Potential use of stem cell therapies for treating osteoarthritis and rheumatoid arthritis
Introduction
Arthritis, one of the most common pathological conditions causing prolonged pain and frequently leading to disability, is recorded in almost all parts of the world including the United States, and its occurrence frequency is likely to increase in the future. Both of the health monitoring agencies of the US, the Center for Disease Control and Prevention (CDC), and the National Health Interview Survey (NHIS), have estimated some degree of its prevalence in about 58.5 million adults (1). Another study has estimated that by 2040, 78.4 million adults in the USA would be suffering from some type of clinically confirmed arthritis (2). This condition is associated with mild to excruciating pain leading to mobility and activity limitations, resulting in declining working ability, productivity, social activity, and quality of life. In other words, arthritis has severe impacts on the economic, psychological, and emotional health on the patient and their relatives. Arthritis encompasses a group of medical problems rather than a single disease generally characterized by inflamed joints, related pain and discomfort. Many types of arthritis have been documented, such as gout arthritis (GA), ankylosing spondylitis (AS), lupus arthritis (LA), infectious arthritis (IA), juvenile arthritis (JA), psoriatic arthritis (PA), and fibromyalgia. Out of these, osteoarthritis (OA) and rheumatoid arthritis (RA) are the two most prevalent categories of the disease. Conditions such as GA, AS, LA, PA, and RA are considered to be autoimmune diseases while OA and fibromyalgia show varying degrees of inflammation in confirmed cases of the disease (3).
The widespread undesired prevalence of OA is emerging as a serious medical concern at a global level. In 2017, approximately 303 million people across the globe were thought to be afflicted by some degree of this condition. In the United States alone, it is estimated that 14 million individuals suffer from some form and degree of the disease. Although it can affect any joint of the body, more likely it affects knees, hands, hips, and the spine and related discomforts (4,5). The appearance of OA is often seen to start at an early median age of 40 years, usually accompanied by the degeneration of cartilage within bone joints. The presence of other comorbidities such as obesity, diabetes, or other similar factors further increases the chances of occurrence and severity of the disease. OA mostly occurs following more than normal stiffness in cartilages, leading to losses in their elasticity, and consequently, those can no longer act as proper force absorbers. Other related symptoms like reduced ranges of motion, crepitus, joint instability, swelling, muscle weakness, and fatigue may develop (3,6,7). As an outcome in due course, bones start rubbing against each other causing excruciating pain, and related other sufferings.
Similarly, RA is characterized primarily by chronic pain, and then by joint tissue destruction which normally progresses from distal to more proximal joints, especially in the hands and feet. It is estimated that this disease affects about 1.5 million adults in the USA itself, and appears that women are three times more prone to develop RA than men (4). Contrary to OA, this condition results from systemic chronic inflammation sometimes acting in association with cellular autoreactivity (8). In all cases of arthritis, patients experience decreased flexibility, swelling in surrounding tissues, and pain in the joints. It is a known fact that during RA, the immune system attacks the cartilages, and thus, generates and aggravates the problem. In this autoimmune disease, inflammation can damage cartilages, as well as bones, resulting in swelling and pain in and around the joints, more commonly affecting the joints of hands, feet, wrists, elbows, knees, and ankles (3,4,9).
Recently, mesenchymal stem cells (MSCs), pluripotent stem cells with the ability to differentiate into cells of the mesodermal lineage, have emerged as a successful therapeutic option for treating various diseases. In addition to their ability to differentiate into cells of multiple lineages, MSCs have shown remarkable immunomodulatory properties, which make them an ideal option for treating diseases rooted in inflammation, or autoimmune conditions such as OA and RA. As the progress in research in MSCs-mediated therapies continues, using stem cells in both of the conditions appears to be a feasible option with very promising outlooks. An attempt has been made in this manuscript to describe the mechanisms induced by the MSC therapy including how these cells regulate the inflammation observed in joints of arthritis patients.
Pathogenesis of OA
Several factors like acute trauma, obesity, and genetic predisposition are often associated with the development of OA. Joints of the patients suffering from this disease may exhibit different clinical and biochemical profiles compared to that of healthy ones, which include breakdown of cartilage, thickening of the subchondral bone, synovial inflammation, narrowing of joint spaces, hypertrophy of the joint capsules to name a few (10). Patients suffering from OA develop synovial lining hyperplasia, sublining fibrosis, and stromal vascularization. Moreover, during the pathogenesis of OA, altered concentrations of hyaluronic acid and lubricin negatively affect cartilage integrity, resulting in increased friction in the joints. At this stage, the synovial membrane also produces proinflammatory and catabolic agents, such as matrix metalloproteinases (MMPs) and aggrecanases, which further contribute to the degradation of the articular matrix (11,12).
During the underlying inflammatory processes, different regulatory pathways have been described to be active leading to OA. The biochemical mediators released into the joints sensitize the primary nerves in such a way that even slight joint movements provoke a pain cascade. Eventually, the increased neuronal activity from the periphery can cause changes in the central nervous system by a process named “wind-up”, leading to intensified pain even in other unrelated parts of the body (6). Some of the most common biomarkers found in synovial fluid of such patients are inflammatory cytokines and chemokines secreted by chondrocytes, macrophages and other cells present in the joints. Uncontrolled M1 polarized macrophages in the synovium can lead to the secretion of a number of cytokines, growth factors, MMPs, and tissue inhibitors of metalloproteinases (TIMPs) perpetuating the inflammatory process along with the cartilage and osteocyte destruction. Not many systematic studies have been performed regarding the complex roles of macrophages in OA underscoring the fact that more research is needed to elucidate the signaling pathways involved and participation of both M1 and M2 in the process (13,14).
Cytokines such as tumor necrosis factor (TNF)-α, interleukin (IL)-1β, IL-6, IL-2, IL-7, IL-15, and leukemia inhibitory factor (LIF) have been directly implicated in OA development and its progression (15). Recently, Kisand et al. characterized the cytokine profiles in knees during different stages of OA. Early dysregulations in cytokine profiles show significant differences between the genders. In women, OA was associated with various angiogenesis-related cytokines, in particular ANG1, C-X-C motif chemokine ligand (CXCL)-10/IP-10, PDGF-BB, TSP2, FGF1, RANTES, and MIP1b, while in men tissue-remodeling cytokines like TIMP4 and TIMP2, along with the tissue-injury associated cytokine MIC1 were predominantly present. Though, both sexes displayed higher than normal levels of CXCL-10/IP-10 and other common proinflammatory cytokines including TNFs, IL-1, IL-6, IL-10, IL-17 along their regulators (16,17).
Factors like IL-1β and TNF-α play active roles in the pathobiology of the disease. While elevated levels of IL-1β are associated with cartilage destruction, while TNF-α induces the inflammatory cascade. Both of these biomolecules can be produced by chondrocytes, mononuclear cells, osteoblasts, and synovial tissue, and can act with each other or even independently to initiate the proinflammatory processes (15,18). It has also been reported that IL-1β and TNF-α downregulate the synthesis of components of the major extracellular matrix (ECM) by the inhibition of anabolic activities in the chondrocytes. At the same time, both of the cytokines can stimulate the release of different proteolytic MMPs including MMP-1 (interstitial collagenase), MMP-3 (stromelysin 1), and MMP-13 (collagenase 3), which in combination with the released nitric oxide (NO) and prostaglandin E2 (PGE2) can result in further destruction of the cartilages (15,18,19). Moreover, both of the cytokines also induce the production of reactive oxygen species (ROS), while downregulating the expression of the antioxidant enzymes like superoxide dismutase, catalase, and glutathione peroxidase, and thus accelerate the damage inflicted on the cartilage tissue (20,21).
The Wnt/β-Catenin signaling cascade, commonly activated during OA, strictly regulates chondrogenesis and chondrocyte maturation during cartilage development. Its excessive activation by IL-1β is believed to be one of the root causes responsible for the progression of OA (10,22,23). Elevated expression of Wnt pathway activator, Wnt 1-inducible-signaling pathway protein 1 (WISP-1), usually observed in OA, tissues induce cartilage degradation via the upregulation of MMPs and aggrecanases in chondrocytes and macrophages. Activation of this pathway promotes the IL-1β-mediated matrix degradation as it stimulates MMP expression in articular chondrocytes (24). Decreased expression of some of the Wnt pathway suppressors, such as sclerostin, dickkopf WNT signaling pathway inhibitor 1 (DKK1), and secreted frizzled-related protein 3 (sFRP3) further facilitate OA progression. It is logical to infer that the inhibition of MMPs and the downregulation of proinflammatory cytokines could have potential therapeutic effects in such patients. Regulating these pathways with potential drugs has proven to alleviate cartilage destruction in OA models (10,18,25-27). A couple of small clinical trials have used adalimumab and infliximab in search of a specific drug targeting the TNF and directly related pathways. Unfortunately, the findings of the studies were inconclusive (18).
Pathogenesis of RA
According to the United States National Health and Nutrition Examination Survey (NHANES) database, advancing age, smoking, diabetes, and osteoporosis are related to higher risks of developing RA. Aging is considered to be one of the primary contributing elements in addition to other genetic and environmental factors (28,29). A gender bias has been recorded regarding the RA occurrence as it has a three times higher prevalence in women than men of 40 to 60 years age group, and it is very rare in children. This condition can affect different parts of the body simultaneously, usually starting from smaller distal joints like wrists, ankles, and feet, and then spreading to elbows, knees, hips, neck, or shoulders (3,9).
A number of factors are thought to play some degree of roles in causing and promoting RA. Autoimmune processes often initiate the disintegration in cartilaginous tissues recorded in RA patients. In this condition, increased levels of proinflammatory biomolecules cause chronic inflammation in the synovium leading to progressive destruction of collagen, and narrowing of the joint spaces, which eventually leads to bone damage. Synovial fluid containing immune systems cells like synovial fibroblasts, macrophages, mast cells, CD4+T cells, CD8+T cells, natural killer (NK) cells, T-cells, B-cells, and plasma cells can get accumulated in the synovium, thus, producing a growth of thickened heterogeneous synovial tissue (28). Consequentially this may produce more of the enzymes responsible for tissue destruction, and therefore, can aggravate the patient’s condition (30). Elevated numbers of neutrophils are also present in the synovial fluid trafficking through the synovial membrane, activated by immune complexes, complement components, and cytokines which release a number of chemokines, prostaglandins, and reactive oxygen intermediates, and thus, contribute to the general hypoxic milieu observed in inflamed joints (28,31). Mast cells, normally involved in innate and adaptive synovial immunity, can be numerously found in the synovial tissues of patients with RA. These cells are known to express a variety of proinflammatory cytokines and proteases like TNF-α, IL-1β, IL-6, interferon (IFN)-γ, PDGFβ, RANTES, among others (32). In addition, the presence and activity of macrophages, specifically the M1 type, is central in perpetuating the development of RA. The M1 macrophages stimulate neovascularization and promote the secretion of proteases as these cells express and produce high levels of proinflammatory cytokines and proteins such as PHD3, CCR2, MMP12, TNF-α, IFN-γ, IL-1, IL-6, C-C motif chemokine ligand 5 (CCL5), CXCL-1, and CXCL-10, while suppressing the polarization of M2 macrophages (33). These cytokines further promote the expression of MMPs like MMP-1, MMP-2, MMP-3, MMP-8, MMP-13, among others. Together, these molecules are largely responsible for the destruction of the cartilages, bones, and tendons in the arthritic joints (34).
Elevated proinflammatory cytokine activities are also involved at each stage of RA development by contributing to the autoimmune response causing chronic inflammation and tissue damage, similar to that observed in OA (28). In comparison to normal counterparts, the synovial fluid of patients with RA presents significantly elevated levels of several chemokines related to immune responses such as eotaxin, hGROα, IL-8, MCP-2, RANTES, TARC, and IL-6 (35). In addition, elevated levels of other proinflammatory cytokines like TNF-α, IL-1β, -6, -7, -8, -15, -18, and IL-23p19 are also present. Though, TNF-α has a potent effect on osteoclast formation, but it can inhibit osteoblast differentiation and functions, thus, moving the balance towards bone destruction (28,36,37). This pleiotropic cytokine has an important role in autoimmunity, primarily through the inhibition of regulatory T-cells, and it has been implicated in the pathogenesis of many inflammatory diseases, including RA (38,39). Along with their own specific functions, cytokines act in synergy to initiate the inflammatory process, as well as in maintaining their own production. Moreover, these additive effects are also extended to induce the production of other chemokines, such as CCL20, thereby, attracting more of the Th17 cells into the affected sites (39,40). Interactions of all these biomolecules result in the activation of the STAT signaling pathway, eventually leading to the over-activation of the JAK/STAT signaling pathway contributing to the perpetuation of the inflammation process, and thus resulting in progressive joint degeneration. Activated SAPK/MAPK and PI-3K/AKT/mTOR cell signaling pathways have also been described to contribute to the RA pathogenesis (41).
High levels of rheumatoid factor (RF) and anti-citrullinated peptide antibodies (ACPAs) are also reported to contribute to bone and cartilage destruction. Although RF can be found in healthy and non-RA patients, their presence appears to be transient, and in some cases even protective. High levels of RF in patients suffering from RA and other autoimmune conditions is reported to be as high as 90% in patients with detrimental effects (42). Similarly, ACPA, which acts against citrullinated peptides and proteins, has an important role in autoimmunity. In RA patients, ACPAs are reported to interact with osteocytes and chondrocytes in the articular cavity and initiate inflammatory processes via the activation of macrophages leading to bone destruction (43). Therefore, approaches based on both of the molecules have been used to perform a more personalized medical intervention in the early diagnosis of RA, and even in some cases for the prognosis for therapeutic outcomes (42-44). As seen in OA, enhanced M1 polarization plays important roles in the development and progression of RA mostly due to the pro-inflammatory cytokines released by these cells. More importantly, it has been observed that M1 polarization is highly related to age, which only complicates the condition since RA is mostly diagnosed in the elderly population (45,46).
Several approaches for treating RA by directly targeting cytokines or the processes induced by those signaling factors like cell proliferation and metabolic routes involved in the pathogenesis are being tried. Different clinical trials have been approved with moderate results on the effects. For example, administration of Tofacitinib is prescribed to suppress the hyper activation of JAK-STAT pathway, commonly found in RA patients (47). Combinations of treatments including TNF-α inhibiting monotherapy, methotrexate as an immune suppressor, anti-IL-6-receptor binding inhibitory, anti-T, and anti-B cell agents, have proven to be helpful but not sufficient (48). The aforementioned treatment methods have been used for treating OA and RA usually alleviating the pain in both conditions. However, none of them resulted in consistently definitive improvements.
Mechanisms of actions of MSCs
Stem cells are partially differentiated cells with the potential to proliferate indefinitely and differentiate into cells of the same lineage family. MSCs are pluripotent stem cells commonly found in distinct tissues including bone marrow and visceral fat; able to transform cell types of the mesodermal lineage. Although the precise mechanisms by which MSCs help heal have not been deciphered completely yet, different theories regarding the mechanisms have been put forward to explain the therapeutic effects recorded after the use of MSCs for treating different pathological conditions. MSCs, in addition to the ability to differentiate in different types of cells, secrete a number of growth factors, anti-inflammatory cytokines that can help in the promotion of angiogenesis and anti-fibrosis. Recent studies confirm that one of the main mechanisms of action by which the MSCs act is their immense ability of immunomodulation (49-53). These cells have the remarkable capacity to interact with the patient’s own microenvironment, and thereby, regulate the actions of the cytokines involved in the inflammatory process in a diversity of conditions (54,55) ultimately leading to tissue regeneration and restoration of organ function. It is important to mention that the MSCs are found in almost all post-natal organs and tissues like adipose tissue, Wharton’s jelly, bone marrow, peripheral blood, cord blood, dental pulp, and periodontal ligament, including the perivascular niches, among others (52,56-58). Although each population of cells has varying capacities for differentiation, distinct morphology, and growth potential, all of them have potent immunomodulatory properties and chondrogenic potential (59). Consequently, considering the dysregulation of cytokines and the inherent inflammatory process found in both types of arthritis described above, MSC therapy could be a feasible treatment for patients suffering from both OA and RA.
Evidence suggests that MSCs are effective in modulating the immune response in situations where proinflammatory molecules are involved (60). A variety of researchers report that MSCs have inhibitory effects on the activity and production of proinflammatory cytokines, including TNF-α, IFN-γ, IL-1β, IL-2, IL-17, CCL5, and CSCLC2. Moreover, the administration of MSCs also stimulates the secretion of antiinflammatory cytokines like IL-5, IL-10, and IL-13; while inducing the expression of transforming growth factor (TGF-β), IDO, PGE2, and programmed death-ligand 1 (PDL-1) (61). Keeping the immunomodulatory effects observed in MSCs, it is possible to postulate that the downregulation of the Wnt/β-Catenin signaling cascade leads to the regeneration of cartilage observed after the administration of MSCs. However, more research is needed to elucidate the exact underlying mechanisms behind the multifaceted signaling routes.
Available data indicate that MSCs have both of the abilities which are inhibiting and activating the proliferation of immune cells, including Follicular T helper (Tfh), Th1, Th17, CD8 lymphocytes, and M1 macrophages. MSCs can prevent the infiltration of proinflammatory cells into the cartilage, thus, can reduce the levels of inflammatory cytokines such as TNF-α, IFN-γ, IL-1β, IL-2, and IL-17 while slowing down the degradation of type II collagen caused by the MMPs produced by synovial cells and chondrocytes (61). Similarly, MSCs promote the proliferation of many immune regulatory cells including Th17, T-regulatory (Treg), and Type 1 regulatory (TR1) lymphocytes while suppressing polarization of macrophages to M1 cells, and favoring the M2 polarization leading to higher levels of antiinflammatory cytokines like IL-5, IL-10, and IL-13 (61,62) (Figure 1). Results of a recent study suggest that the cytokine environment found in patients strongly influences the behavior of MSCs to regulate the functions of macrophages, preventing excessive inflammatory actions that could possibly impair the process of tissue healing (63). Eventually, this leads to regaining the balance between proinflammatory and antiinflammatory memory T cell populations (62).
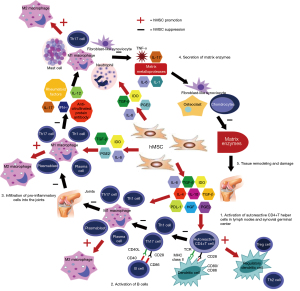
Another important property of the MSCs is their response to the proinflammatory cytokines, especially to IFN-γ which is marked by the increased production of indoleamine 2,3 dioxygenase (IDO). This immunosuppressive molecule increases the catabolism of tryptophan and the production of kynurenines. These increased levels of kynurenine result in cell cycle arrest and cellular death of T cells, thus, favor the production of Treg cells (64). Moreover, elevated levels of proinflammatory cytokines could be prompting the MSCs to produce another signaling molecule known as suppressor of cytokine signaling 1 (SOCS1), which inhibits the JAK activity by binding to the catalytic site of JAK in addition to recruiting the ubiquitin-transferase complex for proteasomal degradation (65). Ultimately, infusion of the MSCs results in the regulation of proinflammatory cytokine-induced inflammatory processes, thereby alleviates the caused discomfort.
Although more research is needed to elucidate the mechanisms of MSCs, recent studies report another therapeutic property found in MSCs. In the presence of cytotoxic cells, as commonly found in autoimmune conditions such as RA, it is reported that MSCs show significant activation of the caspase pathway leading to apoptosis. It appears that this process is necessary to exert the immunosuppressive therapeutic properties of the cells. One possible explanation is that by engulfing apoptotic MSCs, macrophages produce IDO and ultimately deliver the MSCs mediated immunosuppressive effects (66,67).
Findings from pre-clinical and clinical trials
Several studies regarding the use of MSCs for OA in animal models including mice, rats, rabbits, goats, and sheep have reported excellent outcomes. Intra-articular injections of adipose-derived MSCs (adMSCs) have been shown to decrease the thickness of the synovial lining, which could be attributed to their interactions with synovial macrophages. Following the infusion of adMSCs in the collateral ligaments or in the cruciate ligaments of the OA mouse knee joints showed significant improvements through chondrogenesis (68). In addition, multiple administration of synovial MSCs (syMSCs) has proven to be more effective than a single dose in stopping the progression of OA in a study carried out in a rat model of the disease. Weekly intra-articular injections of syMSCs significantly upregulated several cytokines like PRG-4 and BMPs which are related to chondro-protection in the articular area. At the same time, the upregulation of TSG-6 following the MSC administration delayed cartilage degradation through attenuating synovitis (69). In a very extensive recent study, intra-articular injection of 12×106±3.2×106 allogeneic MSCs was reported to have long-term safety results in the canine model of OA. Temporary local inflammation in two animals was reported to be the only adverse event, which lasted for less than a week after the injection. Therapeutic outcomes of a single dose of MSCs were reported to last as long as 4–5 years as evaluated at regular follow-ups, showing a very promising result with no secondary effects (70).
Recent studies in animal models of RA report reductions in the levels of serum vascular endothelial growth factor (VEGF), an inflammatory marker, and in the pro-coagulant tissue factor (TF), after the administration of human MSCs. MSCs therapy counteracts the effects of ROS by significantly increasing the levels of the superoxide dismutase (SOD), glutathione peroxidase (GSH-Px), the total antioxidant capacity (tAOC), and by reducing the level of malondialdehyde (MDA) (71). Also, administrations of umbilical cord-derived MSCs (ucMSCs) have proven to shift the macrophage phenotype from the CD14+, CD86+ M1 to the CD14+, CD206+ M2 type, thus contributing to a reduction in the inflammatory process (72). As a synergistic result, a reduction in the severity of arthritis was observed in RA animal models, confirmed by the histological observations. In a very recent study, systemic administration of ucMSC as well as bone marrow-derived MSCs (bmMSC) has proven to reduce the levels of TNF-α and IL1-β in the synovial fluid, evident by histological observations showing reduced synovitis and articular destruction (73). It is important to mention that very few studies have been published regarding the MSC therapy on rheumatoid animal models. A recent study in a canine model of the disease had shown excellent outcomes. The therapy protocol was the intravascular administration of 5×105 allogeneic adMSCs performed once a month for a period of 11 months. Although the analyses performed are limited, clinical signs of RA improvement were registered without any adverse effects during the treatment. The authors of this first trial in veterinary medicine for treating RA with MSC therapy suggest that it can substitute completely or can be complementary to current medical treatments for this condition and to other immune related diseases (74).
Considering the immunomodulatory properties of MSCs and conclusions derived from the histological results following the administration observed in animal models, it is logical to infer that this therapy could be an excellent approach for treating both OA and RA in patients. Several clinical trials have reported promising results in treating these conditions. In a 5-year follow-up study, three patients between ages 54 to 65, diagnosed with moderate to severe OA, received 8×106–9×106 autologous bone-marrow-derived MSCs via intra-articular injection. The patients showed significant improvements in pain, functions of joints, and walking abilities through almost all of the beneficial effects were observed in the first 6 months following the administration of MSCs. Interestingly, the improvements stayed noticeably significant for an extended duration of 5 years (75). In a similar study, the administration of 20×106–24×106 bmMSCs, resulted in increased cartilage thickness, along with the decrease of edematous subchondral patches eventually leading to an appreciable decrease in general pain. Significant improvements in the ability for walking were also observed up to 6 months after the injection, which appeared to decrease from 6 to 12 months after the treatment. These findings suggest that a second reinforcement application would probably be necessary for the long-lasting curative effects of the MSC administration (76). Moreover, in a fairly complete clinical trial, a comparative study on the effects, administration of low-, moderate- and high-doses of adMSC was performed. Patients enrolled received intra-articular injections of 1×107 or 5×107 or 1×108 cells suspended in 3 mL of saline. Based on clinical, radiological, and arthroscopic results, administration of the highest number of MSCs showed better results than the other two doses. Nevertheless, increasing the number of cells injected could raise concerns regarding the formation of fibrous foreign bodies. Therefore, repetition of administration in a lower number of cells could be a safer approach with better outcomes than injecting a very large number of cells at one time (77). In general, it is clear that interactions of MSCs with the patient’s microenvironment are complex, which ultimately determine the extent of cartilage regeneration. Cytokines and growth factors including stanniocalcin-1 (STC-1), basic fibroblast growth factor (bFGF), age-related macular degeneration (AMD), hepatocyte growth factor (HGF), TIMP, TSP2, PGE2, and contents of extracellular vesicles are reported to have anti-apoptotic, anti-fibrotic, chondrogenic, and immunosuppressive activities in cartilage protection in OA patients (78).
Other clinical trials also have reported promising results regarding treating RA using MSCs. A study reported that the administration of 4×107 ucMSCs resulted in significant improvements within the 12 hours following the injection (79). In addition, other related symptoms like joint pain and inflammation were alleviated. Clinical analysis showed decreased levels of inflammatory cytokines and chemokines including TNF-α and IL-6, increased percentage of Treg cells in the peripheral blood, and upregulation in the number of IL-4-producing Th2 cells in the group treated with MSCs. The study confirmed the safety and efficacy of MSC administration for RA patients on one hand, and demonstrated a significant duration of the therapeutic effects, which lasted for at least 3 months (79). In another extensive investigation, 105 patients with active RA and deficient response to traditional medication were divided into MSC therapy and control groups. Patients received one dose of 1×106 cells/kg of ucMSC via intravenous route, and the evaluation of outcomes was performed 12 weeks later. Increases in the IL-10 levels and the Treg/Th17 ratios were observed along with the reductions in the IL-6 levels, confirming the immunomodulatory properties of the MSCs. Furthermore, a positive correlation between MSC therapy-mediated lowering of the higher-than-normal levels of IFN-γ was observed in patients. This finding further confirms the anti-inflammatory function of the MSC and also encourages using the IFN-γ levels as a biomarker in the patients suffering from the disease (80). Another clinical trial trying to confirm the safety of this novel therapy was carried out on 53 patients diagnosed with RA. The patients received intravenous administration of a varying number of adMSCs per kilogram of body weight, or placebo. The study only evaluated the efficacy in the exploratory context, and safety for using the cells and every group receiving MSCs showed general overall improvements (62,81). According to Lopez-Santalla in 2022, only nine clinical trials have been completed, though their results had not been published yet. It is important to note that almost all of the studies, in general, were aiming to establish the safety and efficacy of MSC therapy for treating arthritis (82).
A number of clinical trials using stem cells in OA have been attempted. By the time of preparation of this manuscript 49 clinical trials have been completed out of 131 studies registered at Clinicaltrials.gov website. Promising results, especially regarding treating OA of the knees recorded in those studies, show very useful clinical importance of the stem cell therapy. Similarly, 152 clinical trials are registered for RA and MSC therapy out of which 52 have been completed with promising outcomes and no secondary effects.
Conclusions
Arthritis is one of the most common disability causing pathological conditions, more often recorded in the aging populations worldwide including the United States. Besides mobility limitations, the socio-economic burden has severe impacts on psychological and emotional health, affecting the quality of life. Although the underlying causes for the conditions differ, the initiation and prolongation of the inflammatory process are comparable. Elevated levels of proinflammatory cytokines accompanied with the excessive production of MMPs aggravate the conditions and perpetuate the degradation of cartilages, bones, and tendons of the joints.
MSC therapy has proven to be efficient and safe for treating a variety of diseases, including arthritis. Through cell communication, MSCs could reestablish the biochemical balance lost in OA and RA patients. For instance, MSCs have inhibitory effects on the production of proinflammatory cytokines while inducing expression of antiinflammatory genes. In addition, MSCs have very strong antioxidant properties (61) as well as are able to suppress the macrophage polarization and regulate the proliferation of Treg, Th7, and TR1 lymphocytes, properties further contributing to their immunomodulatory abilities (61,62). Additionally, patients responding poorly to traditional medications, have also been shown significant improvements after the administration of MSCs (80), evident by the reductions in the proinflammatory cytokine levels were observed (79,80), accompanied by the relief of pain and general long-term health improvements (75,76,79,81,82).
The prospect of using MSC therapy looks promising for treating arthritis. Despite numerous findings demonstrating the beneficial effect of MSC therapy in arthritis patients, some concerns regarding the dose and routes of administration still linger. These concerns demand more research regarding the optimal dosage, administration routes, and one-dose vs. periodic administrations. It has been suggested by several studies, that intra-articular administration of the cells in cases of OA preserve the MSC population within the affected joints. Considering the autoimmune nature of RA, intravenous administration of MSCs in addition to the intra-articular route could be highly beneficial to these types of patients. Keeping the immunomodulatory properties of MSCs, along with their ability to promote tissue regeneration in mind, MSC therapy is a feasible option with very promising outcomes for treating a number of inflammatory diseases including OA and RA.
Acknowledgments
Funding: None.
Footnote
Peer Review File: Available at https://atm.amegroups.com/article/view/10.21037/atm-23-1951/prf
Conflicts of Interest: All authors have completed the ICMJE uniform disclosure form (available at https://atm.amegroups.com/article/view/10.21037/atm-23-1951/coif). D.E. is an employee of GIOSTAR. R.M. and A.S. are employees of GIOSTAR and Cellebrations Life Sciences. The authors have no other conflicts of interest to declare.
Ethical Statement: The authors are accountable for all aspects of the work in ensuring that questions related to the accuracy or integrity of any part of the work are appropriately investigated and resolved.
Open Access Statement: This is an Open Access article distributed in accordance with the Creative Commons Attribution-NonCommercial-NoDerivs 4.0 International License (CC BY-NC-ND 4.0), which permits the non-commercial replication and distribution of the article with the strict proviso that no changes or edits are made and the original work is properly cited (including links to both the formal publication through the relevant DOI and the license). See: https://creativecommons.org/licenses/by-nc-nd/4.0/.
References
- Center for Disease Control and Prevention. National Statistics. 2023. Available online: https://www.cdc.gov/arthritis/basics/faqs.htm
- Hootman JM, Helmick CG, Barbour KE, et al. Updated Projected Prevalence of Self-Reported Doctor-Diagnosed Arthritis and Arthritis-Attributable Activity Limitation Among US Adults, 2015-2040. Arthritis Rheumatol 2016;68:1582-7. [Crossref] [PubMed]
- Mitra SP. 2013. Arthritis: classification, nature & cause - a review. Am. J. Bio-pharmacol. Biochem. Life Sciences 02 (03). Available online: https://www.researchgate.net/publication/281064966_ARTHRITIS_CLASSIFICATION_NATURE_CAUSE_-_A_REVIEW_Sankar_Mitra_PhD
- Arthritis Foundation. Arthritis By The Numbers, Book of Trusted Facts & Figures. 2019. Available online: https://www.arthritis.org/getmedia/e1256607-fa87-4593-aa8a-8db4f291072a/2019-abtn-final-march-2019.pdf
- Kloppenburg M, Berenbaum F. Osteoarthritis year in review 2019: epidemiology and therapy. Osteoarthritis Cartilage 2020;28:242-8. [Crossref] [PubMed]
- Hunter DJ, McDougall JJ, Keefe FJ. The symptoms of osteoarthritis and the genesis of pain. Med Clin North Am 2009;93:83-100. xi. [Crossref] [PubMed]
- Hunter DJ, Bierma-Zeinstra S. Osteoarthritis. Lancet 2019;393:1745-59. [Crossref] [PubMed]
- Kourilovitch M, Galarza-Maldonado C, Ortiz-Prado E. Diagnosis and classification of rheumatoid arthritis. J Autoimmun 2014;48-49:26-30. [Crossref] [PubMed]
- Aletaha D, Neogi T, Silman AJ, et al. 2010 Rheumatoid arthritis classification criteria: an American College of Rheumatology/European League Against Rheumatism collaborative initiative. Arthritis Rheum 2010;62:2569-81. [Crossref] [PubMed]
- He Y, Li Z, Alexander PG, et al. Pathogenesis of Osteoarthritis: Risk Factors, Regulatory Pathways in Chondrocytes, and Experimental Models. Biology (Basel) 2020;9:194. [Crossref] [PubMed]
- Scanzello CR, Goldring SR. The role of synovitis in osteoarthritis pathogenesis. Bone 2012;51:249-57. [Crossref] [PubMed]
- Mathiessen A, Conaghan PG. Synovitis in osteoarthritis: current understanding with therapeutic implications. Arthritis Res Ther 2017;19:18. [Crossref] [PubMed]
- Zhang H, Cai D, Bai X. Macrophages regulate the progression of osteoarthritis. Osteoarthritis Cartilage 2020;28:555-61. [Crossref] [PubMed]
- Zhu X, Lee CW, Xu H, et al. Phenotypic alteration of macrophages during osteoarthritis: a systematic review. Arthritis Res Ther 2021;23:110. [Crossref] [PubMed]
- Miller RE, Miller RJ, Malfait AM. Osteoarthritis joint pain: the cytokine connection. Cytokine 2014;70:185-93. [Crossref] [PubMed]
- Kisand K, Tamm AE, Lintrop M, et al. New insights into the natural course of knee osteoarthritis: early regulation of cytokines and growth factors, with emphasis on sex-dependent angiogenesis and tissue remodeling. A pilot study. Osteoarthritis Cartilage 2018;26:1045-54. [Crossref] [PubMed]
- Nguyen LT, Sharma AR, Chakraborty C, et al. Review of Prospects of Biological Fluid Biomarkers in Osteoarthritis. Int J Mol Sci 2017;18:601. [Crossref] [PubMed]
- Kapoor M, Martel-Pelletier J, Lajeunesse D, et al. Role of proinflammatory cytokines in the pathophysiology of osteoarthritis. Nat Rev Rheumatol 2011;7:33-42. [Crossref] [PubMed]
- Mengshol JA, Vincenti MP, Coon CI, et al. Interleukin-1 induction of collagenase 3 (matrix metalloproteinase 13) gene expression in chondrocytes requires p38, c-Jun N-terminal kinase, and nuclear factor kappaB: differential regulation of collagenase 1 and collagenase 3. Arthritis Rheum 2000;43:801-11. [Crossref] [PubMed]
- Afonso V, Champy R, Mitrovic D, et al. Reactive oxygen species and superoxide dismutases: role in joint diseases. Joint Bone Spine 2007;74:324-9. [Crossref] [PubMed]
- Scott JL, Gabrielides C, Davidson RK, et al. Superoxide dismutase downregulation in osteoarthritis progression and end-stage disease. Ann Rheum Dis 2010;69:1502-10. [Crossref] [PubMed]
- Usami Y, Gunawardena AT, Iwamoto M, et al. Wnt signaling in cartilage development and diseases: lessons from animal studies. Lab Invest 2016;96:186-96. [Crossref] [PubMed]
- Xi Y, Huang X, Tan G, et al. Protective effects of Erdosteine on interleukin-1β-stimulated inflammation via inhibiting the activation of MAPK, NF-κB, and Wnt/β-catenin signaling pathways in rat osteoarthritis. Eur J Pharmacol 2020;873:172925. [Crossref] [PubMed]
- Yuasa T, Otani T, Koike T, et al. Wnt/beta-catenin signaling stimulates matrix catabolic genes and activity in articular chondrocytes: its possible role in joint degeneration. Lab Invest 2008;88:264-74. [Crossref] [PubMed]
- Blom AB, Brockbank SM, van Lent PL, et al. Involvement of the Wnt signaling pathway in experimental and human osteoarthritis: prominent role of Wnt-induced signaling protein 1. Arthritis Rheum 2009;60:501-12. [Crossref] [PubMed]
- Oh H, Chun CH, Chun JS. Dkk-1 expression in chondrocytes inhibits experimental osteoarthritic cartilage destruction in mice. Arthritis Rheum 2012;64:2568-78. [Crossref] [PubMed]
- Snelling SJ, Davidson RK, Swingler TE, et al. Dickkopf-3 is upregulated in osteoarthritis and has a chondroprotective role. Osteoarthritis Cartilage 2016;24:883-91. [Crossref] [PubMed]
- McInnes IB, Schett G. Cytokines in the pathogenesis of rheumatoid arthritis. Nat Rev Immunol 2007;7:429-42. [Crossref] [PubMed]
- Xu B, Lin J. Characteristics and risk factors of rheumatoid arthritis in the United States: an NHANES analysis. PeerJ 2017;5:e4035. [Crossref] [PubMed]
- Krishna A. An Overall Review on Rheumatoid Arthritis. J Curr Pharma Res 2014;4:1138-43.
- Edwards SW, Hallett MB. Seeing the wood for the trees: the forgotten role of neutrophils in rheumatoid arthritis. Immunol Today 1997;18:320-4. [Crossref] [PubMed]
- Nigrovic PA, Lee DM. Mast cells in inflammatory arthritis. Arthritis Res Ther 2005;7:1-11. [Crossref] [PubMed]
- Yang X, Chang Y, Wei W. Emerging role of targeting macrophages in rheumatoid arthritis: Focus on polarization, metabolism and apoptosis. Cell Prolif 2020;53:e12854. [Crossref] [PubMed]
- Burrage PS, Mix KS, Brinckerhoff CE. Matrix metalloproteinases: role in arthritis. Front Biosci 2006;11:529-43. [Crossref] [PubMed]
- Hampel U, Sesselmann S, Iserovich P, et al. Chemokine and cytokine levels in osteoarthritis and rheumatoid arthritis synovial fluid. J Immunol Methods 2013;396:134-9. [Crossref] [PubMed]
- Lam J, Takeshita S, Barker JE, et al. TNF-alpha induces osteoclastogenesis by direct stimulation of macrophages exposed to permissive levels of RANK ligand. J Clin Invest 2000;106:1481-8. [Crossref] [PubMed]
- Osta B, Benedetti G, Miossec P. Classical and Paradoxical Effects of TNF-α on Bone Homeostasis. Front Immunol 2014;5:48. [Crossref] [PubMed]
- Bystrom J, Clanchy FI, Taher TE, et al. TNFα in the regulation of Treg and Th17 cells in rheumatoid arthritis and other autoimmune inflammatory diseases. Cytokine 2018;101:4-13. [Crossref] [PubMed]
- Noack M, Miossec P. Selected cytokine pathways in rheumatoid arthritis. Semin Immunopathol 2017;39:365-83. [Crossref] [PubMed]
- Chabaud M, Page G, Miossec P. Enhancing effect of IL-1, IL-17, and TNF-alpha on macrophage inflammatory protein-3alpha production in rheumatoid arthritis: regulation by soluble receptors and Th2 cytokines. J Immunol 2001;167:6015-20. [Crossref] [PubMed]
- Malemud CJ. Intracellular Signaling Pathways in Rheumatoid Arthritis. J Clin Cell Immunol 2013;4:160. [Crossref] [PubMed]
- Ingegnoli F, Castelli R, Gualtierotti R. Rheumatoid factors: clinical applications. Dis Markers 2013;35:727-34. [Crossref] [PubMed]
- Liu J, Gao J, Wu Z, et al. Anti-citrullinated Protein Antibody Generation, Pathogenesis, Clinical Application, and Prospects. Front Med (Lausanne) 2021;8:802934. [Crossref] [PubMed]
- Hermosillo-Villafranca JA, Guillén-Lozoya AH, Vega-Morales D, et al. Role of rheumatoid factor isotypes and anti-citrullinated peptide antibodies in the differential diagnosis of non-selected patients with inflammatory arthralgia. Reumatol Clin (Engl Ed) 2021;17:12-5. [Crossref] [PubMed]
- Fukui S, Iwamoto N, Takatani A, et al. M1 and M2 Monocytes in Rheumatoid Arthritis: A Contribution of Imbalance of M1/M2 Monocytes to Osteoclastogenesis. Front Immunol 2017;8:1958. [Crossref] [PubMed]
- Tardito S, Martinelli G, Soldano S, et al. Macrophage M1/M2 polarization and rheumatoid arthritis: A systematic review. Autoimmun Rev 2019;18:102397. [Crossref] [PubMed]
- Palmroth M, Kuuliala K, Peltomaa R, et al. Tofacitinib Suppresses Several JAK-STAT Pathways in Rheumatoid Arthritis In Vivo and Baseline Signaling Profile Associates With Treatment Response. Front Immunol 2021;12:738481. [Crossref] [PubMed]
- Friedman B, Cronstein B. Methotrexate mechanism in treatment of rheumatoid arthritis. Joint Bone Spine 2019;86:301-7. [Crossref] [PubMed]
- Wang M, Yuan Q, Xie L. Mesenchymal Stem Cell-Based Immunomodulation: Properties and Clinical Application. Stem Cells Int 2018;2018:3057624. [Crossref] [PubMed]
- Pittenger MF, Discher DE, Péault BM, et al. Mesenchymal stem cell perspective: cell biology to clinical progress. NPJ Regen Med 2019;4:22. [Crossref] [PubMed]
- Esquivel D, Mishra R, Soni P, et al. Stem Cells Therapy as a Possible Therapeutic Option in Treating COVID-19 Patients. Stem Cell Rev Rep 2021;17:144-52. [Crossref] [PubMed]
- Meirelles Lda S. Mechanisms involved in the therapeutic properties of mesenchymal stem cells. Cytokine Growth Factor Rev 2009;20:419-27. [Crossref] [PubMed]
- Madrigal M, Rao KS, Riordan NH. A review of therapeutic effects of mesenchymal stem cell secretions and induction of secretory modification by different culture methods. J Transl Med 2014;12:260. [Crossref] [PubMed]
- Li H, Shen S, Fu H, et al. Immunomodulatory Functions of Mesenchymal Stem Cells in Tissue Engineering. Stem Cells Int 2019;2019:9671206. [Crossref] [PubMed]
- Ellison-Hughes GM, Colley L, O'Brien KA, et al. The Role of MSC Therapy in Attenuating the Damaging Effects of the Cytokine Storm Induced by COVID-19 on the Heart and Cardiovascular System. Front Cardiovasc Med 2020;7:602183. [Crossref] [PubMed]
- Hass R, Kasper C, Böhm S, et al. Different populations and sources of human mesenchymal stem cells (MSC): A comparison of adult and neonatal tissue-derived MSC. Cell Commun Signal 2011;9:12. [Crossref] [PubMed]
- Marquez-Curtis LA, Janowska-Wieczorek A, McGann LE, et al. Mesenchymal stromal cells derived from various tissues: Biological, clinical and cryopreservation aspects. Cryobiology 2015;71:181-97. [Crossref] [PubMed]
- da Silva Meirelles L, Chagastelles PC, Nardi NB. Mesenchymal stem cells reside in virtually all post-natal organs and tissues. J Cell Sci 2006;119:2204-13. [Crossref] [PubMed]
- Harrell CR, Markovic BS, Fellabaum C, et al. Mesenchymal stem cell-based therapy of osteoarthritis: Current knowledge and future perspectives. Biomed Pharmacother 2019;109:2318-26. [Crossref] [PubMed]
- Sugimoto MA, Sousa LP, Pinho V, et al. Resolution of Inflammation: What Controls Its Onset? Front Immunol 2016;7:160. [Crossref] [PubMed]
- Leyendecker A Jr, Pinheiro CCG, Amano MT, et al. The Use of Human Mesenchymal Stem Cells as Therapeutic Agents for the in vivo Treatment of Immune-Related Diseases: A Systematic Review. Front Immunol 2018;9:2056. [Crossref] [PubMed]
- Luque-Campos N, Contreras-López RA, Jose Paredes-Martínez M, et al. Mesenchymal Stem Cells Improve Rheumatoid Arthritis Progression by Controlling Memory T Cell Response. Front Immunol 2019;10:798. [Crossref] [PubMed]
- Saldaña L, Bensiamar F, Vallés G, et al. Immunoregulatory potential of mesenchymal stem cells following activation by macrophage-derived soluble factors. Stem Cell Res Ther 2019;10:58. [Crossref] [PubMed]
- Mellor AL, Munn DH. IDO expression by dendritic cells: tolerance and tryptophan catabolism. Nat Rev Immunol 2004;4:762-74. [Crossref] [PubMed]
- Waiboci LW, Ahmed CM, Mujtaba MG, et al. Both the suppressor of cytokine signaling 1 (SOCS-1) kinase inhibitory region and SOCS-1 mimetic bind to JAK2 autophosphorylation site: implications for the development of a SOCS-1 antagonist. J Immunol 2007;178:5058-68. [Crossref] [PubMed]
- Galleu A, Riffo-Vasquez Y, Trento C, et al. Apoptosis in mesenchymal stromal cells induces in vivo recipient-mediated immunomodulation. Sci Transl Med 2017;9:eaam7828. [Crossref] [PubMed]
- Pang SHM, D'Rozario J, Mendonca S, et al. Mesenchymal stromal cell apoptosis is required for their therapeutic function. Nat Commun 2021;12:6495. [Crossref] [PubMed]
- ter Huurne M, Schelbergen R, Blattes R, et al. Antiinflammatory and chondroprotective effects of intraarticular injection of adipose-derived stem cells in experimental osteoarthritis. Arthritis Rheum 2012;64:3604-13. [Crossref] [PubMed]
- Ozeki N, Muneta T, Koga H, et al. Not single but periodic injections of synovial mesenchymal stem cells maintain viable cells in knees and inhibit osteoarthritis progression in rats. Osteoarthritis Cartilage 2016;24:1061-70. [Crossref] [PubMed]
- Kriston-Pál É, Haracska L, Cooper P, et al. A Regenerative Approach to Canine Osteoarthritis Using Allogeneic, Adipose-Derived Mesenchymal Stem Cells. Safety Results of a Long-Term Follow-Up. Front Vet Sci 2020;7:510. [Crossref] [PubMed]
- Shu J, Pan L, Huang X, et al. Transplantation of human amnion mesenchymal cells attenuates the disease development in rats with collagen-induced arthritis. Clin Exp Rheumatol 2015;33:484-90.
- Shin TH, Kim HS, Kang TW, et al. Human umbilical cord blood-stem cells direct macrophage polarization and block inflammasome activation to alleviate rheumatoid arthritis. Cell Death Dis 2016;7:e2524. [Crossref] [PubMed]
- Zhang Q, Li Q, Zhu J, et al. Comparison of therapeutic effects of different mesenchymal stem cells on rheumatoid arthritis in mice. PeerJ 2019;7:e7023. [Crossref] [PubMed]
- Seo MG, Park S, Han S, et al. Long-term treatment of allogeneic adipose-derived stem cells in a dog with rheumatoid arthritis. J Vet Sci 2022;23:e61. [Crossref] [PubMed]
- Davatchi F, Sadeghi Abdollahi B, Mohyeddin M, et al. Mesenchymal stem cell therapy for knee osteoarthritis: 5 years follow-up of three patients. Int J Rheum Dis 2016;19:219-25. [Crossref] [PubMed]
- Emadedin M, Aghdami N, Taghiyar L, et al. Intra-articular injection of autologous mesenchymal stem cells in six patients with knee osteoarthritis. Arch Iran Med 2012;15:422-8.
- Jo CH, Lee YG, Shin WH, et al. Intra-articular injection of mesenchymal stem cells for the treatment of osteoarthritis of the knee: a proof-of-concept clinical trial. Stem Cells 2014;32:1254-66. [Crossref] [PubMed]
- Mancuso P, Raman S, Glynn A, et al. Mesenchymal Stem Cell Therapy for Osteoarthritis: The Critical Role of the Cell Secretome. Front Bioeng Biotechnol 2019;7:9. [Crossref] [PubMed]
- Wang L, Wang L, Cong X, et al. Human umbilical cord mesenchymal stem cell therapy for patients with active rheumatoid arthritis: safety and efficacy. Stem Cells Dev 2013;22:3192-202. [Crossref] [PubMed]
- Yang Y, He X, Zhao R, et al. Serum IFN-γ levels predict the therapeutic effect of mesenchymal stem cell transplantation in active rheumatoid arthritis. J Transl Med 2018;16:165. [Crossref] [PubMed]
- Álvaro-Gracia JM, Jover JA, García-Vicuña R, et al. Intravenous administration of expanded allogeneic adipose-derived mesenchymal stem cells in refractory rheumatoid arthritis (Cx611): results of a multicentre, dose escalation, randomised, single-blind, placebo-controlled phase Ib/IIa clinical trial. Ann Rheum Dis 2017;76:196-202. [Crossref] [PubMed]
- Lopez-Santalla M, Fernandez-Perez R, Garin MI. Mesenchymal Stem/Stromal Cells for Rheumatoid Arthritis Treatment: An Update on Clinical Applications. Cells 2020;9:1852. [Crossref] [PubMed]