Eliminating residual neuromuscular blockade: a literature review
Introduction
Approximately 21 million people receive a general anesthetic each year. As part of that anesthetic, many of these individuals receive succinylcholine and/or a nondepolarizing neuromuscular blocking agent (NMBA). NMBAs are used most commonly to facilitate endotracheal intubation and optimize surgical conditions. Although they are frequently incorporated into a general anesthetic, their use is associated with increased surgical morbidity and mortality—at least some of which is due to the physiologic effects of residual neuromuscular blockade (NMB). Depth of neuromuscular blockade is defined by the muscular response to stimulation of a motor nerve, commonly the ulnar nerve. Contraction of the adductor pollicis when four responses occur after train-of-four (TOF) stimulation, is quantified by the train-of-four ratio (TOFR), the strength of the fourth response relative to the first response. When there are fewer than four responses to stimulation, the response is described as the train-of-four count (TOFC), or the number of responses to TOF stimulation. The post-tetanic count (PTC) defines the level of profound NMB (before a response to TOF stimulation occurs). The response to TOF stimulation is used to guide the dosing of maintenance doses of NMBAs and reversal when NMB is no longer needed. The TOFR is obtained by stimulating a superficial nerve, such as the ulnar nerve, with four supramaximal stimuli at a frequency of 0.5 Hz (over the course of 2 s) and measuring the number and strength of the responses to the stimuli. A TOFR =0.5 would have four responses to stimulation and the 4th response would be 50% the strength of the first response. Adequate recovery of neuromuscular function, defined as a TOFR ≥0.9, is the degree of recovery necessary for safe extubation of the trachea. Residual paralysis, a TOFR <0.9, is associated with postoperative pulmonary complications due to a decreased ability to protect the airway associated with an increased incidence of micro-aspirations and hypoxia, death, decreased patient satisfaction, and longer stays in the post-anesthesia care unit. It is both iatrogenic and avoidable.
The incidence of inadequate recovery of neuromuscular function occurs frequently and has been described in as many as 65% of patients receiving NMBAs (1). Some of the reasons that residual NMB occurs with any frequency include:
- Profound depths of NMB are maintained intraoperatively.
- Recovery from NMB varies from one individual to the next and is, in many cases impacted by the presence of co-morbidities.
- Reversal agents are not dosed as recommended and their administration is not based on the TOFR.
- While optimal dosing of NMBAs and reversal agents is based on the results of monitoring, monitoring depth of NMB is not routine.
- Complete recovery from NMB, even after administration of a reversal agent, is not guaranteed.
Adequate recovery of neuromuscular function depends on appropriate dosing of NMBAs and reversal agents as well as intraoperative quantitative monitoring of depth of NMB. As will be described in this review, use of these guiding principles can minimize the risk of residual NMB in patients receiving NMBAs and decrease its associated risks. We present this article in accordance with the Narrative Review reporting checklist (available at https://atm.amegroups.com/article/view/10.21037/atm-23-1743/rc).
Methods
Research for this review was accomplished through a literature search of PubMed, a free resource that supports the identification of scientific literature based on topic area and other identifying features (Table 1). The PubMed database has more than 36 million citations and abstracts of biomedical literature. It does not consistently provide links to the selected references. PubMed has been available to the public as an online resource since 1996. It was developed and is maintained by the National Center for Biotechnology Information, at the US National Library of Medicine. Key words used in the search included: nondepolarizing NMBAs, cisatracurium, rocuronium, vecuronium, pharmacodynamics of NMBAs, reversal agents, anticholinesterases, neostigmine, selective relaxant binding agents, sugammadex, residual NMB, incidence of residual NMB, complications of residual NMB, monitoring depth of paralysis, quantitative monitoring, qualitative monitoring, clinical monitoring for weakness, and adequacy of recovery of neuromuscular function. While the focus of the search was to provide more recent literature to support recommended changes, older articles were included when necessary to provide context for the more recent work.
Table 1
Items | Specification |
---|---|
Date of search | Search originally initiated 2023/3/22 and was expanded with the article revision 2023/8/23 |
Databases and other sources searched | PubMed |
Search terms used | Nondepolarizing neuromuscular blocking agents, cisatracurium, rocuronium, vecuronium, pharmacodynamics of neuromuscular blocking agents, reversal agents, anticholinesterases, neostigmine, selective relaxant binding agents, sugammadex, residual neuromuscular blockade, incidence of residual neuromuscular blockade, complications of residual neuromuscular blockade, monitoring depth of paralysis, quantitative monitoring, qualitative monitoring, clinical monitoring for weakness, and adequacy of recovery of neuromuscular function |
Timeframe | 1976–2023 |
Inclusion and exclusion criteria | Articles were published in English and includes clinical trials, retrospective analysis, clinical series. Case reports were excluded from the study |
Selection process | The search was conducted by Drs. C.A.L. and M.F. |
Decreasing the incidence of residual NMB
Monitoring
Avoiding residual NMB is difficult. It requires monitoring of depth of NMB so that unnecessary overdosing of NMBAs can be avoided, maintenance doses of NMBAs are administered at the appropriate time, the correct dose of reversal agent, whether it’s an anticholinesterase (neostigmine) with an antimuscarinic agent (glycopyrrolate) to minimize the incidence of indiscriminate systemic actions of acetylcholine (bradycardia) or a selective relaxant binding agent (sugammadex) can be administered, and patients are extubated only once they have fully recovered muscle strength. Accurate assessment of depth of paralysis is paramount to administering either additional doses of NMBAs or reversal agents. Historically three types of assessments have been used, the clinical exam, qualitative assessment, and quantitative monitoring of depth of paralysis. Each of these modalities has its challenges and only quantitative assessment can provide an accurate determination of the depth of NMB.
Clinical assessment of depth of NMB
Clinical assessment may include the presence of a curare cleft in the measured concentration of exhaled end-tidal carbon dioxide (EtCO2), the ability of a patient to lift their head or legs up from a stretcher and maintain that position for 5 s, and a sustained strong hand grip. These assessments cannot indicate with any certainty that a patient either requires an additional dose of NMBA or has fully recovered from NMB.
Capnography in the mechanically ventilated patient is used to determine the adequacy of ventilation and the presence of physiologic conditions that may impact EtCO2. The presence of a cleft in an EtCO2 tracing (Figure 1) indicates movement of the diaphragm. For movement of the diaphragm to be suppressed, a patient needs to be deeply paralyzed (have a PTC of 3–5) or be anesthetized deeply enough to suppress respiratory drive. For reference, a PTC is elicited by providing a tetanic stimulus (50 Hz) to a superficial motor nerve for 5 s, waiting 3 s and then stimulating the same nerve with single twitch stimuli at a frequency of 1 Hz. The PTC is the number of muscle contractions (adduction of the thumb) that occurs in response to the 1 Hz stimuli. PTC can be used to assess depth of NMB when the block is too profound to allow a response to TOF stimulation.
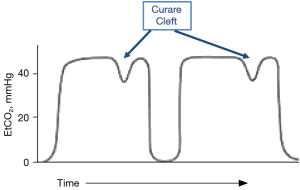
The diaphragm is relatively resistant to NMBAs and recovers more quickly from NMB than the adductor pollicis (2). The varied responses of different muscle groups to NMBAs has been attributed to muscle type, with fast twitch muscles being relatively resistant to NMBAs (3-5), muscle fiber size, with sensitivity increasing with fiber size (6), and blood flow, with faster onset and relative resistance in muscles with greater blood flow (7,8). All dosing recommendations for NMBA and reversal agents are based on the response of the adductor pollicis to ulnar nerve stimulation.
Similarly, work from the 1970s suggested that vital capacity and inspiratory force could be surrogates for adequacy of recovery as both were significantly reduced at a TOFR <0.6 (9,10). However, the muscles of respiration recover from NMB earlier than the adductor pollicis and an adequate tidal volume cannot, by itself, be considered a reliable gauge of recovery from NMB (11). Subsequent work by Donati et al. (2) confirmed that the diaphragm recovers more quickly from NMB than the adductor pollicis. The orbicularis oculi and the laryngeal musculature also recover more quickly than the adductor pollicis. Since adequate recovery of neuromuscular function is now considered a TOFR ≥0.9, rather than a TOFR ≥0.7 as it was at the time of these studies, respiratory function in the intubated patient will certainly recover before there is adequate recovery of strength in the adductor pollicis. Recommendations to not use recovery of ventilatory parameters, including end-tidal CO2, tidal volume, minute ventilation and peak inspiratory flow, as an indicator of adequacy of recovery of neuromuscular function have been recognized in veterinary medicine (12).
Although, the muscles of respiration recover earlier than the more peripherally located neuromuscular units, respiratory failure and pneumonia occur more commonly in patients who are inadequately recovered from NMB. In addition to decreased lower esophageal tone and discoordination of breathing relative to swallowing leading to increased likelihood of aspiration, residual neuromuscular block impairs the hypoxic ventilatory response through depression of carotid body chemoreceptor function (13,14). This impairment of the hypoxic ventilatory response has been documented in unanesthetized volunteers receiving either vecuronium or atracurium (15,16).
A “correct” head lift is done when a patient can lift their head off a surface from a supine position and maintain that position for 5 s. Historically, the ability to accomplish this task was assumed to indicate that the patient has regained enough muscle strength to breath spontaneously and protect their airway once the endotracheal tube was removed. A 5-s leg lift was presumed to provide the same information and in children, who may not follow a request to lift their heads, could be used as a surrogate clinical measure. However, a study published in 1997 found that young, healthy volunteers could perform either of these tasks when their TOFR was <0.6 (17), the TOFR at which vital capacity, inspiratory force, and peak expiratory flow rate were at clinically acceptable values (9).
Qualitative assessment of depth of NMB
Qualitative assessment of the depth of neuromuscular block offers some advantage over clinical assessment in that it requires neither patient effort nor cooperation. That being said, there are several requirements for this type of assessment to even begin to deliver usable information (Table 2). The “twitch monitors” as they are commonly referred to must be able to deliver an adequate supramaximal current, they must be able to provide a TOF stimulus (4 stimuli given every 0.5 s) and they must have the ability to deliver a stimulus of 0.2–0.3 ms pulse duration, an output of more than 60 mA in the presence of 2,000 Ohm impedance, and a digital ammeter to display the delivered current. Even if a monitor were to have all of these features, visual or tactile interpretation of the response to stimulation would not be accurate. To interpret the response to stimulation, one looks for, or feels, the contractions resulting from stimulation, counts the responses and, if there are 4 responses, determines the strength of the 4th response relative to the 1st. While counting the number of responses to stimulation may seem simple—a study in a critical care unit demonstrated the difficulty associated with establishing consistency in the interpretation of the number of responses to a stimulus. It is even more difficult to assess fade in the TOFR and, unless the 4th response is less than 40% of the 1st response, fade cannot be reliably detected (18). This means that a patient may have a TOFR =40% and be assessed as having 4 equal responses to TOF stimulation, and therefore, erroneously erroneously determined to be adequately recovered from NMB. Qualitative assessment of the TOF count or ratio requires that a stimulus is applied through a peripheral nerve stimulator to a superficially located motor nerve and the muscular response to this stimulation is assessed or “measured”. While any superficially located motor nerve can be stimulated to elicit a muscle contraction, NMBA and reversal dosing recommendations are based on the response of the adductor pollicis to stimulation of the ulnar nerve. Because the patient’s arms are frequently tucked by their side for surgery, or simply because it is more easily accessed, the facial nerve, which innervates the orbicularis oculi muscle is commonly used intraoperatively to assess depth of NMB. Although more convenient and accessible, the orbicularis oculi more closely reflects the onset of NMB and will, under the best of circumstances, overestimate the degree of recovery of neuromuscular function. Optimal monitoring at the orbicularis oculi requires that the stimulating electrodes be placed as far apart as is possible (not adjacent to each other over the patient’s eyebrow) as is shown in Figure 2 and that the maximal stimulating current used is 40 mA, or less, in order to minimize the likelihood of direct muscle stimulation. Even when monitored correctly, the response of the orbicularis oculi does not reflect recovery at the adductor pollicis. When NMB is monitored at the orbicularis, patients are more likely to have an inadequate recovery of neuromuscular function on admission to the post-anesthesia care unit (19).
Table 2
The monitor must have specific characteristics including |
Ability to generate a supramaximal stimulus (at least 30–60 mA) |
Ability to generate a stimulus of the proper duration (0.2–0.3 ms) |
Adequate output in the presence of 2,000 Ω impedance |
Ability to provide a train-of-four stimulus |
Presence of a digital ammeter |
Electrodes must be placed properly |
The different responses of monitoring sites (sensitivity, onset, and recovery) to NMBAs must be understood |
The digit being assessed needs to be able to move freely or clearly visible |
NMBA, neuromuscular blocking agent.
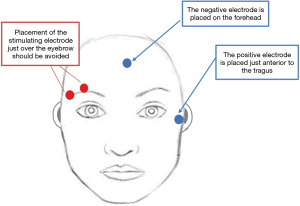
Ideally, more peripherally located neuromuscular units are used for monitoring. All dosing recommendations for neuromuscular blocking and reversal agents have been based on the response of the adductor pollicis to ulnar nerve stimulation. Onset of neuromuscular block at the adductor pollicis is slower than it is at the orbicularis, larynx and diaphragm. Similarly, recovery occurs more slowly in the adductor pollicis than is does in these other muscle groups. As shown in Figure 3, when the arms are not available, strength can be monitored in the lower extremities by either stimulating the common peroneal nerve and assessing dorsiflexion of the foot or the posterior tibial nerve and assessing plantar flexion of the great toe. The utility of using the lower extremity for monitoring the depth of NMB has not been studied extensively. One study demonstrated slower recovery than at the adductor pollicis (20) and another found that the flexor hallucis brevis recovered more quickly (21).
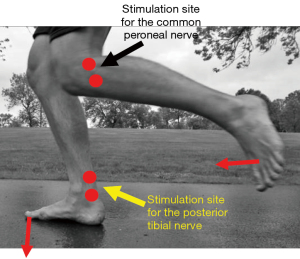
Quantitative assessment of depth of NMB
Quantitative assessment of the depth of NMB is the only monitoring methodology that allows determination, with certainty, of depth of NMB (22-24). As with qualitative monitoring, a stimulus (TOF, or PTC) is applied to a superficially located motor neuron. In contrast to a qualitative monitor, though, the response to stimulation is measured by the device. While the early studies of muscle strength during onset and recovery from neuromuscular block used mechanomyography to measure the strength of contraction of the adductor pollicis in response to stimulation of the ulnar nerve, this monitoring modality is complex in its set-up, and not available for routine clinical use. Available quantitative monitors, monitor one of two responses to neural stimulation of a motor nerve. Acceleromyography determines the acceleration of movement of the thumb in response to stimulation of the ulnar nerve and electromyography measure electrical activity in response to neural stimulation. While each of these modalities has its advantages and disadvantages as outlined in Table 3, the use of either has been shown in many clinical trials to decrease the likelihood of unacceptable levels of NMB after an anesthetic. As with qualitative monitoring, neither modality requires patient cooperation. Guidelines recommending quantitative monitoring of NMB whenever a patient receives a NMBA have now been instituted through the American Society of Anesthesiologists, the European Society of Anaesthesiology and Critical Care (25), and the Spanish Society of Anaesthesiology and Resuscitation (26).
Table 3
Monitoring modality | Advantages | Disadvantages |
---|---|---|
Acceleromyography | Relatively easy to set up | Thumb has to be able to move in response to neural stimulation |
Provides the measured depth of neuromuscular blockade in an easy-to-see format | Degree of recovery may be overestimated unless the response to stimulation is normalized | |
If the hand is not available to monitor, the monitor can be used on a lower extremity | Integration with the electronic medical record may be complex | |
Subject to electrical interference | ||
Electromyography | Relatively easy to set up | Integration with the electronic medical record may be complex |
The thumb does not have to move for detection of muscle strength | ||
Provides the measured depth of neuromuscular blockade in an easy-to-see format | Subject to electrical interference | |
If the hand is not available to monitor, the monitor can be used on a lower extremity | ||
Measured responses are the same as those obtained with mechanomyography; the degree of recovery is not over estimated |
Nondepolarizing NMBAs
Duration of action
There have been many reviews of NMBAs (27-31) and repeating that information is beyond the scope of this chapter. NMBAs were developed to facilitate endotracheal intubation, provide a still surgical field, and allow mechanical ventilation. There have been a number of different nondepolarizing NMBAs used over the years. The majority of them have had one of two basic structures—either steroidal or benzylisoquinoline and all have been classified on their durations of actions (short-, intermediate-, or long-). Nondepolarizing NMBAs in the most simplistic of descriptions, cause NMB by competitively inhibiting the acetylcholine receptor in the neuromuscular junction of the muscle membrane by binding to one or both of the receptor’s acetylcholine binding sites. With this inhibition, the acetylcholine receptor cannot be activated to allow the influx of sodium and efflux of potassium required for neuromuscular transmission. As briefly discussed later in this review, NMBAs also interact with the presynaptic neuronal acetylcholine receptors to impact the release of acetylcholine from the nerve terminal after the application of a stimulus.
In the current practice of anesthesiology, the most commonly used NMBA is rocuronium followed by much less frequent use of vecuronium and cisatracurium (32). Each of these compounds is an intermediate-acting NMBA, which means that following administration of an intubating dose, the time to recovery to T1 (the first response in the TOFR) to 25% of its baseline value is between 20 and 50 min (33). The differences in the NMBAs are outlined in Table 4.
Table 4
NMBA | Chemical structure | Elimination/metabolism | ED95 (mg/kg) | Intubating dose (mg/kg) | Multiples of ED95 | Time to max block (min) after 2× ED95 | Clinical duration (min) |
---|---|---|---|---|---|---|---|
Rocuronium | Steroidal | Renal and biliary elimination | 0.3 | 0.6–1.0 | 2–3.3 | 1.7 | 36 |
Vecuronium | Hepatic metabolism, renal and hepatic elimination | 0.05 | 0.1–0.2 | 2–4 | 2.4 | 41 | |
Cisatracurium | Benzylisoquinolinium | Hofmann degradation and renal elimination | 0.05 | 0.15–0.2 | 3–4 | 7.7 | 46 |
NMBA, neuromuscular blocking agent; ED95, the dose of a NMBA that will, on average, cause 95% suppression of the muscle response to stimulation.
Dosing
The ED95 of a NMBA is the dose that, on average, will cause 95% suppression of the single twitch response to stimulation of the ulnar nerve. Onsets of NMBA vary with the neuromuscular unit being monitored (2) and the potency of the NMBA. Table 5 lists the ED95 of several NMBAs and the onset of action of an ED95 dose. When comparing the onset of effect of equipotent of cisatracurium, vecuronium, rocuronium, and succinylcholine, the least potent compounds, rocuronium and succinylcholine, have the most rapid onset of effect (34). To decrease the time required for onset of block and to better guarantee that complete NMB will occur following a single dose, the recommended intubating dose of a NMBA is typically 2–4 times the ED95 (Table 4). The increase in dose in order to achieve a more rapid onset results in an increased clinical duration of action. It is this increase in clinical duration of action as well as an increased likelihood of adverse reactions with larger doses that limit the size of an intubation dose when speed of onset is important.
Table 5
NMBA | ED95 (mg/kg) | Onset time (min) of 2× ED95 | Minutes to 25% recovery |
---|---|---|---|
Short-acting neuromuscular blocking agents | |||
Mivacurium | 0.08 | 3–4 | 15–20 |
Intermediate-acting neuromuscular blocking agents | |||
Atracurium | 0.25 | 3–4 | 35–45 |
Cisatracurium | 0.05 | 5–7 | 35–45 |
Rocuronium | 0.3 | 1.5–2 | 30–40 |
Vecuronium | 0.05 | 2–3 | 35–45 |
Long-acting neuromuscular blocking agents | |||
Pancuronium | 0.07 | 2–4 | 60–120 |
NMBA, neuromuscular blocking agents; ED95, the dose of a NMBA that will, on average, cause 95% suppression of the muscle response to stimulation.
Variability of duration of action
Interpatient variability in response to an intubating dose and speed of recovery is often overlooked and is an important factor to consider when administering NMBAs. When the ED95 of a NMBA is described, it is often described as a single value in a study. Similarly, when recovery is described, it is most commonly the mean value that is reported along with the standard deviation. There is a significant amount of interpatient variability in recovery amongst young, healthy patients of ideal body weight (35). In elderly patients, this difference becomes greater with the steroidal NMBAs. The increase in duration of action and interpatient variability observed in geriatric patients are not observed following the administration of benzylisoquinolinium NMBAs. This is likely due to the metabolism of the benzylisoquinoliniums rather than dependence on hepatic and renal systems for inactivation and elimination.
Reversal agents
Neostigmine and sugammadex
There are two different classes of reversal agents: anticholinesterases (neostigmine, edrophonium, pyridostigmine) of which neostigmine is the one that is currently available for clinical use (36), and selective relaxant binding agents, of which sugammadex is used clinically (Figure 4). Administration of these reversal agents will increase muscle strength after administration of an NMBA. Neostigmine works as an indirect antagonist, inhibiting acetylcholinesterase—which is responsible for the breakdown of acetylcholine. The result is that the acetylcholine released from the presynaptic terminal is not metabolized and the levels of acetylcholine at the neuromuscular junction are increased allowing the competitive NMB of acetylcholine receptors to be at least partially overcome and neuromuscular transmission increased. Once acetylcholinesterase is completely inhibited, administration of additional anticholinesterase will not have any effect and, if it administered when there are significant concentrations of NMBAs present, the competitive neuromuscular block cannot be overcome. Sugammadex encapsulates free rocuronium in the plasma so that it cannot enter the neuromuscular junction and bind to acetylcholine receptors (37,38) and it increases the movement of rocuronium from the neuromuscular junction to the plasma by decreasing the concentration of free rocuronium there (37,39,40). One molecule of sugammadex binds noncovalently to 1 molecule or rocuronium, incorporating its steroidal rings into its hydrophobic central core. The affinity constant value of rocuronium for sugammadex is 25,000,000 M, which is about 2.5 times greater than the affinity of vecuronium (38) for sugammadex and, once bound, there is no evidence that the complex dissociates appreciably.
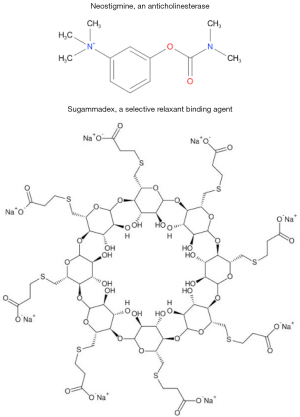
Dosing of reversal agents
Neostigmine cannot reverse deep NMB and in the recently published guidelines (Table 6) is not recommended for reversal of anything other than shallow depths of neuromuscular block, 0.4≤ TOFR <0.9 (41). Stated differently, neostigmine should not be used to antagonize residual neuromuscular block when the TOFC <4, and when fade is appreciable in the TOF response. If used to reverse these deeper levels of NMB, there will be some increase in muscle strength. However, recovery will likely not be complete. Since there will be some increase in muscle strength, though, residual NMB may not be appreciated unless a quantitative monitor of NMB is being used. Larger doses of neostigmine will cause greater inhibition of acetylcholinesterase and perhaps facilitate greater recovery. Once the enzyme is maximally inhibited, though, administration of additional neostigmine will have no further impact on the concentration of acetylcholine and residual neuromuscular block will persist if significant levels of NMBA are present at the neuromuscular junction. This limited degree of efficacy was demonstrated many years ago with the attempted neostigmine reversal of 60% and 95% pancuronium-induced block (42). The authors found that, contrary to what would have been expected, excessive doses of neostigmine caused increased fade in the TOFR with repeated stimulation (43,44). Similarly, unwarranted administration of neostigmine will cause decreased grip strength (45) and, in animals, impairs normal function of the musculature of the airway (46,47).
Table 6
Response to stimulation | Sugammadex dose (mg/kg) | Neostigmine dose (mcg/kg) |
---|---|---|
PTC =0 | 16 | |
PTC =2 to TOFC =1 | 4 | |
TOFC =2, 3, or 4 (if TOFR <0.9) | 2 | |
TOFC =4 and 0.4≤ TOFR <0.9 | 30 |
PTC, post-tetanic count; TOFC, train-of-four count; TOFR, train-of-four ratio.
Of note, NMBAs also bind to presynaptic acetylcholine receptors causing decreased release of acetylcholine in response to neural stimulation and inhibition of neuromuscular transmission (48,49) as demonstrated by increased fade in the TOF. Conversely, in the presence of large concentrations of acetylcholine, the neuromuscular blockade becomes noncompetitive (49), further complicating attempts to reverse deep levels of NMB with anticholinesterase.
Smaller doses of neostigmine 30 µg/kg or less, are effective in reversing shallow degrees of residual NMB (44,50-52). Since full reversal doses of neostigmine (50–70 mcg/kg) can cause neuromuscular block when administered unnecessarily recommendations are to administer smaller doses of neostigmine to reverse shallow depths of NMB (41).
The mechanism of action of sugammadex is completely different than that of neostigmine. While neostigmine is an indirect antagonist, inhibiting acetylcholinesterase and increasing the amount of acetylcholine at the neuromuscular junction, sugammadex is a direct antagonist, encapsulating the steroidal NMBAs so that they cannot enter the neuromuscular junction and bind to acetylcholine receptors (37,38). Sugammadex was designed to specifically interact with the steroidal NMBAs (rocuronium > vecuronium > pancuronium) and will not reverse NMB induced with benzylisoquinoliniums (atracurium, cisatracurium).
Sugammadex has a much more rapid onset of effect than neostigmine, 1 to 2 minutes versus 9–10 (53), and, in contrast to neostigmine, administration of a large dose, 16 mg/kg, will rapidly antagonize profound levels of neuromuscular block.
The definition of an appropriate dose is still being examined and smaller doses of sugammadex have been found to effectively antagonize shallow depths of NMB (a TOFR =0.5) (50). When considering that the same dose of sugammadex is currently recommended for depths of NMB ranging from a PTC of 2 to a TOF count of 1, and a TOF count of 2 to a TOFR <0.9, either of which is a very wide range of depth of NMB, there is room for greater delineation of an “optimal” dose of sugammadex for different levels of block. A recent dose-finding study identified a range of doses necessary to reverse NMB at the conclusion of cardiac surgery (54). The study results indicated that there was significant inter-patient variability in the response to sugammadex, that 87% of patients required less sugammadex than would have been administered based on current recommendations, and 13% of patients required more sugammadex.
Significantly decreasing the dose of sugammadex beyond those currently recommended may increase the likelihood of inadequate recovery of neuromuscular function (55,56). If quantitative monitoring is not being used, the inadequate recovery of neuromuscular function may not be detected. As with all neuromuscular blocking and reversal agents, there is interpatient variability in the response to a dose of sugammadex. Even administration of a correct dose of sugammadex, while it is more likely to result in complete reversal of neuromuscular function, does not guarantee adequate reversal of residual NMB (57). Recurarization can occur even after complete recovery of neuromuscular function following sugammadex administration (54) and the likelihood of residual NMB, even after administration of an appropriate dose of sugammadex, is increased especially if considering a TOFR =1, rather than 0.9, as the endpoint of recovery (58).
Neostigmine and sugammadex in patients with renal failure
Of the currently used NMBAs all have some component of renal elimination (59-61), Vecuronium and its metabolites have the greatest renal elimination and vecuronium has a prolonged duration of action in patients with decreased renal function, as well as the decreases in renal blood flow associated with advanced age (62). Neostigmine is also eliminated through the kidneys and has a decreased clearance in patients with renal disease (63). Therefore, its dosing does not have to be adjusted in patients with renal failure even when reversing NMB induced by vecuronium—which has a longer duration of action in patients with end-stage renal disease (60).
Recovery of neuromuscular function after administration of sugammadex is due to the binding of rocuronium by sugammadex, rather than the elimination of the complex from the body (64). Because of this, even though the sugammadex and the sugammadex-rocuronium complex are eliminated in the urine (65), sugammadex is effective in patients with end-stage renal disease. Since the rocuronium—sugammadex complex will not dissociate, even though the complex remains in the plasma, the rocuronium remains associated with the sugammadex and is unable to enter the neuromuscular junction (66,67). In patients with end-stage renal disease the rocuronium-sugammadex is dialyzable (68).
Pharmacologic interactions of reversal medications
Both neostigmine and sugammadex interact with other medications. Neostigmine appears to potentiate anti-inflammatory medications, will inhibit plasma cholinesterase and prolong the duration of action of medications metabolized by this enzyme, such as succinylcholine and mivacurium (69,70). Additionally, neostigmine may reduce the effectiveness of tricyclic antidepressants, such as amitriptyline and has been used to treat tricyclic antidepressant overdoses (71). On the other hand, the main pharmaceutical interaction with sugammadex is concern that it may bind to, and reduce the levels of, progesterone within the body (72). This is of special in individuals taking hormonal, progesterone-based, contraceptives and the manufacturer recommends that individuals using hormonal birth control use an additional contraceptive for 7 days after exposure to sugammadex. While sugammadex may reduce progesterone levels within the body, there has been no evidence supporting the assumption that the levels will drop enough to cause early cessation of pregnancy. In fact, there is no evidence in humans and a trial in which rats were exposed to high doses of sugammadex in the first trimester did not increase the rates of stillbirth (73,74). Since national consensus guidelines recommend the use of rocuronium as an alternative to succinylcholine for rapid sequence induction in pregnant patients (75), one can expect that sugammadex will be used to reverse NMB in the “cannot intubate – cannot ventilate” scenario. There is no published data on the presence of sugammadex in human breast milk. Because of its large molecular size and polarization, though, there is likely to be little maternal-fetal transfer of sugammadex (72,76,77).
Residual NMB
Incidence
Residual NMB has been recognized since the 1970’s when long-acting NMBAs were used (Table 7). Adequate recovery of neuromuscular function at that time was defined as a TOFR ≥0.7 and 42% of patients were identified as having a TOFR <0.7 on admission to the recovery room after having received pancuronium, gallamine, or d-tubocurarine (78). Almost 10 years later, after the introduction of atracurium and vecuronium into clinical practice, a similar observational study was done. In this study, 36% of patients were found to have a TOFR <0.7 if they had received pancuronium while only 5% of patients who had received either of the intermediate-acting NMBAs had a TOFR <0.7 (79). In addition to the availability of atracurium and vecuronium, the difference in the more recent study was that qualitative monitors for assessment of depth on NMB were available. Based on these results, one could have easily expected the incidence of residual NMB to decrease with the increased use of intermediate-acting NMBAs. However, as the effects of residual NMB were increasingly recognized, the definition of adequate recovery of neuromuscular function was changed from a TOFR ≥0.7 to ≥0.9. With this, as well as changing surgical practices, the incidence of residual NMB following administration vecuronium, rocuronium or atracurium (TOFR <0.9) was 37% (82). More recently, The RECITE-US study (1) found that the incidence of unacceptable levels of recovery in patients receiving NMBAs across community and academic medical centers was almost 65%. In this study, risk factors for residual NMB included male gender, increased body mass index, and surgery at a community hospital. Other independent risk factors for residual paralysis have been identified and include abdominal surgery, advanced age and surgery of longer duration (62,83,84).
Table 7
Year (reference) | Neuromuscular blocking agent | TOFR in the PACU | % of patients |
---|---|---|---|
1979 (78) | Pancuronium, gallamine, D-tubocurarine | <70% | 42 |
1988 (79) | Pancuronium | <70% | 36 |
Atracurium, vecuronium | <70% | 5 | |
2003 (80) | Atracurium, vecuronium, rocuronium | <90% | 37 |
2008 (81) | Rocuronium | <90% | 30 |
2019 (1) | Rocuronium | <90% | 40** |
**, at Academic Medical Centers. TOFR <0.9 at Academic and Community hospitals combined was 65%. TOFR, train-of-four ratio; PACU, postanesthesia care unit.
Adverse effects of residual NMB
Residual NMB, defined as a TOFR <0.9, is a concern because of the adverse consequences with which it is associated (Table 8). These adverse effects range from relatively minor (such as generalized fatigue) (17) to significant (respiratory failure requiring reintubation) (85)—with some of these adverse effects persisting beyond recovery of neuromuscular function to a TOFR =1.0 (86).
Table 8
Prolonged postoperative ventilatory weaning times in cardiac surgery patients |
Visual disturbances, including diplopia |
General fatigue |
Decreased patient satisfaction |
Delayed discharge from the post-anesthesia care unit |
Increased risk of critical respiratory events, upper airway obstruction, postoperative hypoxemia, postoperative pulmonary complication |
Decreased upper esophageal motor tone |
Decreased sensitivity to hypercapnea |
Impaired ventilatory response to hypoxia |
Reintubation |
Death |
TOFR, train-of-four ratio.
Of the adverse effects of residual NMB, its interference with the hypoxic ventilatory response is one of the least discussed. Since the diaphragm is relatively resistant to NMBAs and because it recovers from NMB more quickly than the adductor pollicis (2), it is possible for patients to breath when inadequately recovered from NMB (11). However, in addition to being more likely to develop a critical respiratory event (81), the hypoxic respiratory drive remains attenuated even with recovery to a TOFR ≥0.9 (86). As described previously in this chapter, NMB depresses carotid body chemoreceptor function resulting in an impaired hypoxic ventilatory response (13,14).
While the use of NMBAs has been demonstrated to decrease mortality in critically ill, ventilated patients (87,88), several databased studies have found an association between intraoperative use of NMBAs and increased morbidity and mortality. Retrospective studies from the 1970–1980’s found that there was a 2–3% increase in mortality when NMBAs were administered that was most commonly associated with compromised respiratory function (89,90). While this represented an improvement over the 6-fold increase in mortality described by Beecher and Todd in 1954 (91), it is a significant increase in risk especially when the reported risk of mortality associated with anesthesia is between 0.01% and 0.02% (92). More recently, high doses of intermediate-acting NMBAs were found to be associated with an increased rate of respiratory complications, readmission, prolonged length of stay, and increased hospital costs (93-95). Bronsert et al. (93) described a decrease in that risk when residual NMB was antagonized. Because these are retrospective databased trials, only the relationship between NMBAs and mortality, but not causality, can be described.
Adequate recovery of neuromuscular function
Definition of adequate recovery of neuromuscular function
The definition of adequate recovery of neuromuscular function after administration of a NMBA has evolved over time as indicated by endpoints for monitoring muscle strength (Table 8). Revision of the standard for adequate recovery occurred as the negative impact of residual NMB was increasingly appreciated, the duration of action of our NMBAs became shorter, our ability to monitor depth of NMB evolved, surgical practice advanced, and our ability to rapidly reverse residual NMB improved (Figure 5).
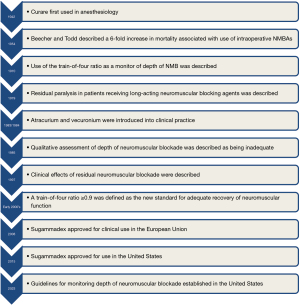
Quantitative monitoring of depth of NMB is essential not only to guide intraoperative dosing of NMBAs but, as discussed previously in this review, to also determine the dose of reversal agent that should be administered and to verify that adequate recovery of neuromuscular function has occurred at the completion of surgery. While quantitative monitoring improves a clinician’s ability to identify residual NMB, the monitors must work reliably even in an environment that is bound to provide both electrical interference and movement of the extremity where the monitoring is being done. Improved monitoring is essential since evidence, both old and new, indicates that a TOFR ≥0.9 may not reflect “full” recovery of neuromuscular function. There is a significant amount of redundancy built into neuromuscular transmission allowing for appropriate functioning of the system even in times of stress. Decreases in functional acetylcholine receptors in patients with myasthenia correlate with the fatigue and weakness (96). In a related fashion, 80% of acetylcholine receptors may be blocked before depression in response to twitch response can be appreciated and recovery of muscle strength can begin even with 80% of receptors occupied (97). Residual occupancy by nondepolarizing NMBAs and significant plasma concentrations of NMBAs during recovery of neuromuscular function (98), may account at least in part for the greater sensitivity patients have for subsequent doses of NMBAs (99), and is the reason that doses of approximately 20–40% of an ED95 of a NMBA are recommended for maintenance of NMB after an intubating dose.
Duchenne muscular dystrophy (DMD) causes hypertrophy of muscle tissue due to fatty infiltration and a progressive decrease in muscle strength. Patients with DMD have a greater sensitivity to nondepolarizing NMBAs and a prolonged duration of action of these compounds (100,101). Anticholinesterases (neostigmine and pyridostigmine) can be used to reverse NMB in patients with DMD (64,100); as in otherwise healthy patients, there is marked interpatient variability in response to anticholinesterases. Sugammadex has been used successfully in these patients (102-105), and based on case reports allows complete recovery of neuromuscular transmission within 3 minutes of administration. As complications caused by residual NMB, such as respiratory failure and aspiration, also occur in patients with DMD, ensuring complete recovery of neuromuscular function to baseline after the conclusion of a surgical or diagnostic procedure is exceptionally important.
Is more recovery of neuromuscular function required?
The physiology of pharmacologically-induced NMB is complex and involves, in addition to competitive blockade of the acetylcholine receptors at the subsynaptic or postsynaptic portion of the neuromuscular junction, blockade of prejunctional acetylcholine receptors (48,106). In a study of strength after complete recovery of neuromuscular function, Eikermann found that patients who had completely recovered muscle strength were unable to generate the same force as volunteers who had never received a NMBA (107). More recent work by Broens et al. (86) demonstrated that hypoxic ventilatory response was not restored even once a patient’s TOFR had recovered to ≥0.9 after the administration of either neostigmine or sugammadex. Interestingly, the POPULAR study (108) found that the use of neuromuscular monitoring, and reversal with either sugammadex or neostigmine to a TOFR ≥0.9 did not decrease the incidence of postoperative pulmonary complications. A subsequent re-analysis of this data, though (109), did demonstrate that the risk of postoperative pulmonary complications was decreased when patients were extubated at a TOFR ≥0.95. While the standard for recovery of neuromuscular function remains recovery to a TOFR ≥0.9, the results indicate that there is still more to be learned regarding safety in the use of NMBAs. To do that, monitors of depth of NMB will have to be more accurate and deliver reliable results—and, they will have to be used.
Conclusions
With the increasing use of NMBAs in clinical practice and documented incidence of inadequate recovery of neuromuscular function on arrival to the post-anesthesia care unit, it is imperative that clinicians adapt their practices to decrease the postoperative complications that are secondary to the use of these agents. Eliminating residual NMB is entirely feasible within modern anesthetic practice. Technological research and advancement have made quantitative measurement of depth of NMB possible so that qualitative observation and assessment of recovery of neuromuscular function and all the error associated therewith is avoidable. With quantitative measurement every time a patient receives a NMBA, clinicians can dose NMBAs to provide the necessary depth of NMB intraoperatively, adjust the dose of the reversal agent based on the results of monitoring, ensure complete recovery of neuromuscular function before extubating the patient, and decrease the incidence of the complications associated with residual NMB. Just as using the pulse oximeter in modern practice reduces the frequency of peri anesthetic hypoxia, the use of quantitative neuromuscular monitors of depth of NMB will further enhance the safety of modern anesthesia practice.
Acknowledgments
The authors would like to thank Samantha Cusick for her editorial assistance.
Funding: None.
Footnote
Provenance and Peer Review: This article was commissioned by the Guest Editors (Tobias Eckle and Dr. Benjamin Scott) for the series “Highlights in Anesthesia and Critical Care Medicine” published in Annals of Translational Medicine. The article has undergone external peer review.
Reporting Checklist: The authors have completed the Narrative Review reporting checklist. Available at https://atm.amegroups.com/article/view/10.21037/atm-23-1743/rc
Peer Review File: Available at https://atm.amegroups.com/article/view/10.21037/atm-23-1743/prf
Conflicts of Interest: Both authors have completed the ICMJE uniform disclosure form (available at https://atm.amegroups.com/article/view/10.21037/atm-23-1743/coif). The series “Highlights in Anesthesia and Critical Care Medicine” was commissioned by the editorial office without any funding or sponsorship. C.A.L. is a board member of ASA Task Force on Developing Neuromuscular Guidelines and Foundation for Education, Education, and Research (FAER). C.A.L. also serves on the Scientific Advisory Council of Senzime (Sweden) and serves as the Secretary-Treasurer of Society for Academic Associations of Anesthesiology & Perioperative Medicine (SAAAPM). None of these responsibilities impacted the work on this manuscript. The authors have no other conflicts of interest to declare.
Ethical Statement: The authors are accountable for all aspects of the work in ensuring that questions related to the accuracy or integrity of any part of the work are appropriately investigated and resolved.
Open Access Statement: This is an Open Access article distributed in accordance with the Creative Commons Attribution-NonCommercial-NoDerivs 4.0 International License (CC BY-NC-ND 4.0), which permits the non-commercial replication and distribution of the article with the strict proviso that no changes or edits are made and the original work is properly cited (including links to both the formal publication through the relevant DOI and the license). See: https://creativecommons.org/licenses/by-nc-nd/4.0/.
References
- Saager L, Maiese EM, Bash LD, et al. Incidence, risk factors, and consequences of residual neuromuscular block in the United States: The prospective, observational, multicenter RECITE-US study. J Clin Anesth 2019;55:33-41. [Crossref] [PubMed]
- Donati F, Meistelman C, Plaud B. Vecuronium neuromuscular blockade at the diaphragm, the orbicularis oculi, and adductor pollicis muscles. Anesthesiology 1990;73:870-5. [Crossref] [PubMed]
- Sanders I, Aviv J, Kraus WM, et al. Transcutaneous electrical stimulation of the recurrent laryngeal nerve in monkeys. Ann Otol Rhinol Laryngol 1987;96:38-42. [Crossref] [PubMed]
- Johnson MA, Polgar J, Weightman D, et al. Data on the distribution of fibre types in thirty-six human muscles. An autopsy study. J Neurol Sci 1973;18:111-29. [Crossref] [PubMed]
- Secher NH, Rube N, Secher O. Effect of tubocurarine on human soleus and gastrocnemius muscles. Acta Anaesthesiol Scand 1982;26:231-4. [Crossref] [PubMed]
- Ibebunjo C, Hall LW. Muscle fibre diameter and sensitivity to neuromuscular blocking drugs. Br J Anaesth 1993;71:732-3. [Crossref] [PubMed]
- Wright PM, Hart P, Lau M, et al. Cumulative characteristics of atracurium and vecuronium. A simultaneous clinical and pharmacokinetic study. Anesthesiology 1994;81:59-68; discussion 27A. [Crossref] [PubMed]
- Donati F. Sensitivity of different muscles to neuromuscular-blocking drugs. Current Opinion in Anesthesiology 1991;4:593-8. [Crossref]
- Ali HH, Wilson RS, Savarese JJ, et al. The effect of tubocurarine on indirectly elicited train-of-four muscle response and respiratory measurements in humans. Br J Anaesth 1975;47:570-4. [Crossref] [PubMed]
- Brand JB, Cullen DJ, Wilson NE, et al. Spontaneous recovery from nondepolarizing neuromuscular blockade: correlation between clinical and evoked responses. Anesth Analg 1977;56:55-8. [Crossref] [PubMed]
- Hackett GH, Hughes R, Payne JP. Recovery of spontaneous breathing following neuromuscular blockade with atracurium. Br J Anaesth 1986;58:494-7. [Crossref] [PubMed]
- Martin-Flores M, Sakai DM, Campoy L, et al. Recovery from neuromuscular block in dogs: restoration of spontaneous ventilation does not exclude residual blockade. Vet Anaesth Analg 2014;41:269-77. [Crossref] [PubMed]
- Wyon N, Joensen H, Yamamoto Y, et al. Carotid body chemoreceptor function is impaired by vecuronium during hypoxia. Anesthesiology 1998;89:1471-9. [Crossref] [PubMed]
- Igarashi A, Amagasa S, Horikawa H, et al. Vecuronium directly inhibits hypoxic neurotransmission of the rat carotid body. Anesth Analg 2002;94:117-22. table of contents. [Crossref] [PubMed]
- Eriksson LI, Lennmarken C, Wyon N, et al. Attenuated ventilatory response to hypoxaemia at vecuronium-induced partial neuromuscular block. Acta Anaesthesiol Scand 1992;36:710-5. [Crossref] [PubMed]
- Eriksson LI. Reduced hypoxic chemosensitivity in partially paralysed man. A new property of muscle relaxants? Acta Anaesthesiol Scand 1996;40:520-3. [Crossref] [PubMed]
- Kopman AF, Yee PS, Neuman GG. Relationship of the train-of-four fade ratio to clinical signs and symptoms of residual paralysis in awake volunteers. Anesthesiology 1997;86:765-71. [Crossref] [PubMed]
- Viby-Mogensen J, Jensen NH, Engbaek J, et al. Tactile and visual evaluation of the response to train-of-four nerve stimulation. Anesthesiology 1985;63:440-3. [Crossref] [PubMed]
- Thilen SR, Hansen BE, Ramaiah R, et al. Intraoperative neuromuscular monitoring site and residual paralysis. Anesthesiology 2012;117:964-72. [Crossref] [PubMed]
- Kitajima T, Ishii K, Kobayashi T, et al. Differential effects of vecuronium on the thumb and great toe as measured by accelography and electromyography. Anaesthesia 1995;50:76-8. [Crossref] [PubMed]
- Kern SE, Johnson JO, Orr JA, et al. Clinical analysis of the flexor hallucis brevis as an alternative site for monitoring neuromuscular block from mivacurium. J Clin Anesth 1997;9:383-7. [Crossref] [PubMed]
- Domenech G, Kampel MA, García Guzzo ME, et al. Usefulness of intra-operative neuromuscular blockade monitoring and reversal agents for postoperative residual neuromuscular blockade: a retrospective observational study. BMC Anesthesiol 2019;19:143. [Crossref] [PubMed]
- Ross Renew J, Brull SJ. The Effect of Quantitative Neuromuscular Monitoring on the Incidence of Residual Neuromuscular Blockade and Clinical Outcomes. Current Anesthesiology Reports 2016;6:170-7. [Crossref]
- Brull SJ, Murphy GS. Residual neuromuscular block: lessons unlearned. Part II: methods to reduce the risk of residual weakness. Anesth Analg 2010;111:129-40. [Crossref] [PubMed]
- Fuchs-Buder T, Romero CS, Lewald H, et al. Peri-operative management of neuromuscular blockade: A guideline from the European Society of Anaesthesiology and Intensive Care. Eur J Anaesthesiol 2023;40:82-94. [Crossref] [PubMed]
- Díaz-Cambronero O, Serrano A, Abad-Gurumeta A, et al. Perioperative neuromuscular blockade. 2020 update of the SEDAR (Sociedad Española de Anestesiología y Reanimación) recommendations. Rev Esp Anestesiol Reanim (Engl Ed) 2023;70:37-50. [Crossref] [PubMed]
- Hunter JM. New neuromuscular blocking drugs. N Engl J Med 1995;332:1691-9. [Crossref] [PubMed]
- Sparr HJ, Beaufort TM, Fuchs-Buder T. Newer neuromuscular blocking agents: how do they compare with established agents? Drugs 2001;61:919-42. [Crossref] [PubMed]
- Heerdt PM, Sunaga H, Savarese JJ. Novel neuromuscular blocking drugs and antagonists. Curr Opin Anaesthesiol 2015;28:403-10. [Crossref] [PubMed]
- Farooq K, Hunter JM. Neuromuscular blocking agents and reversal agents. Anaesthesia & Intensive Care Medicine 2017;18:279-84. [Crossref]
- Pollard BJ. Neuromuscular blocking agents and reversal agents. Anaesthesia & Intensive Care Medicine 2005;6:189-92. [Crossref]
- Weber V, Abbott TEF, Ackland GL. Reducing the dose of neuromuscular blocking agents with adjuncts: a systematic review and meta-analysis. Br J Anaesth 2021;126:608-21. [Crossref] [PubMed]
- Bevan DR. Newer neuromuscular blocking agents. Pharmacol Toxicol 1994;74:3-9. [Crossref] [PubMed]
- Kopman AF, Klewicka MM, Kopman DJ, et al. Molar potency is predictive of the speed of onset of neuromuscular block for agents of intermediate, short, and ultrashort duration. Anesthesiology 1999;90:425-31. [Crossref] [PubMed]
- Pühringer FK, Heier T, Dodgson M, et al. Double-blind comparison of the variability in spontaneous recovery of cisatracurium- and vecuronium-induced neuromuscular block in adult and elderly patients. Acta Anaesthesiol Scand 2002;46:364-71. [Crossref] [PubMed]
- Srivastava A, Hunter JM. Reversal of neuromuscular block. Br J Anaesth 2009;103:115-29. [Crossref] [PubMed]
- Bom A, Hope F, Rutherford S, et al. Preclinical pharmacology of sugammadex. J Crit Care 2009;24:29-35. [Crossref] [PubMed]
- Schaller SJ, Fink H. Sugammadex as a reversal agent for neuromuscular block: an evidence-based review. Core Evid 2013;8:57-67. [PubMed]
- Rex C, Bergner UA, Pühringer FK. Sugammadex: a selective relaxant-binding agent providing rapid reversal. Curr Opin Anaesthesiol 2010;23:461-5. [Crossref] [PubMed]
- Akha AS, Rosa J 3rd, Jahr JS, et al. Sugammadex: cyclodextrins, development of selective binding agents, pharmacology, clinical development, and future directions. Anesthesiol Clin 2010;28:691-708. [Crossref] [PubMed]
- Thilen SR, Weigel WA, Todd MM, et al. 2023 American Society of Anesthesiologists Practice Guidelines for Monitoring and Antagonism of Neuromuscular Blockade: A Report by the American Society of Anesthesiologists Task Force on Neuromuscular Blockade. Anesthesiology 2023;138:13-41. [Crossref] [PubMed]
- Bartkowski RR. Incomplete reversal of pancuronium neuromuscular blockade by neostigmine, pyridostigmine, and edrophonium. Anesth Analg 1987;66:594-8. [Crossref] [PubMed]
- Goldhill DR, Wainwright AP, Stuart CS, et al. Neostigmine after spontaneous recovery from neuromuscular blockade. Effect on depth of blockade monitored with train-of-four and tetanic stimuli. Anaesthesia 1989;44:293-9. [Crossref] [PubMed]
- Caldwell JE. Reversal of residual neuromuscular block with neostigmine at one to four hours after a single intubating dose of vecuronium. Anesth Analg 1995;80:1168-74. [PubMed]
- Kent NB, Liang SS, Phillips S, et al. Therapeutic doses of neostigmine, depolarising neuromuscular blockade and muscle weakness in awake volunteers: a double-blind, placebo-controlled, randomised volunteer study. Anaesthesia 2018;73:1079-89. [Crossref] [PubMed]
- Eikermann M, Zaremba S, Malhotra A, et al. Neostigmine but not sugammadex impairs upper airway dilator muscle activity and breathing. Br J Anaesth 2008;101:344-9. [Crossref] [PubMed]
- Herbstreit F, Zigrahn D, Ochterbeck C, et al. Neostigmine/glycopyrrolate administered after recovery from neuromuscular block increases upper airway collapsibility by decreasing genioglossus muscle activity in response to negative pharyngeal pressure. Anesthesiology 2010;113:1280-8. [Crossref] [PubMed]
- Jonsson M, Gurley D, Dabrowski M, et al. Distinct pharmacologic properties of neuromuscular blocking agents on human neuronal nicotinic acetylcholine receptors: a possible explanation for the train-of-four fade. Anesthesiology 2006;105:521-33. [Crossref] [PubMed]
- Jonsson Fagerlund M, Dabrowski M, Eriksson LI. Pharmacological characteristics of the inhibition of nondepolarizing neuromuscular blocking agents at human adult muscle nicotinic acetylcholine receptor. Anesthesiology 2009;110:1244-52. [Crossref] [PubMed]
- Schaller SJ, Fink H, Ulm K, et al. Sugammadex and neostigmine dose-finding study for reversal of shallow residual neuromuscular block. Anesthesiology 2010;113:1054-60. [Crossref] [PubMed]
- Harper NJ, Wallace M, Hall IA. Optimum dose of neostigmine at two levels of atracurium-induced neuromuscular block. Br J Anaesth 1994;72:82-5. [Crossref] [PubMed]
- Fuchs-Buder T, Meistelman C, Alla F, et al. Antagonism of low degrees of atracurium-induced neuromuscular blockade: dose-effect relationship for neostigmine. Anesthesiology 2010;112:34-40. [Crossref] [PubMed]
- Sacan O, White PF, Tufanogullari B, et al. Sugammadex reversal of rocuronium-induced neuromuscular blockade: a comparison with neostigmine-glycopyrrolate and edrophonium-atropine. Anesth Analg 2007;104:569-74. [Crossref] [PubMed]
- Bowdle TA, Haththotuwegama KJ, Jelacic S, et al. A Dose-finding Study of Sugammadex for Reversal of Rocuronium in Cardiac Surgery Patients and Postoperative Monitoring for Recurrent Paralysis. Anesthesiology 2023;139:6-15. [Crossref] [PubMed]
- Eleveld DJ, Kuizenga K, Proost JH, et al. A temporary decrease in twitch response during reversal of rocuronium-induced muscle relaxation with a small dose of sugammadex. Anesth Analg 2007;104:582-4. [Crossref] [PubMed]
- Ploeger BA, Smeets J, Strougo A, et al. Pharmacokinetic-pharmacodynamic model for the reversal of neuromuscular blockade by sugammadex. Anesthesiology 2009;110:95-105. [Crossref] [PubMed]
- Nemes R, Fülesdi B, Pongrácz A, et al. Impact of reversal strategies on the incidence of postoperative residual paralysis after rocuronium relaxation without neuromuscular monitoring: A partially randomised placebo controlled trial. Eur J Anaesthesiol 2017;34:609-16. [Crossref] [PubMed]
- Kotake Y, Ochiai R, Suzuki T, et al. Reversal with sugammadex in the absence of monitoring did not preclude residual neuromuscular block. Anesth Analg 2013;117:345-51. [Crossref] [PubMed]
- Kisor DF, Schmith VD, Wargin WA, et al. Importance of the organ-independent elimination of cisatracurium. Anesth Analg 1996;83:1065-71. [Crossref] [PubMed]
- Lynam DP, Cronnelly R, Castagnoli KP, et al. The pharmacodynamics and pharmacokinetics of vecuronium in patients anesthetized with isoflurane with normal renal function or with renal failure. Anesthesiology 1988;69:227-31. [Crossref] [PubMed]
- Staals LM, Snoeck MM, Driessen JJ, et al. Reduced clearance of rocuronium and sugammadex in patients with severe to end-stage renal failure: a pharmacokinetic study. Br J Anaesth 2010;104:31-9. [Crossref] [PubMed]
- Lien CA, Matteo RS, Ornstein E, et al. Distribution, elimination, and action of vecuronium in the elderly. Anesth Analg 1991;73:39-42. [Crossref] [PubMed]
- Dhonneur G, Rebaine C, Slavov V, et al. Neostigmine reversal of vecuronium neuromuscular block and the influence of renal failure. Anesth Analg 1996;82:134-8. [PubMed]
- Plaud B, Meretoja O, Hofmockel R, et al. Reversal of rocuronium-induced neuromuscular blockade with sugammadex in pediatric and adult surgical patients. Anesthesiology 2009;110:284-94. [Crossref] [PubMed]
- Peeters P, Passier P, Smeets J, et al. Sugammadex is cleared rapidly and primarily unchanged via renal excretion. Biopharm Drug Dispos 2011;32:159-67. [Crossref] [PubMed]
- Adams DR, Tollinche LE, Yeoh CB, et al. Short-term safety and effectiveness of sugammadex for surgical patients with end-stage renal disease: a two-centre retrospective study. Anaesthesia 2020;75:348-52. [Crossref] [PubMed]
- Staals LM, Snoeck MM, Driessen JJ, et al. Multicentre, parallel-group, comparative trial evaluating the efficacy and safety of sugammadex in patients with end-stage renal failure or normal renal function. Br J Anaesth 2008;101:492-7. [Crossref] [PubMed]
- Cammu G, Van Vlem B, van den Heuvel M, et al. Dialysability of sugammadex and its complex with rocuronium in intensive care patients with severe renal impairment. Br J Anaesth 2012;109:382-90. [Crossref] [PubMed]
- Baraka A, Wakid N, Mansour R, et al. Effect of neostigmine and pyridostigmine on the plasma cholinesterase activity. Br J Anaesth 1981;53:849-51. [Crossref] [PubMed]
- Lien CA, Belmont MR, Wray Roth DL, et al. Pharmacodynamics and the plasma concentration of mivacurium during spontaneous recovery and neostigmine-facilitated recovery. Anesthesiology 1999;91:119-26. [Crossref] [PubMed]
- Brown TC. Tricyclic antidepressant overdosage: experimental studies on the management of circulatory complications. Clin Toxicol 1976;9:255-72. [Crossref] [PubMed]
- Available online: https://www.merck.com/product/usa/pi_circulars/b/bridion/bridion_pi.pdf
- Richardson MG, Raymond BL. Sugammadex Administration in Pregnant Women and in Women of Reproductive Potential: A Narrative Review. Anesth Analg 2020;130:1628-37. [Crossref] [PubMed]
- Et T, Topal A, Erol A, et al. Effect of sugammadex on progesterone level in pregnant rats: 9AP1-6. Eur J Anaesthesiol 2013;30:141. [Crossref]
- Mushambi MC, Kinsella SM, Popat M, et al. Obstetric Anaesthetists' Association and Difficult Airway Society guidelines for the management of difficult and failed tracheal intubation in obstetrics. Anaesthesia 2015;70:1286-306. [Crossref] [PubMed]
- Jahr JS, Miller JE, Hiruma J, et al. Sugammadex: A Scientific Review Including Safety and Efficacy, Update on Regulatory Issues, and Clinical Use in Europe. Am J Ther 2015;22:288-97. [Crossref] [PubMed]
- Munro A, McKeen D, Coolen J. Maternal respiratory distress and successful reversal with sugammadex during intrauterine transfusion with fetal paralysis. Int J Obstet Anesth 2019;39:129-31. [Crossref] [PubMed]
- Viby-Mogensen J, Jørgensen BC, Ording H. Residual curarization in the recovery room. Anesthesiology 1979;50:539-41. [Crossref] [PubMed]
- Bevan DR, Smith CE, Donati F. Postoperative neuromuscular blockade: a comparison between atracurium, vecuronium, and pancuronium. Anesthesiology 1988;69:272-6. [Crossref] [PubMed]
- Debaene B, Plaud B, Dilly MP, et al. Residual paralysis in the PACU after a single intubating dose of nondepolarizing muscle relaxant with an intermediate duration of action. Anesthesiology 2003;98:1042-8. [Crossref] [PubMed]
- Murphy GS, Szokol JW, Marymont JH, et al. Residual neuromuscular blockade and critical respiratory events in the postanesthesia care unit. Anesth Analg 2008;107:130-7. [Crossref] [PubMed]
- Butterly A, Bittner EA, George E, et al. Postoperative residual curarization from intermediate-acting neuromuscular blocking agents delays recovery room discharge. Br J Anaesth 2010;105:304-9. [Crossref] [PubMed]
- Berg H, Roed J, Viby-Mogensen J, et al. Residual neuromuscular block is a risk factor for postoperative pulmonary complications. A prospective, randomised, and blinded study of postoperative pulmonary complications after atracurium, vecuronium and pancuronium. Acta Anaesthesiol Scand 1997;41:1095-103. [Crossref] [PubMed]
- Murphy GS, Szokol JW, Avram MJ, et al. Residual Neuromuscular Block in the Elderly: Incidence and Clinical Implications. Anesthesiology 2015;123:1322-36. [Crossref] [PubMed]
- Todd MM, Hindman BJ, King BJ. The implementation of quantitative electromyographic neuromuscular monitoring in an academic anesthesia department. Anesth Analg 2014;119:323-31. [Crossref] [PubMed]
- Broens SJL, Boon M, Martini CH, et al. Reversal of Partial Neuromuscular Block and the Ventilatory Response to Hypoxia: A Randomized Controlled Trial in Healthy Volunteers. Anesthesiology 2019;131:467-76. [Crossref] [PubMed]
- Torbic H, Krishnan S, Harnegie MP, et al. Neuromuscular Blocking Agents for ARDS: A Systematic Review and Meta-Analysis. Respir Care 2021;66:120-8. [Crossref] [PubMed]
- Steingrub JS, Lagu T, Rothberg MB, et al. Treatment with neuromuscular blocking agents and the risk of in-hospital mortality among mechanically ventilated patients with severe sepsis. Crit Care Med 2014;42:90-6. [Crossref] [PubMed]
- Harrison GG. Death attributable to anaesthesia. A 10-year survey (1967--1976). Br J Anaesth 1978;50:1041-6. [Crossref] [PubMed]
- Lunn JN, Hunter AR, Scott DB. Anaesthesia-related surgical mortality. Anaesthesia 1983;38:1090-6. [Crossref] [PubMed]
- BEECHER HK. TODD DP. A study of the deaths associated with anesthesia and surgery: based on a study of 599, 548 anesthesias in ten institutions 1948-1952, inclusive. Ann Surg 1954;140:2-35. [PubMed]
- Braz LG, Braz DG, Cruz DS, et al. Mortality in anesthesia: a systematic review. Clinics (Sao Paulo) 2009;64:999-1006. [Crossref] [PubMed]
- Bronsert MR, Henderson WG, Monk TG, et al. Intermediate-Acting Nondepolarizing Neuromuscular Blocking Agents and Risk of Postoperative 30-Day Morbidity and Mortality, and Long-term Survival. Anesth Analg 2017;124:1476-83. [Crossref] [PubMed]
- Thevathasan T, Shih SL, Safavi KC, et al. Association between intraoperative non-depolarising neuromuscular blocking agent dose and 30-day readmission after abdominal surgery. Br J Anaesth 2017;119:595-605. [Crossref] [PubMed]
- McLean DJ, Diaz-Gil D, Farhan HN, et al. Dose-dependent Association between Intermediate-acting Neuromuscular-blocking Agents and Postoperative Respiratory Complications. Anesthesiology 2015;122:1201-13. [Crossref] [PubMed]
- Drachman DB, Adams RN, Josifek LF, et al. Functional activities of autoantibodies to acetylcholine receptors and the clinical severity of myasthenia gravis. N Engl J Med 1982;307:769-75. [Crossref] [PubMed]
- Paton WD, Waud DR. The margin of safety of neuromuscular transmission. J Physiol 1967;191:59-90. [Crossref] [PubMed]
- Campkin NT, Hood JR, Fauvel NJ, et al. The effect of residual receptor occupancy on sensitivity to repeated vecuronium. Anaesthesia 1993;48:572-4. [Crossref] [PubMed]
- Fisher DM, Fahey MR, Cronnelly R, et al. Potency determination for vecuronium (ORG NC45): comparison of cumulative and single-dose techniques. Anesthesiology 1982;57:309-10. [Crossref] [PubMed]
- Wick S, Muenster T, Schmidt J, et al. Onset and duration of rocuronium-induced neuromuscular blockade in patients with Duchenne muscular dystrophy. Anesthesiology 2005;102:915-9. [Crossref] [PubMed]
- Ririe DG, Shapiro F, Sethna NF. The response of patients with Duchenne's muscular dystrophy to neuromuscular blockade with vecuronium. Anesthesiology 1998;88:351-4. [Crossref] [PubMed]
- Tobias JD. Current evidence for the use of sugammadex in children. Paediatr Anaesth 2017;27:118-25. [Crossref] [PubMed]
- de Boer HD, van Esmond J, Booij LH, et al. Reversal of rocuronium-induced profound neuromuscular block by sugammadex in Duchenne muscular dystrophy. Paediatr Anaesth 2009;19:1226-8. [Crossref] [PubMed]
- Kim JE, Chun HR. Rocuronium-induced neuromuscular block and sugammadex in pediatric patient with duchenne muscular dystrophy: A case Report. Medicine (Baltimore) 2017;96:e6456. [Crossref] [PubMed]
- Gurunathan U, Kunju SM, Stanton LML. Use of sugammadex in patients with neuromuscular disorders: a systematic review of case reports. BMC Anesthesiol 2019;19:213. [Crossref] [PubMed]
- Lebowitz PW, Ramsey FM, Savarese JJ, et al. Potentiation of neuromuscular blockade in man produced by combinations of pancuronium and metocurine or pancuronium and d-tubocurarine. Anesth Analg 1980;59:604-9. [Crossref] [PubMed]
- Eikermann M, Gerwig M, Hasselmann C, et al. Impaired neuromuscular transmission after recovery of the train-of-four ratio. Acta Anaesthesiol Scand 2007;51:226-34. [Crossref] [PubMed]
- Kirmeier E, Eriksson LI, Lewald H, et al. Post-anaesthesia pulmonary complications after use of muscle relaxants (POPULAR): a multicentre, prospective observational study. Lancet Respir Med 2019;7:129-40. [Crossref] [PubMed]
- Blobner M, Hunter JM, Meistelman C, et al. Use of a train-of-four ratio of 0.95 versus 0.9 for tracheal extubation: an exploratory analysis of POPULAR data. Br J Anaesth 2020;124:63-72. [Crossref] [PubMed]