A HIF-2α-dependent KMT2E-AS1/KMT2E axis orchestrates endothelial epigenetic and metabolic dysfunction in pulmonary hypertension
Pulmonary hypertension (PH) encompasses an heterogenous group of disorders, all hemodynamically defined by a mean pulmonary artery (PA) pressure >20 mmHg and characterized by endothelial injury and dysfunction, excessive vasoconstriction, and abnormal vascular remodeling of small PAs (1,2). These changes cause an increase in pulmonary vascular resistance, which ultimately results in right ventricular failure (2). Patients with PH are classified into five groups based upon etiology and mechanism. Although uncommon compared to other forms of PH, pulmonary arterial hypertension (PAH; group 1 PH) is the most severe and best studied subtype (3). Additionally, PH frequently complicates the clinical course of patients with lung diseases and/or hypoxemia, such as chronic obstructive pulmonary disease and interstitial lung disease (group 3 PH). This comorbidity exacerbates the already elevated morbidity and mortality rates associated with these conditions (4). Despite intense research endeavors and Food and Drug Administration (FDA)-approved drugs that mainly target pulmonary vasoconstriction (2,3,5), the prognosis of patients with group 1 and group 3 PH remains poor, emphasizing the urgent need for a better understanding of the molecular mechanisms leading to vascular remodeling.
Activation of hypoxia-inducible factors (HIFs) is a hallmark of various subtypes of PH and a driving force in reprogramming the cellular metabolic and epigenetic landscape of PA resident cells (6,7). Among the different oxygen-sensitive alpha subunits, HIF-2α has emerged to play a predominant role in mediating PA endothelial cell (PAEC) proliferation contributing to the development of occlusive intimal/neointimal lesions. Notably, inactivation of HIF-2α targeted to the pulmonary endothelium has been shown to confer protection against chronic hypoxia-induced PH to a greater extent compared to mice with pulmonary endothelial deletion of HIF-1α (8,9). Additionally, pharmacological inhibition of HIF-2α was reported to improve pulmonary vascular remodeling and hemodynamics in several preclinical models of established PH (10). Although HIF-2α is a central protagonist of pulmonary vascular remodeling, the molecular mechanisms mediating its effects and accounting for its upregulation in PAECs remain poorly characterized. Similarly, although the implication of epigenetic changes, such as histone modifications and non-coding RNAs, is gaining increasing attention at the forefront of PH research field, they are still at the beginning.
In their recent article published in Science Translational Medicine (11) (summarized in Figure 1), Pr. Chan and colleagues provide comprehensive and compelling insights into the intricate relationship between hypoxia and epigenetic events, and its critical impact on metabolic reprogramming that favors PAEC proliferation. As a starting point, the authors identified a long non-coding RNA (lncRNA) called E22, which was upregulated in the lungs of mice in which PH was induced by the combined exposure to hypoxia and a VEGF receptor antagonist. Interest in this lncRNA was piqued by its conserved syntenic location, positioned head-to-head with the histone lysine N-methyltransferase 2E (KMT2E) gene and its partially conserved sequence with human KMT2E-AS1.
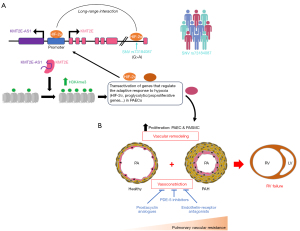
Subsequent experiments showed that the increase in E22, KMT2E-AS1, and KMT2E transcripts was mainly detected in PAEC of remodeled PAs from both group 1 and group 3 PH patients and PH animal models with preferential nuclear localization. Furthermore, using a bidirectional approach in cultured endothelial cells, HIF-2α was identified as a key regulator of the lncRNA-KMT2E pair. Indeed, depletion of HIF-2α was found to abolish the up-regulation of KMT2E-AS1 and KMT2E transcripts under hypoxia, whereas forced expression of a constitutively active form of HIF-2α in normoxic cells led to opposite effects.
Since its nuclear-enriched localization implies a role in chromatin remodeling and transcriptional regulation, and the KMT2E-AS1 gene locus is adjacent to the KMT2E gene, research efforts were made to elucidate a possible connection between them. In an elegant series of experiments, the authors found that KMT2E-AS1 complexes with and stabilizes the KMTE2 protein, leading to increased trimethylation of histone H3 lysine 4 (H3K4me3; a mark of transcriptional activity). Furthermore, reduced expression of HIF-2α was observed in hypoxic PAECs depleted of either KMT2E-AS1 or KMT2E using small interfering RNAs. Further mechanistic studies demonstrated that KMT2E-AS1 and KMT2E indirectly promote HIF-2α transcription, translation, and stability, giving rise to a positive feedback loop (Figure 1).
To gain insight into the function of this lncRNA, genome-wide RNA sequencing was performed in KMT2E-AS1- and KMT2E-knockdown PAECs exposed to hypoxia. It was shown that their depletion significantly reversed hypoxia-induced transcriptomic changes, with regulated genes being enriched in biological processes related to metabolism and hypoxia. As expected, several genes upregulated by hypoxia and downregulated upon knockdown of KMT2E-AS1 or KMT2E exhibit more H3K4me3 marks in their promoter regions under hypoxic conditions. Functional analyses revealed that KMT2E-AS1 promotes a shift from oxidative to glycolytic metabolism, allowing PAECs to adapt to hypoxia and proliferate. Having shown that a 550-bp region in the 5' end of E22 carries similar activity as KMT2E-AS1, the authors next investigated the importance of E22 to promote PH development and progression in vivo. To this end, lung-targeted adeno-associated virus 6 (AAV6)-mediated E22 overexpression and generation of an E22 loss-of-function mouse model using clustered regularly interspaced short palindromic repeats (CRISPR)/CRISPR-associated protein 9 (Cas9)-mediated genome engineering were pursued. Overexpression of E22 was found to worsen PH in mice exposed to chronic hypoxia, as evidenced by an increased proportion of fully muscularized vessels and elevated right ventricular systolic pressure (RVSP). Chronic hypoxia-induced right ventricular hypertrophy was further augmented in E22-overexpressing mice compared to AAV6-green fluorescent protein (GFP)-treated mice. In contrast, mice harboring the 550-bp deletion in E22 exhibited reduced vascular muscularization and RVSP when exposed to chronic hypoxia compared to their wild-type counterparts. Similar results were obtained when mutant mice were crossed with transgenic mice overexpressing interleukin-6 under chronic hypoxic conditions to induce more robust PH (11). Furthermore, pharmacological inhibition of histone methyltransferases (HMTs) that catalyze H3K4 and H3K9 trimethylation using chaetocin significantly improved established PH in rats subjected to Sugen/hypoxia, which is consistent with a recent study showing that blockade of the Euchromatic lysine methyltransferases 1 and 2 ameliorates pulmonary hemodynamics in PH models (12). Although the study has the merit of demonstrating the protective effect of E22 loss-of-function in multiple PH animal models, it would have been interesting, albeit tedious, to assess structural and functional changes after crossing E22 knockout mice with mice harboring endothelial/hematopoietic-specific deletion of prolyl 4-hydroxylase, for which HIF-2α-dependent and irreversible obliterative vascular remodeling has been reported (13,14).
In addition to presenting convincing molecular and genetic in vitro and in vivo approaches supporting a causal relationship between the activation of the HIF-2α/KMT2E-AS1/KMT2E axis and the pro-proliferative phenotype of PAECs in PH, the authors found a significant association for a single nucleotide variant (SNV; rs73184087, G>A) with predicted HIF-2α binding located at a KMT2E intronic site 75 kb downstream of the lncRNA-KMT2E shared promoter with an increased risk of developing PAH in several independent cohorts (11). Molecular and functional assays demonstrated a specific interaction between the SNV and the promoter and revealed that the G allele of rs73184087 has a higher binding affinity for HIF-2α and that this increased binding is associated with increased expression of the lncRNA-KMT2E pair (11). Beyond offering mechanistic explanation underlying the enrichment of SNV rs73184087 (G) allele in PAH patients, these results suggest that this SNV may help identify individuals at increased risk for PAH who may benefit from more intensive surveillance.
Often referred to as “genomic dark matter”, over 98% of our genome that does not encode for protein has been found to be rich in biologically active elements, including lncRNAs. It has become clear that a better understanding of PH pathogenesis requires examination of its lncRNAs. However, unraveling their involvement in normal and disease states is hampered by several factors, including a low basal expression of most of them, a poor sequence conservation among species, and most often, the implementation of more technically challenging approaches to capture their capricious nuclear functions. The work carried out by Pr. Chan and coworkers represents an important step in this direction by demonstrating the functional and translational importance of KMT2E-AS1, with no previously assigned function, in controlling pathological vascular remodeling in PH. In this regard, this work adds to the rare studies exploring the role of lncRNA landscape in human PH (15-17).
In summary, the results described by Chan’s group have significant implications for our understanding of the HIF-2α/KMT2E-AS1/KMT2E feed-forward loop in PH pathogenesis. In addition to emphasizing lncRNA-targeted therapy as a potential avenue to combat vascular remodeling in PH, the convincing data presented in this article further support a key role for HIF-2α in orchestrating the metabolic reprogramming of PAECs in PAH. Although transcription factors have traditionally been considered difficult to target, the recent U.S. FDA approval of belzutifan (18), a highly specific and well tolerated HIF-2α inhibitor for the treatment of advanced kidney cancer, may provide a meaningful new treatment option for certain PAH patients. On another note, the study leaves open questions that are potentially important for precisely understanding the role of KMT2E. Indeed, although it belongs to the KMT2 family of lysine methyltransferases responsible for methylating H3K4, KMT2E appears to lack intrinsic HMT activity (19,20), suggesting that its effects on H3K4 are indirect. Moreover, future studies should address the possible involvement of KMT2E-AS1 as guide molecule to regulate transcription by targeting KMT2E to its genomic targets (21) and its role in pulmonary adventitial fibroblasts in which increased expression of the lncRNA-KMT2E pair was also reported. Finally, a potential role of KMT2E-AS1/KMT2E in pathological right ventricular remodeling needs to be investigated.
Acknowledgments
Funding: None.
Footnote
Provenance and Peer Review: This article was commissioned by the editorial office, Annals of Translational Medicine. The article has undergone external peer review.
Peer Review File: Available at https://atm.amegroups.com/article/view/10.21037/atm-24-54/prf
Conflicts of Interest: All authors have completed the ICMJE uniform disclosure form (available at https://atm.amegroups.com/article/view/10.21037/atm-24-54/coif). The authors have no conflicts of interest to declare.
Ethical Statement: The authors are accountable for all aspects of the work in ensuring that questions related to the accuracy or integrity of any part of the work are appropriately investigated and resolved.
Open Access Statement: This is an Open Access article distributed in accordance with the Creative Commons Attribution-NonCommercial-NoDerivs 4.0 International License (CC BY-NC-ND 4.0), which permits the non-commercial replication and distribution of the article with the strict proviso that no changes or edits are made and the original work is properly cited (including links to both the formal publication through the relevant DOI and the license). See: https://creativecommons.org/licenses/by-nc-nd/4.0/.
References
- Simonneau G, Montani D, Celermajer DS, et al. Haemodynamic definitions and updated clinical classification of pulmonary hypertension. Eur Respir J 2019;53:1801913. [Crossref] [PubMed]
- Humbert M, Kovacs G, Hoeper MM, et al. 2022 ESC/ERS Guidelines for the diagnosis and treatment of pulmonary hypertension. Eur Heart J 2022;43:3618-731. [Crossref] [PubMed]
- Humbert M, Sitbon O, Guignabert C, et al. Treatment of pulmonary arterial hypertension: recent progress and a look to the future. Lancet Respir Med 2023;11:804-19. [Crossref] [PubMed]
- Mocumbi A, Humbert M, Saxena A, et al. Pulmonary hypertension. Nat Rev Dis Primers 2024;10:1. [Crossref] [PubMed]
- Waxman A, Restrepo-Jaramillo R, Thenappan T, et al. Inhaled Treprostinil in Pulmonary Hypertension Due to Interstitial Lung Disease. N Engl J Med 2021;384:325-34. [Crossref] [PubMed]
- Pullamsetti SS, Mamazhakypov A, Weissmann N, et al. Hypoxia-inducible factor signaling in pulmonary hypertension. J Clin Invest 2020;130:5638-51. [Crossref] [PubMed]
- Bryant AJ, Carrick RP, McConaha ME, et al. Endothelial HIF signaling regulates pulmonary fibrosis-associated pulmonary hypertension. Am J Physiol Lung Cell Mol Physiol 2016;310:L249-62. [Crossref] [PubMed]
- Cowburn AS, Crosby A, Macias D, et al. HIF2α-arginase axis is essential for the development of pulmonary hypertension. Proc Natl Acad Sci U S A 2016;113:8801-6. [Crossref] [PubMed]
- Tang H, Babicheva A, McDermott KM, et al. Endothelial HIF-2α contributes to severe pulmonary hypertension due to endothelial-to-mesenchymal transition. Am J Physiol Lung Cell Mol Physiol. 2018;314:L256-75. [PubMed]
- Dai Z, Zhu MM, Peng Y, et al. Therapeutic Targeting of Vascular Remodeling and Right Heart Failure in Pulmonary Arterial Hypertension with a HIF-2α Inhibitor. Am J Respir Crit Care Med 2018;198:1423-34. [Crossref] [PubMed]
- Tai YY, Yu Q, Tang Y, et al. Allele-specific control of rodent and human lncRNA KMT2E-AS1 promotes hypoxic endothelial pathology in pulmonary hypertension. Sci Transl Med 2024;16:eadd2029. [Crossref] [PubMed]
- Awada C, Bourgeois A, Lemay SE, et al. G9a/GLP Targeting Ameliorates Pulmonary Vascular Remodeling in Pulmonary Arterial Hypertension. Am J Respir Cell Mol Biol 2023;68:537-50. [Crossref] [PubMed]
- Kapitsinou PP, Rajendran G, Astleford L, et al. The Endothelial Prolyl-4-Hydroxylase Domain 2/Hypoxia-Inducible Factor 2 Axis Regulates Pulmonary Artery Pressure in Mice. Mol Cell Biol 2016;36:1584-94. [Crossref] [PubMed]
- Dai Z, Li M, Wharton J, et al. Prolyl-4 Hydroxylase 2 (PHD2) Deficiency in Endothelial Cells and Hematopoietic Cells Induces Obliterative Vascular Remodeling and Severe Pulmonary Arterial Hypertension in Mice and Humans Through Hypoxia-Inducible Factor-2α. Circulation 2016;133:2447-58. [Crossref] [PubMed]
- Zehendner CM, Valasarajan C, Werner A, et al. Long Noncoding RNA TYKRIL Plays a Role in Pulmonary Hypertension via the p53-mediated Regulation of PDGFRβ. Am J Respir Crit Care Med 2020;202:1445-57. [Crossref] [PubMed]
- Omura J, Habbout K, Shimauchi T, et al. Identification of Long Noncoding RNA H19 as a New Biomarker and Therapeutic Target in Right Ventricular Failure in Pulmonary Arterial Hypertension. Circulation 2020;142:1464-84. [Crossref] [PubMed]
- Wang S, Cao W, Gao S, et al. TUG1 Regulates Pulmonary Arterial Smooth Muscle Cell Proliferation in Pulmonary Arterial Hypertension. Can J Cardiol 2019;35:1534-45. [Crossref] [PubMed]
- Expanding "Practice-Changing" Belzutifan's Reach in RCC. Cancer Discov 2023;13:OF5. [Crossref]
- Sebastian S, Sreenivas P, Sambasivan R, et al. MLL5, a trithorax homolog, indirectly regulates H3K4 methylation, represses cyclin A2 expression, and promotes myogenic differentiation. Proc Natl Acad Sci U S A 2009;106:4719-24. [Crossref] [PubMed]
- Zhang X, Novera W, Zhang Y, et al. MLL5 (KMT2E): structure, function, and clinical relevance. Cell Mol Life Sci 2017;74:2333-44. [Crossref] [PubMed]
- Sun Q, Hao Q, Prasanth KV. Nuclear Long Noncoding RNAs: Key Regulators of Gene Expression. Trends Genet 2018;34:142-57. [Crossref] [PubMed]