Multiple sclerosis: the NLRP3 inflammasome, gasdermin D, and therapeutics
Introduction
Multiple sclerosis (MS) is characterized by both inflammation and immune-mediated neurodegeneration within the central nervous system (CNS). Inflammation is not merely a consequence but a critical driving force in the disease’s pathogenesis (1). Magnetic resonance imaging (MRI) reveals characteristic lesions or plaques in the CNS that indicate demyelination and inflammation, supporting the diagnosis of MS (2). Plaques are regions of nerves where the myelin has been removed, preventing them from conducting the electrical signals necessary for proper function and are associated with inflammation and the accumulation of inflammation-related cells (3).
The cause of MS-related neurodegeneration is in question. Still, evidence supports genetic and environmental causes, such as low vitamin D serum levels, smoking, obesity, and Epstein-Barr virus infection (EBV) (4). Evidence that EBV, a herpesvirus, may be responsible for triggering the autoimmune response and lead to demyelination (5). The study utilized a large sample size over the course of 20 years and found that the risk of MS increased 32-fold after infection with EBV. Additionally, connections have been made between EBV reactivation and the nucleotide-binding oligomerization domain (NOD)-, leucine-rich repeat (LRR)-, and pyrin domains (PYD)-containing protein 3 (NLRP3) inflammasome, a possible initiator of the MS-associated inflammatory response (6).
NLRP3 is the sensor component of the inflammasome complex that responds to stimuli threatening cell homeostasis. Expression is high in macrophages and dendritic cells, and its activation in these cells leads to the production of pro-inflammatory cytokines, such as interleukin-1β (IL-1β) and interleukin-18 (IL-18), which contribute to neuroinflammation and tissue damage in MS (7-9). These cytokines can be secreted from the cell or released via pyroptotic cell death and can be detected within exosomes in the cerebrospinal fluid (CSF) of MS patients (10,11). The activation of the NLRP3 inflammasome can be triggered by a wide range of stimuli, including microbial toxins, adenosine triphosphate (ATP), crystalline substances like uric acid crystals, and environmental irritants (12-14). Upon activation, NLRP3 recruits apoptosis-associated speck-like protein containing a caspase-recruitment domain (ASC), which recruits pro-caspase 1. The proximity of two pro-caspase-1 leads to their cleavage into active caspase-1, an enzyme that plays a key role in the processing and secretion of pro-inflammatory cytokines, particularly IL-1β and IL-18 (13,15,16). These cytokines are crucial for initiating and propagating inflammatory responses, attracting immune cells to the site of infection or damage, and modulating the immune reaction.
Gasdermin D (GsdmD)-mediated pyroptosis results in a lytic cell death that releases cellular content into the extracellular space (17-19). GsdmD consists of two domains: an N-terminal domain responsible for pore formation and a C-terminal repressor domain. Inactive GsdmD is kept in a closed conformation through the interaction between these two domains. Upon activation, typically by inflammatory caspase-1, caspase-4, caspase-5 in humans, and caspase-11 in mice, GsdmD is cleaved. This cleavage separates the N-terminal pore-forming domain from the C-terminal domain, allowing the N-terminal fragment to oligomerize and insert into the plasma membrane.
Methodology
This literature review systematically examines current knowledge and recent advancements concerning the NLRP3 protein complex, focusing on its structure, function, and role in MS. The primary objective of this review is to synthesize research findings related to the NLRP3 inflammasome and GsdmD, specifically its molecular structure, biological functions, and implications in the pathogenesis of MS. A comprehensive search was conducted in PubMed, Web of Science, and Scopus, covering publications up to March 2024. Search terms used were “NLRP3”, “inflammasomes”, “Multiple Sclerosis”, and “Gasdermin D”, along with their synonyms and relevant combinations, employing Boolean operators to refine the search. Peer-reviewed articles in English detailing the structure, function, or disease relevance of the NLRP3 protein complex, including original research articles, reviews, and meta-analyses were included. Non-peer-reviewed articles, conference abstracts, and studies not focusing on the NLRP3 protein complex or not providing specific insights into its structure, function, or disease relevance were excluded.
NLRP3 inflammasome
NLRP3: function
NLRP3 serves as the signal detector that initiates inflammasome assembly (20). Specifically, it is a pattern recognition receptor (PRR), which detects threats to the host and regulates immune responses to these threats (21). As a PRR, NLRP3 is activated by several signals, making it probable that the signal detection occurs not through direct binding but rather through some other common factor. Two specific activators are pathogen-associated molecular patterns (PAMPs) and damage-associated molecular patterns (DAMPs) (22). PAMPs are present when a foreign molecule is detected, while DAMPs are present when the cell itself is damaged (23).
NLRP3: domains
The NLRP3 protein consists of 3 domains: an amino-terminal PYD, a central NOD, and a C-terminal LRR (24). The pyrin domain is necessary for assembling the inflammasome complex, as it interacts with the ASC protein. As its name suggests, the NOD domain facilitates NLRP3 inflammasome oligomerization, which occurs after NLRP3 is activated. LRRs were originally thought to serve as an NLRP3 autoinhibitor but have now been found unnecessary in the NLRP3 activation process (24).
NLRP3: regulation
The detection of PAMPs and DAMPs by toll-like receptor (TLR) or NOD-like receptors (NLR) initiates the upregulation of the required inflammasome components (25). NLRP3 is unique in its activation in that “cellular stress” is the only requirement for activation. PRRs must normally detect a specific molecular pattern, but this is not true for NLRP3 (26).
The detection of these signals then activates nuclear factor-κB (NF-κB), which upregulates transcription of the inflammasome components (27). Two recipients of the transcription upregulation effects are NLRP3 and interleukins, which are both necessary in increased quantities to continue the inflammasome activation process (28). When NF-κB is mutated, increased severity of inflammation, such as that associated with MS, has been reported with both the canonical and noncanonical activation pathways (27). The mutation must cause excessive or uninitiated upregulation, producing excessive cytokine release (29). NLRP3 must also undergo post-translational modifications, stabilizing it before activation (30,31).
Another important regulator of NLRP3 oligomerization is NIMA-related kinase protein 7 (NEK7), which is required for NLRP3 oligomerization (32). This interaction occurs with the NOD and LRR domains of NLRP3 and facilitates the promotion of the NLRP3-ASC complex. Recent evidence suggests that NLRP3 ubiquitination by mitochondria-associated E3-ligase, membrane-associated ring-CH-type finger 5 (MARCH5), is required for this interaction with NEK7. MARCH5 interaction with NLRP3 occurs on its NOD domain, specifically residues K324 and K430 (30).
Cryo-electron microscopy (Cryo-EM) approaches revealed that inactive NLRP3 forms a double-ring structure composed of 12–16 monomers, which act as a shield to protect its pyrin domains from the cytosol (33,34). This novel mechanism of inflammasome regulation sheds light on how the danger sensor NLRP3 is activated, challenging previous understandings of its activation process. The research also delves into the cellular location of the NLRP3 cage, suggesting that NLRP3 recruitment to the trans-Golgi network (TGN) may be crucial for NLRP3 signaling. Membrane extracts from cells at rest contained large NLRP3 oligomers consistent with the purified double-ring structure, while cytosolic NLRP3 was found to be monomeric or dimeric.
Box 1: ion changes during NLRP3 activation
During the activation of NLRP3, several adjustments to ion concentration occur. An adjustment of K+ and Ca2+ ions occurs upstream of the NLRP3 inflammasome activation (20). The first change in ion levels to note is a nearly universal decrease in intracellular K+ levels (31). The family of receptors responsible for the flow of K+ out of the cell is the P2X family. However, the P2X7 receptor plays the biggest role in ion release (32). The binding of ATP opens this ligand-gated ion channel, although relatively large amounts of ATP are required to activate this receptor (32). The diameter of the ion channel increases the longer it is open (30). This enlargement results in surrounding plasma membrane channels allowing the influx of Ca2+ ions, while the P2X7 receptor allows for the efflux of K+ ions (30,33). The efflux of K+ rather than the influx of Ca2+ is essential for NLRP3 inflammasome activation. However, the movement of both cations may help equilibrate the intra- and extra-cellular charges (34). Other P2X receptors are being investigated as possible activators of NLRP3. However, a clear mechanism is still forthcoming (35,36). Increased extracellular K+ is necessary for NLRP3 activation, although increased intracellular Ca2+ is not (31,37). It is possible to inhibit the activation of the NLRP3 inflammasome by inhibiting the efflux of K+ ions (38). Another important adjustment in ion level is the increase of intracellular Ca2+ ions accompanying the exodus of K+ ions. G-protein coupled receptors facilitate the movement of calcium ions (34). Increased intracellular Ca2+ precludes a chain of initiations that include activation of the TAK1 kinase, which helps regulate cell death by activating the NLRP3 inflammasome (39-41).
Cl– ion channels are also crucial for NLRP3 inflammasome activation. It was found that fenamate, which blocks the volume-regulated anion channels (VRACs) through which Cl– flows, is an effective inhibitor of NLRP3 inflammasome activation (42). In particular, the only ion affected by the blockage of these channels is Cl–, indicating that the disruption of this anion prevents NLRP3 activation (41). This supports the claim that efflux of this anion is essential for NLRP3 activation, as do additional research studies (43,44).
NLRP3: activation
Activation of NLRP3 is believed to be a two-step process (35). The first step is the priming of NLRP3 (24). This process is carried out by NF-κB, which begins upregulating inflammasome components once activated by TLRs (36). Upregulation to ensure component availability is necessary for inflammasome activation (37). NLRP3 is unique from other inflammasome complexes in that it can be activated by many different molecular patterns, which include, but are not limited to, viral RNA, asbestos, and extracellular ATP (38). These molecular patterns vary greatly in structure, leading to the hypothesis that it is not a physical interaction between NLRP3 and the molecular patterns that lead to activation but rather a different common signal produced by each activator (26,38).
Step two is where the NLRP3 inflammasome is assembled and where its effector stage begins. This step is activated due to several possible factors, including foreign RNA, ion flux, and lysosomal damage (24). NLRP3 oligomerization occurs through interactions between the NOD domains on two separate NLRP3 proteins (39). NLRP3 then engages with ASC via PYD-PYD interaction, thereby promoting ASC speck formation, followed by recruitment of pro-caspase 1 through interaction between the caspase recruitment domains (CARD) of caspase 1 and ASC (30). Caspase 1 activation occurs through proximity-induced self-cleavage, specifically at the linker region between p20 and p10 (40). Following activation, NEK7 interaction with NLRP3 surges and is required for ASC oligomerization and the resulting catalytic activity of caspase 1 (41).
ASC
Following NLRP3 activation and oligomerization, recruitment of the second component of the inflammasome complex must occur (25). The ASC protein is recruited via interaction between the PYD domain of NLRP3 and the PYD domain of ASC (24). ASC contains both a PYD, like that possessed by NLRP3, and a CARD (15). The purpose of ASC is to act as an adaptor that facilitates interaction between the NLRP3 inflammasome and pro-caspase 1 (15). Recognition of the molecular patterns also causes a change in the location of ASC from the mitochondria to the cytosol, where it can join the inflammasome complex (42). This movement is important because the entire inflammasome complex must be in the cytosol to activate GsdmD and the interleukins (43). ASC has also been shown to be a reliable biomarker in inflammatory CNS conditions. This part of the inflammasome is found in the serum and CSF of diagnosed stroke, traumatic brain injury, Alzheimer’s disease, and MS patients (44-47).
Caspase 1
The addition of pro-caspase 1 to the NLRP3 inflammasome marks the completed assembly of the inflammasome complex (48). Pro-caspase 1 initially associates with ASC via CARD-CARD interactions while in the inactivated form (43). Pro-caspase 1 consists of a p10 and p20 region connected by a small linker sequence that is self-cleaved into its activated form (49,50). Activation of caspase 1 marks the point at which the inflammasome is considered completely activated and can perform many functions necessary for the inflammatory response associated with NLRP3 inflammasome activation (51,52).
Caspase 1: cleaves interleukins
Caspase 1 cleaves both IL-1β and IL-18 from their “pro” forms into their activated forms (53). Upregulation of cytokines results from NF-κB activation (54). Releasing these cytokines into the extracellular space causes the final inflammatory response associated with inflammasome activation (55). Under normal conditions, this response is triggered when the cell is damaged and needs to be destroyed or when the cell has become infected and needs to be destroyed (56). When this part of the immune system malfunctions, the inflammatory response can either occur too infrequently, permitting uncontrolled proliferation of mutant cells, or too frequently, resulting in excessive inflammation and killing of otherwise healthy cells (57). We must now determine how these cytokines enter the extracellular space.
Caspase 1: cleaves GsdmD
GsdmD serves as the link between inflammasome activation and the pyroptotic response. Caspase 1 cleaves GsdmD, which splits the protein into N- and C-termini (58). The two termini are connected by a short linker region that must be cleaved at Asp275 for the N-terminus to be activated and penetrate the plasma membrane. The C-terminus folds onto the N-terminus in the full-length protein to block the portion of the N-terminus that must be exposed to the plasma membrane, thus acting as an autoinhibitory domain to pore formation.
Interleukins and MS
IL-1β
MS severity is correlated with increased IL-1β levels in the CSF (59). Upregulation of this cytokine is associated with a higher probability of relapsing-remitting MS progressing to more debilitating forms. When IL-1β levels are elevated, the IL-1 receptor antagonist is reduced, which is an interleukin that normally combats inflammation caused by IL-1β (60,61). An additional study has shown increased expression of the genes encoding IL-1β and NLRP3 in those with primary-progressive MS (PPMS), a more severe form of MS, compared to those with less severe forms and those without MS (7). In addition, elevated IL-1β levels were found in CSF samples of patients who were currently experiencing a relapse of MS (62). This cytokine can damage neurons by inducing excitatory glutamate signaling, which leads to cell death in the CNS (63). Furthermore, elevated IL-1β levels during remission can be an early sign of worsening disease progression (63). On the other hand, decreased levels of IL-1β are associated with alleviation of symptoms (64). Decreased IL-1β levels are also associated with reduced experimental autoimmune encephalomyelitis (EAE) symptoms (64). It is important to note that GsdmD-mediated cell death is not a requirement for elevated IL-1β secretion from inflammatory myeloid cells of patients with MS.
IL-18
IL-18 levels are also elevated in the CSF of MS patients (60). This leads to a chain of events that can further exacerbate MS symptoms. IL-18 is another cytokine activated by caspase 1 and released via a transmembrane pore during pyroptosis. It was also shown that those with active MS lesions have greatly increased IL-18 levels compared to those who, despite having a confirmed MS diagnosis, do not have active lesions (65). Additionally, the murine form of MS (EAE) was prevented when IL-18 antibodies were used as treatment. Rodent models with IL-18 deficiencies experienced less severe forms of EAE, although the extent of the reduction of MS severity varied (66). Interestingly, mice with knockout IL-18 receptors did not develop EAE at all (67). It was concluded that IL-18 may play a role in the active progression of MS.
It was found that NLRP3 and ASC knockout mice had milder EAE, but the reason for this was that the Th17 cells present could not enter the CNS (59). The importance of immune cells in the progression of MS is that T cells attack the myelin coating of the CNS. Oligodendrocytes repair myelin, but immune cells such as microglia harm the myelin sheath and oligodendrocytes via the reactive oxygen species (ROS) they release when activated. Microglial cells have been found in MS plaques, both new and old. IL-1β is known to damage the blood-brain barrier (BBB), thus making the CNS more susceptible to attack by the body’s other immune cells. Another consequence of IL-1β secretion is that this cytokine, in turn, activates more microglia cells, leading to increased T cell response in the CNS. IL-1β is required to form Th17 cells from naïve T cells, which ultimately regulate the body’s immune response by releasing additional cytokines and cytotoxic immune cells (68).
Box 2: studies on IL-1β
This study tested the hypothesis that glutamate was partly responsible for disease progression and neuron damage aided by inflammation (62). Cytokines, including IL-1β, were monitored to see if fluctuations in their levels affected the ability of glutamate signaling to cause further neuron damage. It was found that the glutamate signaling was more pronounced in patients actively experiencing MS symptoms and that such signaling required an increased presence of IL-1β (62).
For this investigation, 170 patients who suffered from relapsing-remitting MS (RRMS) were monitored for four years following analysis of their CSF for IL-1β levels (63). MRI and optical coherence tomography (OCT) were used to view disease progression. Their findings revealed that in patients who initially had detectable levels of IL-1β in their CSF, there were higher progression index (PI) values and higher MS Severity Scale (MSSS) values, indicating worsening of the disease (63). In contrast, patients without detectable IL-1β levels were more likely to have a non-aggressive form of MS with little to no worsening of symptoms. Additionally, there is less likelihood of damage to particular nerve areas, including retinal nerves, when IL-1β levels are initially undetectable (63). Further discussion describes potential sources of this cytokine, including NLRP3 inflammasome-related pathways (64).
GsdmD
Pyroptosis: canonical pathway
There are two main pathways by which the autoinhibitory C-terminus domain of GsdmD can be removed from the N-terminus (Figure 1). The first is the canonical pathway, which is most relevant to the discussion regarding MS. The canonical pathway is carried out after the formation and activation of the NLRP3 inflammasome, which occurs upon detection of PAMPs and DAMPs by the PRRs (69). The formation of the inflammasome complex then leads to the activation of caspase 1 from pro-caspase 1 (70). Activated caspase 1 cleaves GsdmD, releasing the N-terminus from the auto-inhibitory C-terminal region (71,72). The N-terminus then implants into the cell membrane, along with additional cleaved N-termini, collectively forming the transmembrane pore (17). It is noted that activated caspase 1 also plays a role in forming IL-18 and IL-1β from their immature/inactivated forms (73,74). It is these interleukins that, when released through the transmembrane pore, cause the inflammatory response characteristic of pyroptosis.
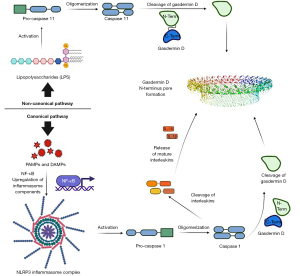
Pyroptosis: noncanonical pathway
The second pathway is the noncanonical pathway. The noncanonical pathway is activated by lipopolysaccharide (LPS), which triggers the activation of pro-caspase 4/5/11, resulting in the activated caspase (75-78). Caspase 4/5/11 oligomerizes before cleaving GsdmD into its respective domains (77). Upon release from the inhibitory C-terminus, the N-terminus can insert into the cell membrane and form a transmembrane pore with additional N-termini (18). Unlike the canonical form, this pathway does not play a role in the recruitment and activation of the cytokines necessary for the inflammatory response.
Box 3: post-pyroptosis transmembrane repair
Following pyroptosis, the cell membrane is left broken and needs repair. It is known that endosomal sorting complexes required for transport play a role in repairing damaged cell membranes (78). As with the activation of the NLRP3 inflammasome, the recruitment of an endosomal sorting complex required for transport (ESCRT) is initiated by an ion influx (79). Specifically, Ca2+ influx has been shown to initiate the recruitment of ESCRTs to begin the membrane repair process (78,80). Detergents and lasers have been used to damage the cell membranes and have observed how ESCRT responds to repair the membrane field (78). The repair begins within seconds of the Ca2+ influx, and this speed is necessary as the cell contents are rapidly leaking out of the cell (78). Tests like the detergent and laser experiments were conducted to determine if ESCRTs could repair the plasma membrane damage done by GsdmD-mediated pore formation (80). ESCRT, when Ca2+ influx from the extracellular space was present, successfully repaired the plasma membrane, making it possible for the cell to survive pyroptosis by both pathways (80). This could be a potential treatment option if Ca2+ supplementation could enhance ESCRT responses in areas affected by pyroptosis.
Inflammasome role in MS
Inflammasomes: NLRP3
Genetics have also been shown to affect MS severity and progression. Specifically, two gain of function mutations, one affecting the NLRP3 inflammasome and the other affecting IL-1β, correlate with increased MS severity. The NLRP3 mutation is Q705K, and the IL-1β mutation is -511 C > T (81). The single nucleotide polymorphisms (SNP) mutation affecting IL-1β is found more often in the progressive forms of MS than in the less severe relapsing-remitting form (82). Furthermore, a mutation in the NLR family CARD domain-containing 4 (NLRC4) gene lowers NLRC4 and IL-18 levels, leading to better results from interferon-β (IFN-β) treatment (81).
Recent studies have delved deeper into the correlation between inflammasome proteins and MS by analyzing their presence and levels in serum samples from MS patients (7,44-47,83). Elevated caspase-1, ASC, and IL-18 levels underscore their potential role in the disease’s inflammatory processes. These studies suggest these proteins could serve as biomarkers for diagnosing MS and provide insights into its pathogenesis and severity. By identifying these proteins’ elevated levels, the research supports their utility in developing diagnostic tools and potentially guiding therapeutic interventions for MS and offers a promising avenue for improving patient outcomes through targeted treatments.
Inflammasomes: NLRP1
The NLRP1 inflammasome shares many traits with NLRP3; however, it also can activate caspase 1 without first binding ASC (84). A unique case exists in which NLRP1 does use ASC when activating caspase 1, but this occurs only when NLRP1 loses its CARD, thus necessitating the use of ASC (Figure 2). The link between NLRP1 and MS is a possible genetic mutation that can lead to increased IL-1β and IL-18 levels in the CNS, promoting the inflammation associated with MS, but another study failed to find this same correlation (82).
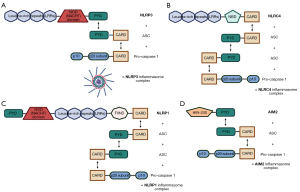
Inflammasomes: NLRC4
The NLRC4 inflammasome can similarly activate caspase 1, and there is a correlation between its upregulation and MS inflammation (81). Additionally, NLRC4 is associated with the microglia immune cells and is present in demyelinated regions of MS patients (85) (Figure 3). Microglial accumulation results from the work of NLRC4 in partnership with NLRP3, leading to increased demyelination (86). NLRC4 and NLRP3 knockout models did not experience either microglial accumulation or subsequent demyelination, further implicating these inflammasomes in MS progression (87). Further studies have shown that loss-of-function mutations in NLRC4 improve disease response to IFN-β treatment and lower IL-18 levels, while constitutive NLRP3 expression is associated with worsening disease progression (82,88).
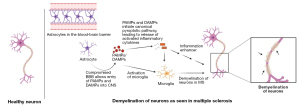
Inflammasomes: absent in melanoma 2 (AIM2)
The AIM2 inflammasome also uses ASC to induce pyroptosis. AIM2 is particularly activated by the DNA of neurons in the CNS, causing inflammation (88). The IFN-β treatment intended to target the NLRP3 and NLRC4 inflammasomes also downregulates AIM2 in MS patients (89). This is contrary to what is expected since IFN-β normally primes AIM2. However, GsdmD may be the reason, as it reduces the effect of cytosolic DNA on AIM2 and also reduces the effects of IFN-β (82). Thus, IFN-β can reduce the amount of cytokines released by all three inflammasomes and reduce overall CNS inflammation in MS patients.
Treatment options
IFN-β
With inflammasomes playing such a critical role in the development of MS, targeting them in MS treatment is one possible option. IFN-β is a possible therapeutic that inhibits the NLRP1 and NLRP3 inflammasomes (82). A study conducted to test the effect of IFN-β in MS patients found that those with higher initial NLRP3 and IL-1β mRNA expression had a decreased response to the treatment (83). This was consistent with another study that found EAE symptoms were alleviated by IFN-β treatment (82). IFN-β works by first inhibiting IFN signaling (14). This IFN signaling controls signal transducer and activator of transcription (STAT1) production, which in turn works to suppress the NLRP1 and NLRP3 inflammasomes (90,91). This suppression can occur either directly by STAT1 or secondarily via Signal transducer and activator of transcription 3 (STAT3) activation which suppress IL-1β production (91). Additionally, IFN-β was found to increase IL-10 levels, a cytokine that works to counter the inflammatory response caused by IL-1β and NLRP3 (92,93). IL-10 also prevents IL-1β production and activation of the NLRP3 inflammasome; without the NLRP3 inflammasome, proIL-1β cannot be activated into its mature form via caspase 1 (91).
There are several options for the administration of IFN-β treatments. The first is IFNβ-1b, which is delivered subcutaneously (94). Clinical trials for this treatment showed that MS attacks were reduced in both severity and frequency of relapsing symptoms (95). It was also observed that there was a reduction in both the size and number of MS lesions detected using MRI. IFNb-1a are recombinant peptides that feature an identical amino acid sequence as endogenous human IFNb and are an alternative treatment that can be given intramuscularly or subcutaneously (96). Lastly, Peginterferon beta-1a reduces dosing frequency due to the pegylation of IFN-β and is administered subcutaneously (97). In a head-to-head comparison, administration of peginterferon beta-1a was found to provide greater drug exposure without a greater rate of side effects than IFNb-1a (97).
Fingolimod
Fingolimod was the first drug that targets sphingosine 1-phosphate (S1P) receptors to be approved for treating people with RRMS (8,98-101). It works by binding to a specific target and causes the S1P receptor to be pulled into the cell, which stops lymphocytes from leaving the lymph nodes and prevents these cells from entering the CNS. As a result, it effectively confines naive and central memory T cells within secondary lymphoid organs, leading to a significant drop in the total number of lymphocytes in the blood.
Ocrelizumab
Ocrelizumab, a humanized monoclonal antibody, operates by selectively eliminating CD20+ B-cells (102-104). In the OPERA I and OPERA II Phase III clinical studies comparing ocrelizumab with interferon beta-1a, ocrelizumab demonstrated a substantial decrease in the annualized relapse rate (ARR) for RRMS patients (104,105). Additionally, a significant reduction in the number of patients experiencing confirmed disability progression (CDP) at 12 and 24 weeks was observed in those receiving ocrelizumab. In the ORATORIO trial, patients with PPMS treated with ocrelizumab showed lower rates of CDP at 12 and 24 weeks compared to those given a placebo (106).
IC100
IC100 (Figure 4) is a humanized IgG4 monoclonal antibody targeting ASC, which operates by internalizing into the cell, associating with endosomes, and binding intracellular ASC and tripartite motif containing protein-21 (TRIM21) (107,108). This prevents ASC from polymerizing and assembling into specks, inhibiting inflammasome activation and IL-1β maturation. Importantly, IC100 can cross the BBB, allowing the treatment access to the CNS. The antibody is internalized by binding with neonatal fragment crystallizable receptor (FcRn) and is endocytosed into the cell. Once inside the cell, IC100 binds to cytosolic ASC and TRIM21. Binding to TRIM21 would typically result in the degradation of the attached antibody, but it was determined that IC100 avoids proteasomal degradation, allowing the treatment to have a reasonable half-life. Since IC100 targets ASC, it allows for the inhibition of not only the NLRP3 inflammasome but also NLRP1, NLRC4, and AIM2 inflammasomes, further expanding its utility. Furthermore, IC100 reduced the severity and progression of EAE in rodent models and improved outcomes for patients following injuries to the CNS (109).
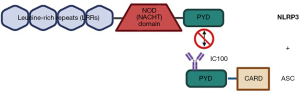
Ketotifen
Ketotifen, a mast cell stabilizer, has succeeded in the murine models, effectively decreasing NLRP3 inflammasome activation and T-cell infiltration of the CNS (110). Ketotifen accomplishes this by inhibiting mast cell degranulation, which normally activates NLRP3 (82). The treatment works not by decreasing the number of mast cells present but rather by decreasing the expression of enzymes produced by these cells, carboxypeptidase A3 (CPA3) and chymase 1 (CMA1) in particular (110). There is substantial evidence that mast cells play a role in BBB disruption, allowing inflammatory cells access to the CNS (110). CNS barrier disruption is accomplished by vasoactive mediators, which lead to endothelial activation (110). If the CNS barrier is compromised, IL-18, IL-1β, and potentially additional cytokines can exacerbate the inflammatory response. Evaluation of the effects of ketotifen has shown support for the role of Ketotifen in restoring normal permeability in the CNS (110). However, this treatment only proved effective when administered within the first 7 to 17 days of disease onset, which could prove to be a problem if it is to be used to treat MS patients (82,110).
Dimethyl fumarate (DMF)
One final treatment is DMF, which has been hypothesized to interfere with GsdmD activation and thus decrease the inflammation associated with MS (111) (Figure 5). Specifically, DMF succinates residue 195 of the GsdmD sequence, while caspase 1 cleaves GsdmD at Asp 275, releasing the N-terminus from the autoinhibitory C-terminus (111). It is thought that DMF can prevent the cleavage of GsdmD if the succination site and cleavage site are close to each other when the protein GsdmD is in its final 3D conformation. Evidence within the literature also supports the notion that DMF can reduce cleavage of GsdmE within GsdmD-deficient cells (112). We tested this hypothesis using molecular modeling and found that both residues are located near each other in the final 3D conformation, validating the mechanism of action proposed for this treatment. It has also been proposed that DMF can modify the GsdmD gene through hypermethylation in natural killer cells (113). The effect of hypermethylation on the promoter region of a gene acts as a blocker to transcription factors and results in gene silencing (113). In addition, a recent report demonstrated a significant increase in natural killer cell count after two years of DMF treatment (114). This inhibitor has already succeeded in clinical trials, and this alternate mechanism shows additional promise in treating inflammatory disorders like MS (115).
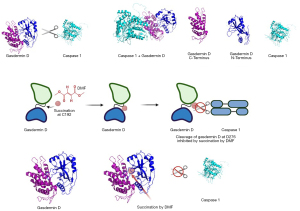
An observational study conducted with a cohort of approximately 2,000 patients suffering from RRMS tested the long-term effects of treatment with DMF vs. teriflunomide (116). With a median follow-up of 1.9 years, the authors found that those in the DMF group were less likely to experience a relapse in MS than those that took teriflunomide, and the patients that switched to DMF from teriflunomide also experienced longer times between relapses. The main limitation of this study, which the authors address, is that it didn’t utilize MRI data to monitor disease progress but monitored new or exacerbated neurological symptoms persisting for >24 hours. While this data is enough to conclude that DMF is a more effective treatment than teriflunomide, more precise worsening criteria and frequent monitoring will be an analysis worth pursuing.
Conclusions
Concluding this comprehensive review on MS, NLRP3 inflammasomes, GsdmD, and therapeutic interventions, it is evident that understanding the intricate pathways of inflammation and cell death in MS offers promising avenues for developing targeted treatments. The role of the NLRP3 inflammasome in MS pathogenesis underscores the importance of the immune system’s balance in maintaining neural health and the devastating consequences of its dysregulation. The canonical and noncanonical pathways leading to pyroptosis, mediated by GsdmD, highlight a critical mechanism by which inflammation contributes to the progression of MS, suggesting that inhibiting these pathways could mitigate disease severity and improve patient outcomes.
Emerging therapies, including IFN-β, fingolimod, ocrelizumab, IC100, ketotifen, and DMF, have shown promise in modulating the inflammatory response, offering hope for patients suffering from this debilitating disease. These treatments, targeting various stages of the inflammatory cascade, from inflammasome assembly to cytokine release and pyroptosis, underline the importance of a multi-targeted approach in managing MS. Furthermore, the potential of novel therapeutic targets, such as inhibiting specific components of the inflammasome complex or modulating GsdmD activity, opens new doors for research and development.
Despite these advances, the complexity of MS and its underlying mechanisms pose significant challenges. The interplay between genetic factors, environmental triggers, and immune system responses necessitates a personalized treatment approach, considering each patient’s unique pathophysiological profile. As research progresses, the integration of biomarkers for early detection and monitoring and advances in drug delivery systems to target CNS more effectively will be critical.
In conclusion, the battle against MS is ongoing, but the insights gained from studying the NLRP3 inflammasome and GsdmD pathways provide a solid foundation for developing more effective and targeted therapies. Continued interdisciplinary research combining molecular biology, immunology, and clinical sciences will be essential in translating these findings into clinical practice, with the goal of improving the quality of life for patients with MS. As we move forward, it is imperative to sustain investment in research, foster collaboration among scientists and clinicians, and engage with patient communities to ensure that the promising potential of current and future therapies is fully realized.
Acknowledgments
Funding: This project was supported by
Footnote
Peer Review File: Available at https://atm.amegroups.com/article/view/10.21037/atm-23-1960/prf
Conflicts of Interest: All authors have completed the ICMJE uniform disclosure form (available at https://atm.amegroups.com/article/view/10.21037/atm-23-1960/coif). S.W. serves as an unpaid editorial board member of Annals of Translational Medicine from September 2023 to August 2025. The other authors have no conflicts of interest to declare.
Ethical Statement: The authors are accountable for all aspects of the work in ensuring that questions related to the accuracy or integrity of any part of the work are appropriately investigated and resolved.
Open Access Statement: This is an Open Access article distributed in accordance with the Creative Commons Attribution-NonCommercial-NoDerivs 4.0 International License (CC BY-NC-ND 4.0), which permits the non-commercial replication and distribution of the article with the strict proviso that no changes or edits are made and the original work is properly cited (including links to both the formal publication through the relevant DOI and the license). See: https://creativecommons.org/licenses/by-nc-nd/4.0/.
References
- Krugmann B, Radulescu A, Appavou MS, et al. Membrane stiffness and myelin basic protein binding strength as molecular origin of multiple sclerosis. Sci Rep 2020;10:16691. [Crossref] [PubMed]
- Cahalane AM, Kearney H, Purcell YM, et al. MRI and multiple sclerosis--the evolving role of MRI in the diagnosis and management of MS: the radiologist's perspective. Ir J Med Sci 2018;187:781-7. [Crossref] [PubMed]
- Louapre C, Lubetzki C. Neurodegeneration in multiple sclerosis is a process separate from inflammation: Yes. Mult Scler 2015;21:1626-8. [Crossref] [PubMed]
- Dobson R, Giovannoni G. Multiple sclerosis - a review. Eur J Neurol 2019;26:27-40. [Crossref] [PubMed]
- Bjornevik K, Cortese M, Healy BC, et al. Longitudinal analysis reveals high prevalence of Epstein-Barr virus associated with multiple sclerosis. Science 2022;375:296-301. [Crossref] [PubMed]
- Burton EM, Goldbach-Mansky R, Bhaduri-McIntosh S. A promiscuous inflammasome sparks replication of a common tumor virus. Proc Natl Acad Sci U S A 2020;117:1722-30. [Crossref] [PubMed]
- Malhotra S, Costa C, Eixarch H, et al. NLRP3 inflammasome as prognostic factor and therapeutic target in primary progressive multiple sclerosis patients. Brain 2020;143:1414-30. [Crossref] [PubMed]
- Malhotra S, Hurtado-Navarro L, Pappolla A, et al. Increased NLRP3 Inflammasome Activation and Pyroptosis in Patients With Multiple Sclerosis With Fingolimod Treatment Failure. Neurol Neuroimmunol Neuroinflamm 2023;10:e200100. [Crossref] [PubMed]
- Guarda G, Zenger M, Yazdi AS, et al. Differential expression of NLRP3 among hematopoietic cells. J Immunol 2011;186:2529-34. [Crossref] [PubMed]
- de Rivero Vaccari JP, Brand F 3rd, Adamczak S, et al. Exosome-mediated inflammasome signaling after central nervous system injury. J Neurochem 2016;136:39-48. [Crossref] [PubMed]
- Lee SW, Gajavelli S, Spurlock MS, et al. Microglial Inflammasome Activation in Penetrating Ballistic-Like Brain Injury. J Neurotrauma 2018;35:1681-93. [Crossref] [PubMed]
- Gris D, Ye Z, Iocca HA, et al. NLRP3 plays a critical role in the development of experimental autoimmune encephalomyelitis by mediating Th1 and Th17 responses. J Immunol 2010;185:974-81. [Crossref] [PubMed]
- Jha S, Srivastava SY, Brickey WJ, et al. The inflammasome sensor, NLRP3, regulates CNS inflammation and demyelination via caspase-1 and interleukin-18. J Neurosci 2010;30:15811-20. [Crossref] [PubMed]
- Tschopp J, Schroder K. NLRP3 inflammasome activation: The convergence of multiple signalling pathways on ROS production? Nat Rev Immunol 2010;10:210-5. [Crossref] [PubMed]
- Stehlik C, Lee SH, Dorfleutner A, et al. Apoptosis-associated speck-like protein containing a caspase recruitment domain is a regulator of procaspase-1 activation. J Immunol 2003;171:6154-63. [Crossref] [PubMed]
- Miao EA, Rajan JV, Aderem A. Caspase-1-induced pyroptotic cell death. Immunol Rev 2011;243:206-14. [Crossref] [PubMed]
- Sborgi L, Rühl S, Mulvihill E, et al. GSDMD membrane pore formation constitutes the mechanism of pyroptotic cell death. EMBO J 2016;35:1766-78. [Crossref] [PubMed]
- Mulvihill E, Sborgi L, Mari SA, et al. Mechanism of membrane pore formation by human gasdermin-D. EMBO J 2018;37:e98321. [Crossref] [PubMed]
- Rathkey JK, Zhao J, Liu Z, et al. Chemical disruption of the pyroptotic pore-forming protein gasdermin D inhibits inflammatory cell death and sepsis. Sci Immunol 2018;3:eaat2738. [Crossref] [PubMed]
- Groß CJ, Mishra R, Schneider KS, et al. K(+) Efflux-Independent NLRP3 Inflammasome Activation by Small Molecules Targeting Mitochondria. Immunity 2016;45:761-73. [Crossref] [PubMed]
- Chen KW, Bezbradica JS, Groß CJ, et al. The murine neutrophil NLRP3 inflammasome is activated by soluble but not particulate or crystalline agonists. Eur J Immunol 2016;46:1004-10. [Crossref] [PubMed]
- Liu D, Zeng X, Li X, et al. Advances in the molecular mechanisms of NLRP3 inflammasome activators and inactivators. Biochem Pharmacol 2020;175:113863. [Crossref] [PubMed]
- Ito T. PAMPs and DAMPs as triggers for DIC. J Intensive Care 2014;2:67. [Crossref] [PubMed]
- Kelley N, Jeltema D, Duan Y, et al. The NLRP3 Inflammasome: An Overview of Mechanisms of Activation and Regulation. Int J Mol Sci 2019;20:3328. [Crossref] [PubMed]
- Swanson KV, Deng M, Ting JP. The NLRP3 inflammasome: molecular activation and regulation to therapeutics. Nat Rev Immunol 2019;19:477-89. [Crossref] [PubMed]
- Zhang Z, Meszaros G, He WT, et al. Protein kinase D at the Golgi controls NLRP3 inflammasome activation. J Exp Med 2017;214:2671-93. [Crossref] [PubMed]
- Liu T, Zhang L, Joo D, et al. NF-κB signaling in inflammation. Signal Transduct Target Ther 2017;2:17023. [Crossref] [PubMed]
- Mezzasoma L, Antognelli C, Talesa VN. Atrial natriuretic peptide down-regulates LPS/ATP-mediated IL-1β release by inhibiting NF-kB, NLRP3 inflammasome and caspase-1 activation in THP-1 cells. Immunol Res 2016;64:303-12. [Crossref] [PubMed]
- Kircheis R, Haasbach E, Lueftenegger D, et al. NF-κB Pathway as a Potential Target for Treatment of Critical Stage COVID-19 Patients. Front Immunol 2020;11:598444. [Crossref] [PubMed]
- Akther M, Haque ME, Park J, et al. NLRP3 Ubiquitination-A New Approach to Target NLRP3 Inflammasome Activation. Int J Mol Sci 2021;22:8780. [Crossref] [PubMed]
- Xia J, Jiang S, Dong S, et al. The Role of Post-Translational Modifications in Regulation of NLRP3 Inflammasome Activation. Int J Mol Sci 2023;24:6126. [Crossref] [PubMed]
- He Y, Zeng MY, Yang D, et al. NEK7 is an essential mediator of NLRP3 activation downstream of potassium efflux. Nature 2016;530:354-7. [Crossref] [PubMed]
- Andreeva L, David L, Rawson S, et al. NLRP3 cages revealed by full-length mouse NLRP3 structure control pathway activation. Cell 2021;184:6299-6312.e22. [Crossref] [PubMed]
- Xiao L, Magupalli VG, Wu H. Cryo-EM structures of the active NLRP3 inflammasome disc. Nature 2023;613:595-600. [Crossref] [PubMed]
- Shi H, Wang Y, Li X, et al. NLRP3 activation and mitosis are mutually exclusive events coordinated by NEK7, a new inflammasome component. Nat Immunol 2016;17:250-8. [Crossref] [PubMed]
- Lawrence T. The nuclear factor NF-kappaB pathway in inflammation. Cold Spring Harb Perspect Biol 2009;1:a001651. [Crossref] [PubMed]
- Franchi L, Muñoz-Planillo R, Núñez G. Sensing and reacting to microbes through the inflammasomes. Nat Immunol 2012;13:325-32. [Crossref] [PubMed]
- Gong T, Yang Y, Jin T, et al. Orchestration of NLRP3 Inflammasome Activation by Ion Fluxes. Trends Immunol 2018;39:393-406. [Crossref] [PubMed]
- Zahid A, Li B, Kombe AJK, et al. Pharmacological Inhibitors of the NLRP3 Inflammasome. Front Immunol 2019;10:2538. [Crossref] [PubMed]
- Ball DP, Taabazuing CY, Griswold AR, et al. Caspase-1 interdomain linker cleavage is required for pyroptosis. Life Sci Alliance 2020;3:e202000664. [Crossref] [PubMed]
- Moretti J, Blander JM. Increasing complexity of NLRP3 inflammasome regulation. J Leukoc Biol 2021;109:561-71. [Crossref] [PubMed]
- Zhou R, Yazdi AS, Menu P, et al. A role for mitochondria in NLRP3 inflammasome activation. Nature 2011;469:221-5. [Crossref] [PubMed]
- Vanaja SK, Rathinam VA, Fitzgerald KA. Mechanisms of inflammasome activation: recent advances and novel insights. Trends Cell Biol 2015;25:308-15. [Crossref] [PubMed]
- Johnson NH, Hadad R, Taylor RR, et al. Inflammatory Biomarkers of Traumatic Brain Injury. Pharmaceuticals (Basel) 2022;15:660. [Crossref] [PubMed]
- Keane RW, Dietrich WD, de Rivero Vaccari JP. Inflammasome Proteins As Biomarkers of Multiple Sclerosis. Front Neurol 2018;9:135. [Crossref] [PubMed]
- Kerr N, García-Contreras M, Abbassi S, et al. Inflammasome Proteins in Serum and Serum-Derived Extracellular Vesicles as Biomarkers of Stroke. Front Mol Neurosci 2018;11:309. [Crossref] [PubMed]
- Suceveanu AI, Mazilu L, Katsiki N, et al. NLRP3 Inflammasome Biomarker-Could Be the New Tool for Improved Cardiometabolic Syndrome Outcome. Metabolites 2020;10:448. [Crossref] [PubMed]
- Moossavi M, Parsamanesh N, Bahrami A, et al. Role of the NLRP3 inflammasome in cancer. Mol Cancer 2018;17:158. [Crossref] [PubMed]
- Boucher D, Monteleone M, Coll RC, et al. Caspase-1 self-cleavage is an intrinsic mechanism to terminate inflammasome activity. J Exp Med 2018;215:827-40. [Crossref] [PubMed]
- Wang K, Sun Q, Zhong X, et al. Structural Mechanism for GSDMD Targeting by Autoprocessed Caspases in Pyroptosis. Cell 2020;180:941-955.e20. [Crossref] [PubMed]
- Malik A, Kanneganti TD. Inflammasome activation and assembly at a glance. J Cell Sci 2017;130:3955-63. [Crossref] [PubMed]
- Song L, Pei L, Yao S, et al. NLRP3 Inflammasome in Neurological Diseases, from Functions to Therapies. Front Cell Neurosci 2017;11:63. [Crossref] [PubMed]
- Kordes M, Matuschewski K, Hafalla JC. Caspase-1 activation of interleukin-1β (IL-1β) and IL-18 is dispensable for induction of experimental cerebral malaria. Infect Immun 2011;79:3633-41. [Crossref] [PubMed]
- Dorrington MG, Fraser IDC. NF-κB Signaling in Macrophages: Dynamics, Crosstalk, and Signal Integration. Front Immunol 2019;10:705. [Crossref] [PubMed]
- Faria SS, Costantini S, de Lima VCC, et al. NLRP3 inflammasome-mediated cytokine production and pyroptosis cell death in breast cancer. J Biomed Sci 2021;28:26. [Crossref] [PubMed]
- Vande Walle L, Lamkanfi M. Inflammasomes: caspase-1-activating platforms with critical roles in host defense. Front Microbiol 2011;2:3. [Crossref] [PubMed]
- Zhao H, Wu L, Yan G, et al. Inflammation and tumor progression: signaling pathways and targeted intervention. Signal Transduct Target Ther 2021;6:263. [Crossref] [PubMed]
- Heilig R, Dilucca M, Boucher D, et al. Caspase-1 cleaves Bid to release mitochondrial SMAC and drive secondary necrosis in the absence of GSDMD. Life Sci Alliance 2020;3:e202000735. [Crossref] [PubMed]
- Inoue M, Shinohara ML. NLRP3 Inflammasome and MS/EAE. Autoimmune Dis 2013;2013:859145. [Crossref] [PubMed]
- de Jong BA, Huizinga TW, Bollen EL, et al. Production of IL-1beta and IL-1Ra as risk factors for susceptibility and progression of relapse-onset multiple sclerosis. J Neuroimmunol 2002;126:172-9. [Crossref] [PubMed]
- Weber A, Wasiliew P, Kracht M. Interleukin-1 (IL-1) pathway. Sci Signal 2010;3:cm1. [Crossref] [PubMed]
- Rossi S, Furlan R, De Chiara V, et al. Interleukin-1β causes synaptic hyperexcitability in multiple sclerosis. Ann Neurol 2012;71:76-83. [Crossref] [PubMed]
- Rossi S, Studer V, Motta C, et al. Cerebrospinal fluid detection of interleukin-1β in phase of remission predicts disease progression in multiple sclerosis. J Neuroinflammation 2014;11:32. [Crossref] [PubMed]
- Lin CC, Edelson BT. New Insights into the Role of IL-1β in Experimental Autoimmune Encephalomyelitis and Multiple Sclerosis. J Immunol 2017;198:4553-60. [Crossref] [PubMed]
- Losy J, Niezgoda A. IL-18 in patients with multiple sclerosis. Acta Neurol Scand 2001;104:171-3. [Crossref] [PubMed]
- Inoue M, Williams KL, Oliver T, et al. Interferon-β therapy against EAE is effective only when development of the disease depends on the NLRP3 inflammasome. Sci Signal 2012;5:ra38. [Crossref] [PubMed]
- Gutcher I, Urich E, Wolter K, et al. Interleukin 18-independent engagement of interleukin 18 receptor-alpha is required for autoimmune inflammation. Nat Immunol 2006;7:946-53. [Crossref] [PubMed]
- Olcum M, Tastan B, Kiser C, et al. Microglial NLRP3 inflammasome activation in multiple sclerosis. Adv Protein Chem Struct Biol 2020;119:247-308. [Crossref] [PubMed]
- Zhao C, Zhao W. NLRP3 Inflammasome-A Key Player in Antiviral Responses. Front Immunol 2020;11:211. [Crossref] [PubMed]
- Tsuchiya K, Nakajima S, Hosojima S, et al. Caspase-1 initiates apoptosis in the absence of gasdermin D. Nat Commun 2019;10:2091. [Crossref] [PubMed]
- Chen KW, Demarco B, Heilig R, et al. Extrinsic and intrinsic apoptosis activate pannexin-1 to drive NLRP3 inflammasome assembly. EMBO J 2019;38:e101638. [Crossref] [PubMed]
- Shi J, Zhao Y, Wang K, et al. Cleavage of GSDMD by inflammatory caspases determines pyroptotic cell death. Nature 2015;526:660-5. [Crossref] [PubMed]
- Conos SA, Lawlor KE, Vaux DL, et al. Cell death is not essential for caspase-1-mediated interleukin-1β activation and secretion. Cell Death Differ 2016;23:1827-38. [Crossref] [PubMed]
- Man SM, Karki R, Briard B, et al. Differential roles of caspase-1 and caspase-11 in infection and inflammation. Sci Rep 2017;7:45126. [Crossref] [PubMed]
- Huang X, Feng Y, Xiong G, et al. Caspase-11, a specific sensor for intracellular lipopolysaccharide recognition, mediates the non-canonical inflammatory pathway of pyroptosis. Cell Biosci 2019;9:31. [Crossref] [PubMed]
- Rühl S, Broz P. Caspase-11 activates a canonical NLRP3 inflammasome by promoting K(+) efflux. Eur J Immunol 2015;45:2927-36. [Crossref] [PubMed]
- Lee BL, Stowe IB, Gupta A, et al. Caspase-11 auto-proteolysis is crucial for noncanonical inflammasome activation. J Exp Med 2018;215:2279-88. [Crossref] [PubMed]
- Vietri M, Radulovic M, Stenmark H. The many functions of ESCRTs. Nat Rev Mol Cell Biol 2020;21:25-42. [Crossref] [PubMed]
- Christ L, Raiborg C, Wenzel EM, et al. Cellular Functions and Molecular Mechanisms of the ESCRT Membrane-Scission Machinery. Trends Biochem Sci 2017;42:42-56. [Crossref] [PubMed]
- Rühl S, Shkarina K, Demarco B, et al. ESCRT-dependent membrane repair negatively regulates pyroptosis downstream of GSDMD activation. Science 2018;362:956-60. [Crossref] [PubMed]
- Soares JL, Oliveira EM, Pontillo A. Variants in NLRP3 and NLRC4 inflammasome associate with susceptibility and severity of multiple sclerosis. Mult Scler Relat Disord 2019;29:26-34. [Crossref] [PubMed]
- Govindarajan V, de Rivero Vaccari JP, Keane RW. Role of inflammasomes in multiple sclerosis and their potential as therapeutic targets. J Neuroinflammation 2020;17:260. [Crossref] [PubMed]
- Malhotra S, Río J, Urcelay E, et al. NLRP3 inflammasome is associated with the response to IFN-β in patients with multiple sclerosis. Brain 2015;138:644-52. [Crossref] [PubMed]
- Latz E, Xiao TS, Stutz A. Activation and regulation of the inflammasomes. Nat Rev Immunol 2013;13:397-411. [Crossref] [PubMed]
- Wang LQ, Zheng YY, Zhou HJ, et al. LncRNA-Fendrr protects against the ubiquitination and degradation of NLRC4 protein through HERC2 to regulate the pyroptosis of microglia. Mol Med 2021;27:39. [Crossref] [PubMed]
- Sariol A, Mackin S, Allred MG, et al. Microglia depletion exacerbates demyelination and impairs remyelination in a neurotropic coronavirus infection. Proc Natl Acad Sci U S A 2020;117:24464-74. [Crossref] [PubMed]
- Freeman L, Guo H, David CN, et al. NLR members NLRC4 and NLRP3 mediate sterile inflammasome activation in microglia and astrocytes. J Exp Med 2017;214:1351-70. [Crossref] [PubMed]
- Zhao J, Miller-Little W, Li X. Inflammasome-independent functions of AIM2. J Exp Med 2021;218:e20210273. [Crossref] [PubMed]
- Noroozi S, Meimand HAE, Arababadi MK, et al. The Effects of IFN-β 1a on the Expression of Inflammasomes and Apoptosis-Associated Speck-Like Proteins in Multiple Sclerosis Patients. Mol Neurobiol 2017;54:3031-7. [Crossref] [PubMed]
- Hu X, Ivashkiv LB. Cross-regulation of signaling pathways by interferon-gamma: implications for immune responses and autoimmune diseases. Immunity 2009;31:539-50. [Crossref] [PubMed]
- Guarda G, Braun M, Staehli F, et al. Type I interferon inhibits interleukin-1 production and inflammasome activation. Immunity 2011;34:213-23. [Crossref] [PubMed]
- Zhang L, Yuan S, Cheng G, et al. Type I IFN promotes IL-10 production from T cells to suppress Th17 cells and Th17-associated autoimmune inflammation. PLoS One 2011;6:e28432. [Crossref] [PubMed]
- Gurung P, Li B, Subbarao Malireddi RK, et al. Chronic TLR Stimulation Controls NLRP3 Inflammasome Activation through IL-10 Mediated Regulation of NLRP3 Expression and Caspase-8 Activation. Sci Rep 2015;5:14488. [Crossref] [PubMed]
- Filipi M, Jack S. Interferons in the Treatment of Multiple Sclerosis: A Clinical Efficacy, Safety, and Tolerability Update. Int J MS Care 2020;22:165-72. [Crossref] [PubMed]
- Interferon beta-1b in the treatment of multiple sclerosis: final outcome of the randomized controlled trial. The IFNB Multiple Sclerosis Study Group and The University of British Columbia MS/MRI Analysis Group. Neurology 1995;45:1277-85.
- Loma I, Heyman R. Multiple sclerosis: pathogenesis and treatment. Curr Neuropharmacol 2011;9:409-16. [Crossref] [PubMed]
- Hu X, Shang S, Nestorov I, et al. COMPARE: Pharmacokinetic profiles of subcutaneous peginterferon beta-1a and subcutaneous interferon beta-1a over 2 weeks in healthy subjects. Br J Clin Pharmacol 2016;82:380-8. [Crossref] [PubMed]
- Callegari I, Derfuss T, Galli E. Update on treatment in multiple sclerosis. Presse Med 2021;50:104068. [Crossref] [PubMed]
- Di Menna L, Molinaro G, Di Nuzzo L, et al. Fingolimod protects cultured cortical neurons against excitotoxic death. Pharmacol Res 2013;67:1-9. [Crossref] [PubMed]
- Guo Y, Gan X, Zhou H, et al. Fingolimod suppressed the chronic unpredictable mild stress-induced depressive-like behaviors via affecting microglial and NLRP3 inflammasome activation. Life Sci 2020;263:118582. [Crossref] [PubMed]
- Yao S, Li L, Sun X, et al. FTY720 Inhibits MPP(+)-Induced Microglial Activation by Affecting NLRP3 Inflammasome Activation. J Neuroimmune Pharmacol 2019;14:478-92. [Crossref] [PubMed]
- Gelfand JM, Cree BAC, Hauser SL. Ocrelizumab and Other CD20(+) B-Cell-Depleting Therapies in Multiple Sclerosis. Neurotherapeutics 2017;14:835-41. [Crossref] [PubMed]
- Kadowaki A, Quintana FJ. The NLRP3 inflammasome in progressive multiple sclerosis. Brain 2020;143:1286-8. [Crossref] [PubMed]
- Lamb YN. Ocrelizumab: A Review in Multiple Sclerosis. Drugs 2022;82:323-34. [Crossref] [PubMed]
- Pontieri L, Blinkenberg M, Bramow S, et al. Ocrelizumab treatment in multiple sclerosis: A Danish population-based cohort study. Eur J Neurol 2022;29:496-504. [Crossref] [PubMed]
- Montalban X, Matthews PM, Simpson A, et al. Real-world evaluation of ocrelizumab in multiple sclerosis: A systematic review. Ann Clin Transl Neurol 2023;10:302-11. [Crossref] [PubMed]
- de Rivero Vaccari JP, Mim C, Hadad R, et al. Mechanism of action of IC 100, a humanized IgG4 monoclonal antibody targeting apoptosis-associated speck-like protein containing a caspase recruitment domain (ASC). Transl Res 2023;251:27-40. [Crossref] [PubMed]
- Ren H, Kong Y, Liu Z, et al. Selective NLRP3 (Pyrin Domain-Containing Protein 3) Inflammasome Inhibitor Reduces Brain Injury After Intracerebral Hemorrhage. Stroke 2018;49:184-92. [Crossref] [PubMed]
- Desu HL, Plastini M, Illiano P, et al. IC100: a novel anti-ASC monoclonal antibody improves functional outcomes in an animal model of multiple sclerosis. J Neuroinflammation 2020;17:143. [Crossref] [PubMed]
- Pinke KH, Zorzella-Pezavento SFG, de Campos Fraga-Silva TF, et al. Calming Down Mast Cells with Ketotifen: A Potential Strategy for Multiple Sclerosis Therapy? Neurotherapeutics 2020;17:218-34. [Crossref] [PubMed]
- Humphries F, Shmuel-Galia L, Ketelut-Carneiro N, et al. Succination inactivates gasdermin D and blocks pyroptosis. Science 2020;369:1633-7. [Crossref] [PubMed]
- Place DE, Kanneganti TD. Metabolic regulation of pyroptotic cell death expands the therapeutic landscape for treating inflammatory disease. Signal Transduct Target Ther 2021;6:37. [Crossref] [PubMed]
- Muhammad JS, Jayakumar MN, Elemam NM, et al. Gasdermin D Hypermethylation Inhibits Pyroptosis And LPS-Induced IL-1β Release From NK92 Cells. Immunotargets Ther 2019;8:29-41. [Crossref] [PubMed]
- Al-Ani M, Elemam NM, Hundt JE, et al. Drugs for Multiple Sclerosis Activate Natural Killer Cells: Do They Protect Against COVID-19 Infection? Infect Drug Resist 2020;13:3243-54. [Crossref] [PubMed]
- Hu Y, Wang B, Li S, et al. Pyroptosis, and its Role in Central Nervous System Disease. J Mol Biol 2022;434:167379. [Crossref] [PubMed]
- Müller J, Schädelin S, Lorscheider J, et al. Comparative analysis of dimethyl fumarate and teriflunomide in relapsing-remitting multiple sclerosis. Eur J Neurol 2023;30:3809-18. [Crossref] [PubMed]