu H, Zhu G, Tian Y. Protective effects of trimetazidine on bone marrow mesenchymal stem cells viability in an ex vivo model of hypoxia and in vivo model of locally myocardial ischemia. J Huazhong Univ Sci Technolog Med Sci 2012;32:36-41. [Crossref] [PubMed]
- Han D, Huang W, Li X, et al. Melatonin facilitates adipose-derived mesenchymal stem cells to repair the murine infarcted heart via the SIRT1 signaling pathway. J Pineal Res 2016;60:178-92. [Crossref] [PubMed]
- Liu C, Fan Y, Zhou L, et al. Pretreatment of mesenchymal stem cells with angiotensin II enhances paracrine effects, angiogenesis, gap junction formation and therapeutic efficacy for myocardial infarction. Int J Cardiol 2015;188:22-32. [Crossref] [PubMed]
- Zhang F, Cui J, Lv B, et al. Nicorandil protects mesenchymal stem cells against hypoxia and serum deprivation-induced apoptosis. Int J Mol Med 2015;36:415-23. Erratum in: Int J Mol Med 2020;46:2280-1. [Crossref] [PubMed]
- Lin M, Liu X, Zheng H, et al. IGF-1 enhances BMSC viability, migration, and anti-apoptosis in myocardial infarction via secreted frizzled-related protein 2 pathway. Stem Cell Res Ther 2020;11:22. [Crossref] [PubMed]
- Haider HKh, Jiang S, Idris NM, et al. IGF-1-overexpressing mesenchymal stem cells accelerate bone marrow stem cell mobilization via paracrine activation of SDF-1alpha/CXCR4 signaling to promote myocardial repair. Circ Res 2008;103:1300-8. [Crossref] [PubMed]
- Guo J, Zheng D, Li WF, et al. Insulin-like growth factor 1 treatment of MSCs attenuates inflammation and cardiac dysfunction following MI. Inflammation 2014;37:2156-63. [Crossref] [PubMed]
- Henning RJ. Stem cells in cardiac repair-recent developments and future directions. Intervent Cardiol 2012;7:10-3. [Crossref]
- Mei X, Cheng K. Recent Development in Therapeutic Cardiac Patches. Front Cardiovasc Med 2020;7:610364. [Crossref] [PubMed]
- Fernández-Santos ME, Garcia-Arranz M, Andreu EJ, et al. Optimization of Mesenchymal Stromal Cell (MSC) Manufacturing Processes for a Better Therapeutic Outcome. Front Immunol 2022;13:918565. [Crossref] [PubMed]
- Butler J, Epstein SE, Greene SJ, et al. Intravenous Allogeneic Mesenchymal Stem Cells for Nonischemic Cardiomyopathy: Safety and Efficacy Results of a Phase II-A Randomized Trial. Circ Res 2017;120:332-40. [Crossref] [PubMed]
- Thalakiriyawa DS, Jayasooriya PR, Dissanayaka WL. Regenerative Potential of Mesenchymal Stem Cell-Derived Extracellular Vesicles. Curr Mol Med 2022;22:98-119. [Crossref] [PubMed]
- Lavrentieva A, Hoffmann A, Lee-Thedieck C. Limited Potential or Unfavorable Manipulations? Strategies Toward Efficient Mesenchymal Stem/Stromal Cell Applications. Front Cell Dev Biol 2020;8:316. [Crossref] [PubMed]
- Zhou T, Yuan Z, Weng J, et al. Challenges and advances in clinical applications of mesenchymal stromal cells. J Hematol Oncol 2021;14:24. [Crossref] [PubMed]
- Childs PG, Reid S, Salmeron-Sanchez M, et al. Hurdles to uptake of mesenchymal stem cells and their progenitors in therapeutic products. Biochem J 2020;477:3349-66. [Crossref] [PubMed]
- Musiał-Wysocka A, Kot M, Majka M. The Pros and Cons of Mesenchymal Stem Cell-Based Therapies. Cell Transplant 2019;28:801-12. [Crossref] [PubMed]
- Bagno L, Hatzistergos KE, Balkan W, et al. Mesenchymal Stem Cell-Based Therapy for Cardiovascular Disease: Progress and Challenges. Mol Ther 2018;26:1610-23. [Crossref] [PubMed]
- Lightner AL, Chan T. Precision regenerative medicine. Stem Cell Res Ther 2021;12:39. [Crossref] [PubMed]
- Rossi F, Noren H, Sarria L, et al. Combination therapies enhance immunoregulatory properties of MIAMI cells. Stem Cell Res Ther 2019;10:395. [Crossref] [PubMed]
- Natsumeda M, Florea V, Rieger AC, et al. A Combination of Allogeneic Stem Cells Promotes Cardiac Regeneration. J Am Coll Cardiol 2017;70:2504-15. [Crossref] [PubMed]
- Patel AN, Henry TD, Quyyumi AA, et al. Ixmyelocel-T for patients with ischaemic heart failure: a prospective randomised double-blind trial. Lancet 2016;387:2412-21. [Crossref] [PubMed]