Association between blood methylmalonic acid and chronic kidney disease in the general US population: insights from multi-cycle National Health and Nutrition Examination Survey (NHANES)
Highlight box
Key findings
• Increased blood methylmalonic acid (MMA) levels were associated with more severe kidney impairment and increased risk of both chronic kidney disease (CKD) prevalence and mortality in participants with CKD.
What is known and what is new?
• Comprehending potential factors associated with the prevalence and outcomes of CKD is important and may be helpful in devising effective therapeutic approaches. MMA is involved in mitochondrial dysfunction (MD), which is a central factor in CKD.
• This study demonstrates that increased blood MMA levels were significantly associated with more severe kidney impairment and increased risk of both CKD prevalence and mortality in participants with CKD, utilizing the data from the National Health and Nutrition Examination Survey.
What is the implication, and what should change now?
• This study indicates that blood MMA, serving as a surrogate biomarker of MD, is independently and robustly associated with increased CKD prevalence and mortality in participants with CKD.
• The current study provides important clinical value for disease prevention and prediction of disease prognosis.
Introduction
Chronic kidney disease (CKD) is a widespread public health concern worldwide, resulting in end-stage kidney disease (ESKD). Based on the 2021 report by the Centers for Disease Control and Prevention (CDC), CKD affects over 1 in 7 American adults, with approximately 37 million individuals (1). Comprehending potential factors associated with CKD prevalence and outcome is important and might be helpful for devising efficacious therapeutic approaches.
Mitochondrial dysfunction (MD) is a central factor in CKD (2-5). The intermediate metabolite methylmalonic acid (MMA), produced through fatty acids and amino acids breakdown, enters the Krebs cycle when converted into succinic acid in normal circumstances (6). MMA was revealed to be involved in MD and oxidative stress (OS). An abnormal MMA accumulation occurs due to a deficiency of coenzyme active vitamin B12 (VitB12) or deactivation of mitochondrial methyl malonyl-CoA mutase (MUT) (7). Excessive accumulation of MMA could disrupt the electron transport chain, further impairing mitochondrial energy metabolism and producing reactive oxygen species (ROS) (8,9). Several studies found that the blood MMA level could be a useful marker for predicting poor outcomes of chronic diseases, including diabetes and cancer, associated with MD or OS (10,11). Moreover, the level of blood MMA was found to be an independent factor associated with all-cause mortality in the general population. This association was more prominent among patients with impaired kidney function (12,13). However, few investigations have concentrated on the associations between blood MMA levels and kidney impairment, the prevalence of CKD, as well as outcomes in CKD patients.
Herein, we explored the associations between blood MMA levels and kidney impairment, the risk of CKD prevalence, as well as all-cause mortality in CKD patients, utilizing the data from the National Health and Nutrition Examination Survey (NHANES), a comprehensive and long-term epidemiological survey conducted in the United States. We present this article in accordance with the STROBE reporting checklist (available at https://atm.amegroups.com/article/view/10.21037/atm-23-1930/rc).
Methods
Study design and participants
The NHANES study is a comprehensive investigation of the health condition of non-institutionalized civilians across all age groups, employing a national, stratified, and multistage probability methodology. This study was conducted in accordance with the Declaration of Helsinki (as revised in 2013). All data were downloaded at https://www.cdc.gov/nchs/nhanes/. The present investigation incorporated 51,057 subjects who participated in five 2-year NHANES cycles (1999–2000, 2001–2002, 2003–2004, 2011–2012, and 2013–2014). We excluded 22,019 participants below the age of 18 years, 3,907 participants who had incomplete blood MMA data, 231 individuals with missing estimated glomerular filtration rate (eGFR), 394 participants without urinary albumin-to-creatinine ratio (uACR), 889 individuals who were pregnant, and 30 participants who lacked follow-up for mortality status. The study eventually enrolled a total of 23,587 participants. For primary analyses, a subset comprising the initial three cycles of NHANES 1999–2004 was utilized (n=13,530). The remaining two cycles (n=10,057) were used for validation. Figure 1 illustrates the specifics of the recruitment process.
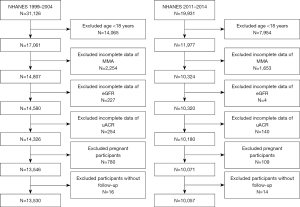
Data collection and definition
All data were collected from demographic surveys, physical examinations, and laboratory data in NHANES. Self-administered questionnaires were utilized for obtaining general data including age, gender, race/ethnicity, and smoking status. The categorization of smoking status was never, ever, and current. Individuals who have smoked a minimum of 100 cigarettes in their whole life but have since ceased smoking were classified as “ever smokers”.
Physical examinations were performed in mobile examination centers (MEC). The study categorized the participants based on their body mass index (BMI) categories into normal weight (BMI <25 kg/m2) and overweight/obesity (BMI ≥25 kg/m2). Average systolic blood pressure (SBP) or diastolic blood pressure (DBP) was calculated as the mean of eligible three readings. Hypertension was characterized by SBP ≥140 mmHg and/or DBP ≥90 mmHg, as determined through multiple examinations or a documented history of hypertension (14).
The laboratory measurements, including uACR, blood MMA level, serum creatinine (Scr), blood urea nitrogen (BUN), serum uric acid (UA), serum VitB12, triglyceride (TG), total cholesterol (TC), high-density lipoprotein-cholesterol (HDL-C), low-density lipoprotein-cholesterol (LDL-C), fasting blood glucose (FBG), and hemoglobin A1c (HbA1c) were conducted across all cycles. However, the measurements of C-reactive protein (CRP) and homocysteine were only available for the NHANES 1999–2004 cycle. The Chronic Kidney Disease Epidemiology Collaboration (CKD-EPI) was utilized for the eGFR calculation (15). In accordance with NHANES reports, cardiovascular disease (CVD) was characterized as a self-reported medical history of coronary heart disease, heart failure, or stroke. The definition of diabetes in this study was based on self-reported diabetes history and/or FBG ≥7.0 mmol/L and/or self-reported utilization of anti-diabetic drugs (16). The diagnostic criteria for hyperlipidemia were established as follows: TC ≥240 mg/dL, TG ≥200 mg/dL, LDL-C ≥160 mg/dL, or HDL-C <40 mg/dL (17). CKD was characterized by either albuminuria, lower eGFR, or both (18). The criterion for albuminuria was established as uACR ≥30 mg/g while defining reduced eGFR as an eGFR ≤60 mL/min/1.73 m2.
The participants with an age of 18 years or above in NHANES were associated with the National Death Index (NDI) through a distinctive sequence number until December 31, 2015 (19). Our study outcome was all-cause mortality.
Blood MMA measurement
Measuring blood MMA levels were performed in either plasma or serum, with a preference for plasma, in NHANES. The previous study validated that the reference range for MMA concentrations in both serum and plasma is consistent and that the coefficient of variation is comparable (20). The level of blood MMA was subjected to analysis through gas chromatograph/mass spectrometer (GC/MS, NHANES 1999–2004) or isotope-dilution high-performance liquid chromatography coupled to tandem mass spectrometry (LC-MS/MS, NHANES 2011–2014). The standard protocols for these methods are accessible on the NHANES website and were followed accordingly. Both methodologies exhibited a strong correlation and were devoid of any bias (21).
Statistical analyses
The data were reported as mean ± standard deviation (SD), or median and interquartile range (IQR) for continuous data, while as absolute frequencies and percentages for categorical data. Normalized expression data were log2-transformed. The comparisons of clinical features between the two groups were conducted through a weighted student’s t-test for continuous data and a weighted chi-square test for categorical data. Weighted linear regression analysis with unstandardized regression coefficients (β) as effect measure was utilized for the correlation analysis between blood MMA levels and other clinical parameters, in which blood MMA levels acted as an independent variable, and eGFR, uACR, folate, serum VitB12, homocysteine, HDL-C, LDL-C, TG, TC, as well as CRP acted as dependent variables, respectively. The association between blood MMA levels and CKD prevalence was evaluated through the weighted logistic regression model. Specifically, model 1 followed an adjustment for age, gender, and race/ethnicity, model 2 followed further adjustment for BMI, smoking status, hypertension, diabetes mellitus (DM) history, and CVD history, and model 3 was further adjusted for eGFR, uACR, HbA1c, FBG, folate, serum VitB12, hyperlipidemia, and homocysteine. Results were reported as odds ratio (OR) with their 95% confidence interval (CI). Furthermore, stratification analyses were also applied for the association between blood MMA levels and CKD prevalence in subgroups by age (<45 and ≥45 years) (22), gender (female and male), race/ethnicity (Mexican American, other Hispanic, non-Hispanic White, non-Hispanic Black, other race), DM (yes or no), homocysteine (<10 and ≥10 µmol/L), and serum VitB12 (<400 and ≥400 pmol/L). Interaction analyses were incorporated to examine the heterogeneity of relations among various subgroups.
The study employed the Kaplan-Meier (K-M) analysis to estimate the survival of patients with CKD. The stratified log-rank test was utilized to evaluate any potential difference in survival rates. The current study employed weighted Cox proportional hazards model for estimating the association of blood MMA levels with all-cause mortality. Model 0 only comprised blood MMA levels, model 1 incorporated demographic variables (age, gender, race/ethnicity), and model 2 comprised variables from model 3 plus physical examination and medical history data (BMI, smoking status, hypertension, diabetes history, and CVD history). Finally, in model 4, laboratory variables were added. The relation robustness between MMA and mortality was additionally verified in NHANES 2011–2014, with the exception of homocysteine, by adjusting for the aforementioned potential confounders. Results were presented in terms of hazard ratio (HR) with 95% CI. Note that analyses were based on log2 transformed blood MMA value, the untransformed value was also used for data analyses. The statistical software package R (version 4.3.0) was employed for conducting the statistical analyses. P<0.05 indicated a significant difference.
Results
The level of blood MMA between participants with or without CKD
Of all participants enrolled in NHANES 1999–2004 (n=13,530), 1,761 (13.02%) were classified as CKD and 11,769 (86.98%) as non-CKD. A comparison of general characteristics between CKD and non-CKD participants is shown in Table 1. Participants with CKD were significantly older than those without CKD (64.45±17.42 vs. 43.43±15.95 years, P<0.001). Participants with CKD also showed significantly higher levels of SBP, folate, homocysteine, HbA1c, and CRP than those without CKD. Compared with participants without CKD, the prevalences of CVD, hypertension, hyperlipidemia, and diabetes were significantly higher in those with CKD. Moreover, blood MMA levels were significantly higher in participants with CKD than those without CKD (7.60±0.86 vs. 7.03±0.62, P<0.001). Even after adjusting for eGFR and serum VitB12, the level of blood MMA in participants with CKD was still significantly higher than those without CKD [mean (95% CI): 7.29 (7.25–7.33) vs. 7.04 (7.03–7.05), P<0.001]. These results were validated in the NHANES 2011–2014 validation cohort (Table S1).
Table 1
Variables | CKD group (n=1,761) | Non-CKD group (n=11,769) | P value |
---|---|---|---|
Age (years) | 64.45±17.42 | 43.43±15.95 | <0.001 |
Gender (male/female) | 43.38/56.62 | 49.87/50.13 | <0.001 |
Race | 0.004 | ||
Mexican American | 5.00 | 7.57 | |
Other Hispanic | 5.17 | 5.98 | |
Non-Hispanic White | 72.87 | 71.73 | |
Non-Hispanic Black | 11.59 | 10.15 | |
Other race | 5.37 | 4.58 | |
BMI (<25/≥25 kg/m2) | 27.86/72.14 | 36.12/63.88 | <0.001 |
Smoke (never/ever/current) | 47.50/37.12/15.39 | 50.18/24.07/25.75 | <0.001 |
SBP (mmHg) | 137.97±25.01 | 121.18±17.26 | <0.001 |
DBP (mmHg) | 68.86±17.61 | 72.12±11.92 | <0.001 |
Hypertension (yes/no) | 73.60/26.40 | 31.06/68.94 | <0.001 |
Diabetes (yes/no) | 30.85/69.15 | 6.90/93.10 | <0.001 |
CVD (yes/no) | 30.95/69.05 | 6.31/93.69 | <0.001 |
Hyperlipidemia (yes/no) | 56.75/43.25 | 50.35/49.65 | <0.001 |
FBG (mmol/L) | 5.80 (5.24, 7.02) | 5.28 (4.93, 5.69) | <0.001 |
HbA1c (%) | 6.10±1.53 | 5.40±0.78 | <0.001 |
eGFR (mL/min/1.73 m2) | 66.71±27.88 | 101.30±19.28 | <0.001 |
Scr (μmol/L) | 97.24 (79.56, 114.92) | 70.72 (61.88, 79.60) | <0.001 |
uACR (mg/g) | 31.86 (4.53, 75.13) | 2.82 (1.92, 4.86) | <0.001 |
BUN (mmol/L) | 6.10 (4.64, 8.20) | 4.30 (3.57, 5.40) | <0.001 |
Folate (nmol/L) | 32.40 (22.00, 50.50) | 27.40 (19.70, 38.50) | <0.001 |
VitB12 (pmol/L) | 351.29 (256.82, 466.42) | 336.53 (258.30, 440.59) | 0.06 |
Homocysteine (μmol/L) | 10.85 (8.49, 13.79) | 7.80 (6.51, 9.38) | <0.001 |
CRP (mg/dL) | 0.29 (0.13, 0.65) | 0.19 (0.07, 0.42) | <0.001 |
TC (mmol/L) | 5.22 (4.47, 5.97) | 5.09 (4.47, 5.84) | 0.004 |
TG (mmol/L) | 1.64 (1.20, 2.45) | 1.29 (0.89, 1.89) | <0.001 |
HDL-C (mmol/L) | 1.24 (1.03, 1.53) | 1.28 (1.06, 1.58) | 0.16 |
LDL-C (mmol/L) | 3.03 (2.48, 3.62) | 3.05 (2.46, 3.67) | 0.23 |
Blood MMA (log2) | 7.60±0.86 | 7.03±0.62 | <0.001 |
Data are presented as mean ± standard deviation, median (interquartile range), or percentage. CKD, chronic kidney disease; BMI, body mass index; SBP, systolic blood pressure; DBP, diastolic blood pressure; CVD, cardiovascular disease; FBG, fasting blood glucose; HbA1c, hemoglobin A1c; eGFR, estimated glomerular filtration rate; Scr, serum creatinine; uACR, urine albumin-to-creatinine ratio; BUN, blood urea nitrogen; VitB12, vitamin B12; CRP, C-reactive protein; TC, total cholesterol; TG, total triglyceride; HDL-C, high-density lipoprotein-cholesterol; LDL-C, low-density lipoprotein-cholesterol; MMA, methylmalonic acid.
Associations of blood MMA levels with clinical parameters
Next, the associations between the blood MMA levels and clinical parameters in patients with CKD were evaluated using weighted linear regression. In NHANES 1999–2004, it was found that blood MMA had a significant positive association with uACR (β=45.29, P=0.01) and homocysteine (β=4.23, P<0.001). Conversely, blood MMA had a significant negative association with eGFR (β=−15.27, P<0.001) and serum VitB12 (β=−78.92, P=0.003). Nevertheless, no significant association was detected between blood MMA level and HDL-C, LDL-C, TG, TC, FBG, HbA1c, or CRP. Furthermore, the significant association between MMA and kidney impairment was further validated in NHANES 2011–2014. We found that blood MMA level was positively correlated with uACR (β=89.96, P<0.001) and inversely correlated with eGFR (β=−20.06, P<0.001) in the validation cohort.
Association between the level of blood MMA and CKD prevalence
With the use of weighted logistic regression, higher blood MMA levels were significantly associated with a higher CKD risk (OR: 2.91, 95% CI: 2.67–3.16, P<0.001). The results maintained statistical significance following the adjustment for age, gender, and race/ethnicity (OR: 2.04, 95% CI: 1.85–2.25, P<0.001). The association also maintained statistical significance following the adjustment for potential risk factors, including age, gender, race/ethnicity, BMI, smoking status, hypertension, diabetes history, CVD history, eGFR, uACR, HbA1c, FBG, folate, serum VitB12, hyperlipidemia and homocysteine (OR: 1.32, 95% CI: 1.05–1.64, P=0.01, Table 2). The aforementioned findings were verified in the validation cohort of NHANES 2011–2014 (Table S2).
Table 2
Variables | OR | 95% Cl | P value |
---|---|---|---|
M0 | 2.91 | 2.67–3.16 | <0.001 |
M1 | 2.04 | 1.85–2.25 | <0.001 |
M2 | 1.95 | 1.78–2.13 | <0.001 |
M3 | 1.32 | 1.05–1.64 | 0.01 |
M0 (n=13,530): blood MMA; M1 (n=13,530): additionally adjusted for age, gender and race/ethnicity; M2 (n=11,541): additionally adjusted for smoke status, BMI, hypertension, diabetes, CVD; M3 (n=5,661): additionally adjusted for eGFR, uACR, HbA1c, FBG, folate, serum VitB12, hyperlipidemia, homocysteine. CKD, chronic kidney disease; MMA, methylmalonic acid; NHANES, National Health and Nutrition Examination Survey; OR, odds ratio; CI, confidence interval; BMI, body mass index; CVD, cardiovascular disease; eGFR, estimated glomerular filtration rate; uACR, urine albumin-to-creatinine ratio; HbA1c, hemoglobin A1c; FBG, fasting blood glucose; VitB12, vitamin B12.
The results of the stratified and interaction analyses of the association between blood MMA level and CKD prevalence are presented in Figure 2. For the subgroup stratified by gender, diabetes, homocysteine and serum VitB12, blood MMA levels were found to be significantly associated with the prevalence of CKD in each subgroup (P<0.05). The stratified analyses demonstrated that blood MMA level was significantly associated with CKD in participants older than 45 years (adjusted OR: 1.83, 95% CI: 1.55–2.17, P<0.001). In Mexican American, non-Hispanic White and non-Hispanic Black, blood MMA levels were associated with a greater risk of CKD prevalence (adjusted OR: 1.81, 95% CI: 1.16–2.81, P=0.009; adjusted OR: 1.67, 95% CI: 1.36–2.05, P<0.001; adjusted OR: 2.21, 95% CI: 1.22–4.01, P=0.009, respectively). The interaction analyses revealed no interactive role in the association between the level of blood MMA and CKD prevalence.
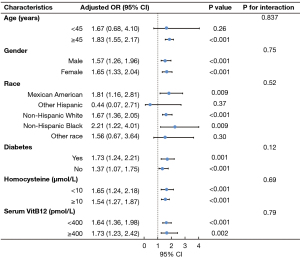
Association of blood level of MMA with all-cause mortality in CKD patients
To investigate the association between blood level of MMA and all-cause mortality in patients with CKD, we gathered data on NHANES participants with CKD and linked them to NDI for follow-up of their survival. During the 1,761 person-years follow-up (median: 12.7 years), 1,219 patients died (all-cause mortality). We found that a higher level of blood MMA at baseline exhibited a significant association with an elevated risk of all-cause mortality during the follow-up (Figure 3A). After adjustment for all relevant confounders, a higher level of blood MMA was significantly associated with an increased risk of all-cause mortality (log-rank test, P<0.001). Weighted univariable regression analysis also identified the increased blood MMA level as a predictor for all-cause mortality in patients with CKD (HR: 1.54, 95% CI: 1.44–1.64, P<0.001). Furthermore, multivariable Cox regression analysis indicated that the level of blood MMA remained an independent predictor for all-cause mortality, even after the adjustment for age, gender, race/ethnicity, BMI, smoking status, hypertension, diabetes history, CVD history, eGFR, uACR, HbA1c, FBG, hyperlipidemia, folate, serum VitB12 and homocysteine (HR: 1.26, 95% CI: 1.11–1.43, P<0.001; Table 3).
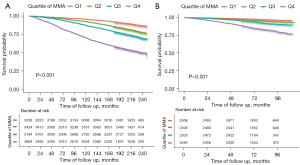
Table 3
Variables | HR | 95% Cl | P value |
---|---|---|---|
M0 | 1.54 | 1.44–1.64 | <0.001 |
M1 | 1.31 | 1.21–1.41 | <0.001 |
M2 | 1.29 | 1.21–1.38 | <0.001 |
M3 | 1.26 | 1.11–1.43 | <0.001 |
M0 (n=1,761): blood MMA; M1 (n=1,761): additionally adjusted for age, gender and race/ethnicity; M2 (n=1,576): additionally adjusted for smoke status, BMI, hypertension, diabetes and CVD; M3 (n=764): additionally adjusted for eGFR, uACR, HbA1c, FBG, folate, serum VitB12, hyperlipidemia and homocysteine. CKD, chronic kidney disease; NHANES, National Health and Nutrition Examination Survey; HR, hazard ratio; CI, confidence interval; MMA, methylmalonic acid; BMI, body mass index; CVD, cardiovascular disease; eGFR, estimated glomerular filtration rate; uACR, urine albumin-to-creatinine ratio; HbA1c, hemoglobin A1c; FBG, fasting blood glucose; VitB12, vitamin B12.
The association between the level of blood MMA and all-cause mortality in CKD patients was verified in 1,793 participants of NHANES 2011–2014. Throughout the median 6.4-year follow-up, 460 patients died. Similarly, the K-M curve and multivariable Cox regression analyses suggested a significant association between blood MMA levels and the risk of all-cause mortality in the validation cohort (HR: 1.35, 95% CI: 1.14–1.60, P<0.001, shown in Figure 3B and Table S3).
Discussion
Elevated circulating MMA levels have been reported in several diseases in adults, such as coronary heart disease, kidney dysfunction, and diabetes (23-25). Moreover, emerging evidence suggested that elevated level of blood MMA may serve as a promising biomarker for predicting adverse outcomes in chronic diseases (12,13,26). As we know, MD is regarded as a contributing factor to CKD progression (27). However, whether the level of blood MMA is associated with the risk of CKD and mortality of CKD participants is of interest for investigation.
Herein, a significant elevation was detected in the level of blood MMA among the participants with CKD compared with those without CKD. Meanwhile, we observed that the blood MMA levels were significantly correlated with kidney impairment in patients with CKD. MMA is one of the biomarkers involved in VitB12 metabolism, alongside folate and homocysteine (28). It was reported significant differences in serum VitB12, folate, and homocysteine levels between CKD and non-CKD populations (29), which was consistent with our findings. However, comparing with serum VitB12, folate and homocysteine, blood level of MMA had a more sensitive and specific performance in reflecting VitB12 homeostasis (30). The disruption of VitB12 homeostasis directly leads to adverse effects of cardiovascular risk and CKD progression (31,32). In addition, the level of blood MMA was found to have a significant association with all-cause mortality in the general population (12). In the present study, we extended previous findings and, for the first time, found that higher MMA levels exhibited a significant association with an elevated risk of CKD prevalence and all-cause mortality in patients with CKD. The associations between blood MMA and the prevalence of CKD patients in the stratified analyses were consistent with that in the multivariable logistic regression analysis.
Although blood level of MMA is primarily recognized as a functional marker of VitB12 deficiency and is influenced by renal function (24), there is increasing evidence suggesting that only a portion of the variation in MMA levels could be explained by VitB12 and eGFR (13,33,34). Consistent with prior studies, our findings demonstrated that even after adjusting for eGFR and VitB12, patients with CKD still exhibited significantly higher blood MMA level compared with the non-CKD group. Increasing evidence has supported that MMA is not only a metabolite indicating VitB12 deficiency but also a marker for MD and OS (35,36). A recent study demonstrated that proximal tubular MD is a key pathogenic mechanism behind MMA-related kidney disorders, and antioxidants can attenuate the severity of nephropathy (37). However, the toxicity of MMA in MD was revealed to be mainly based on congenital methylmalonic acidemia (8,38). Mitochondrial damage could exacerbate kidney damage, leading to a vicious cycle in CKD progression (39,40). We assumed that MMA may be involved in the pathogenesis of CKD.
This study has several strengths. Regarding current knowledge, this study presents the initial investigation documenting the associations between circulating MMA and CKD prevalence. Using a nationally representative sample improves the extent to which our findings can be generalized. Nonetheless, there were no supplementary data beyond mortality for follow-up purposes in NHANES analysis. Subsequent investigations should document repeated measurements of eGFR and questionnaires to authenticate the reported associations. There are some limitations to our study. The current study is observational, and additional research is required to ascertain whether the relationship between MMA and kidney progression in patients with CKD is causal.
Conclusions
Blood MMA level was positively associated with CKD prevalence. Moreover, blood MMA level was an independent prognostic factor in the mortality of patients with CKD.
Acknowledgments
The authors would like to acknowledge the patients and staff of the NHANES.
Funding: This investigation was supported by grants from the National Key Research and Development Program (Nos. 2022YFC2502500 and 2022YFC2502502), National Natural Science Fund (Nos. 82200794, 82270754, and 82090020), CAMS Innovation Fund for Medical Sciences (No. 2019-I2M-5-046), and National High-Level Hospital Clinical Research Funding (Nos. 2022SF01 and 2022XW01).
Footnote
Reporting Checklist: The authors have completed the STROBE reporting checklist. Available at https://atm.amegroups.com/article/view/10.21037/atm-23-1930/rc
Peer Review File: Available at https://atm.amegroups.com/article/view/10.21037/atm-23-1930/prf
Conflicts of Interest: All authors have completed the ICMJE uniform disclosure form (available at https://atm.amegroups.com/article/view/10.21037/atm-23-1930/coif). The authors have no conflicts of interest to declare.
Ethical Statement: The authors are accountable for all aspects of the work in ensuring that questions related to the accuracy or integrity of any part of the work are appropriately investigated and resolved. This study was conducted in accordance with the Declaration of Helsinki (as revised in 2013).
Open Access Statement: This is an Open Access article distributed in accordance with the Creative Commons Attribution-NonCommercial-NoDerivs 4.0 International License (CC BY-NC-ND 4.0), which permits the non-commercial replication and distribution of the article with the strict proviso that no changes or edits are made and the original work is properly cited (including links to both the formal publication through the relevant DOI and the license). See: https://creativecommons.org/licenses/by-nc-nd/4.0/.
References
- Centers for Disease Control and Prevention. Chronic Kidney Disease Surveillance System. Available online: https://nccd.cdc.gov/CKD. (Accessed 2/19/2021).
- Hallan S, Afkarian M, Zelnick LR, et al. Metabolomics and Gene Expression Analysis Reveal Down-regulation of the Citric Acid (TCA) Cycle in Non-diabetic CKD Patients. EBioMedicine 2017;26:68-77. [Crossref] [PubMed]
- Kang HM, Ahn SH, Choi P, et al. Defective fatty acid oxidation in renal tubular epithelial cells has a key role in kidney fibrosis development. Nat Med 2015;21:37-46. [Crossref] [PubMed]
- Galvan DL, Green NH, Danesh FR. The hallmarks of mitochondrial dysfunction in chronic kidney disease. Kidney Int 2017;92:1051-7. [Crossref] [PubMed]
- Wu L, Liu C, Chang DY, et al. The Attenuation of Diabetic Nephropathy by Annexin A1 via Regulation of Lipid Metabolism Through the AMPK/PPARα/CPT1b Pathway. Diabetes 2021;70:2192-203. [Crossref] [PubMed]
- Green R, Allen LH, Bjørke-Monsen AL, et al. Vitamin B(12) deficiency. Nat Rev Dis Primers 2017;3:17040. [Crossref] [PubMed]
- Chandler RJ, Venditti CP. Genetic and genomic systems to study methylmalonic acidemia. Mol Genet Metab 2005;86:34-43. [Crossref] [PubMed]
- Stepien KM, Heaton R, Rankin S, et al. Evidence of Oxidative Stress and Secondary Mitochondrial Dysfunction in Metabolic and Non-Metabolic Disorders. J Clin Med 2017;6:71. [Crossref] [PubMed]
- Luciani A, Schumann A, Berquez M, et al. Impaired mitophagy links mitochondrial disease to epithelial stress in methylmalonyl-CoA mutase deficiency. Nat Commun 2020;11:970. [Crossref] [PubMed]
- Obeid R, Jung J, Falk J, et al. Serum vitamin B12 not reflecting vitamin B12 status in patients with type 2 diabetes. Biochimie 2013;95:1056-61. [Crossref] [PubMed]
- Gomes AP, Ilter D, Low V, et al. Age-induced accumulation of methylmalonic acid promotes tumour progression. Nature 2020;585:283-7. [Crossref] [PubMed]
- Wang S, Liu Y, Liu J, et al. Mitochondria-derived methylmalonic acid, a surrogate biomarker of mitochondrial dysfunction and oxidative stress, predicts all-cause and cardiovascular mortality in the general population. Redox Biol 2020;37:101741. [Crossref] [PubMed]
- Riphagen IJ, Minović I, Groothof D, et al. Methylmalonic acid, vitamin B12, renal function, and risk of all-cause mortality in the general population: results from the prospective Lifelines-MINUTHE study. BMC Med 2020;18:380. [Crossref] [PubMed]
- Unger T, Borghi C, Charchar F, et al. 2020 International Society of Hypertension Global Hypertension Practice Guidelines. Hypertension 2020;75:1334-57. [Crossref] [PubMed]
- Levey AS, Stevens LA, Schmid CH, et al. A new equation to estimate glomerular filtration rate. Ann Intern Med 2009;150:604-12. [Crossref] [PubMed]
- Irazola V, Rubinstein A, Bazzano L, et al. Prevalence, awareness, treatment and control of diabetes and impaired fasting glucose in the Southern Cone of Latin America. PLoS One 2017;12:e0183953. [Crossref] [PubMed]
- Yancy WS Jr, Olsen MK, Guyton JR, et al. A low-carbohydrate, ketogenic diet versus a low-fat diet to treat obesity and hyperlipidemia: a randomized, controlled trial. Ann Intern Med 2004;140:769-77. [Crossref] [PubMed]
- Afkarian M, Katz R, Bansal N, et al. Diabetes, Kidney Disease, and Cardiovascular Outcomes in the Jackson Heart Study. Clin J Am Soc Nephrol 2016;11:1384-91. [Crossref] [PubMed]
- Wang S, Tian W, Liu Y, et al. Temporal trend of circulating trans-fatty acids and risk of long-term mortality in general population. Clin Nutr 2021;40:1095-101. [Crossref] [PubMed]
- Erdogan E, Nelson GJ, Rockwood AL, et al. Evaluation of reference intervals for methylmalonic acid in plasma/serum and urine. Clin Chim Acta 2010;411:1827-9. [Crossref] [PubMed]
- Mineva EM, Zhang M, Rabinowitz DJ, et al. An LC-MS/MS method for serum methylmalonic acid suitable for monitoring vitamin B12 status in population surveys. Anal Bioanal Chem 2015;407:2955-64. [Crossref] [PubMed]
- Qian D, Wang ZF, Cheng YC, et al. Early Menopause May Associate With a Higher Risk of CKD and All-Cause Mortality in Postmenopausal Women: An Analysis of NHANES, 1999-2014. Front Med (Lausanne) 2022;9:823835. [Crossref] [PubMed]
- Polytarchou K, Dimitroglou Y, Varvarousis D, et al. Methylmalonic acid and vitamin B12 in patients with heart failure. Hellenic J Cardiol 2020;61:330-7. [Crossref] [PubMed]
- van Loon SL, Wilbik AM, Kaymak U, et al. Improved testing for vitamin B(12) deficiency: correcting MMA for eGFR reduces the number of patients classified as vitamin B(12) deficient. Ann Clin Biochem 2018;55:685-92. [Crossref] [PubMed]
- Wang J, Tang Y, Liu Y, et al. Correlations between circulating methylmalonic acid levels and all-cause and cause-specific mortality among patients with diabetes. Front Nutr 2022;9:974938. [Crossref] [PubMed]
- Wang S, Wang Y, Wan X, et al. Cobalamin Intake and Related Biomarkers: Examining Associations With Mortality Risk Among Adults With Type 2 Diabetes in NHANES. Diabetes Care 2022;45:276-84. [Crossref] [PubMed]
- He L, Wei Q, Liu J, et al. AKI on CKD: heightened injury, suppressed repair, and the underlying mechanisms. Kidney Int 2017;92:1071-83. [Crossref] [PubMed]
- Klee GG. Cobalamin and folate evaluation: measurement of methylmalonic acid and homocysteine vs vitamin B(12) and folate. Clin Chem 2000;46:1277-83. [Crossref] [PubMed]
- Capelli I, Cianciolo G, Gasperoni L, et al. Folic Acid and Vitamin B12 Administration in CKD, Why Not? Nutrients 2019;11:383. [Crossref] [PubMed]
- Kopp M, Morisset R, Rychlik M. Characterization and Interrelations of One-Carbon Metabolites in Tissues, Erythrocytes, and Plasma in Mice with Dietary Induced Folate Deficiency. Nutrients 2017;9:462. [Crossref] [PubMed]
- Strain JJ, Dowey L, Ward M, et al. B-vitamins, homocysteine metabolism and CVD. Proc Nutr Soc 2004;63:597-603. [Crossref] [PubMed]
- Verhoef P, Stampfer MJ, Buring JE, et al. Homocysteine metabolism and risk of myocardial infarction: relation with vitamins B6, B12, and folate. Am J Epidemiol 1996;143:845-59. [Crossref] [PubMed]
- Vogiatzoglou A, Oulhaj A, Smith AD, et al. Determinants of plasma methylmalonic acid in a large population: implications for assessment of vitamin B12 status. Clin Chem 2009;55:2198-206. [Crossref] [PubMed]
- Mineva EM, Sternberg MR, Zhang M, et al. Age-specific reference ranges are needed to interpret serum methylmalonic acid concentrations in the US population. Am J Clin Nutr 2019;110:158-68. [Crossref] [PubMed]
- Chandler RJ, Zerfas PM, Shanske S, et al. Mitochondrial dysfunction in mut methylmalonic acidemia. FASEB J 2009;23:1252-61. [Crossref] [PubMed]
- Mirandola SR, Melo DR, Schuck PF, et al. Methylmalonate inhibits succinate-supported oxygen consumption by interfering with mitochondrial succinate uptake. J Inherit Metab Dis 2008;31:44-54. [Crossref] [PubMed]
- Manoli I, Sysol JR, Li L, et al. Targeting proximal tubule mitochondrial dysfunction attenuates the renal disease of methylmalonic acidemia. Proc Natl Acad Sci U S A 2013;110:13552-7. [Crossref] [PubMed]
- Kölker S, Okun JG. Methylmalonic acid--an endogenous toxin? Cell Mol Life Sci 2005;62:621-4. [Crossref] [PubMed]
- Duann P, Lin PH. Mitochondria Damage and Kidney Disease. Adv Exp Med Biol 2017;982:529-51. [Crossref] [PubMed]
- Takemura K, Nishi H, Inagi R. Mitochondrial Dysfunction in Kidney Disease and Uremic Sarcopenia. Front Physiol 2020;11:565023. [Crossref] [PubMed]