Aldehyde dehydrogenases in cancer stem cells: potential as therapeutic targets
Solid tumors are composed of a variety of genetically distinct cancer cell types that are adapted to the conditions of the microenvironment. The majority of cells that comprise the tumor have limited capacity for self-renewal and are surprisingly, poorly tumorigenic. It is generally accepted that malignant tumors contain a small subpopulation of cells with stem cell properties (1,2). These cells, referred to as cancer stem cells (CSCs) or tumor initiating cells (TICs) are capable of evading current anti-neoplastic treatment regimens including chemotherapy and radiotherapy and dividing and differentiating into normal tumor cells after conclusion of treatment, leading to tumor recurrence or metastasis (2-4). This creates a serious problem with recurrent or metastatic tumors being responsible for most (≈90%) cancer deaths. The majority of current treatment strategies are based upon the clonal model of cancer and, therefore, target rapidly dividing cells leaving the quiescent and highly chemoresistant CSCs behind (Figure 1). This issue highlights the drastic need to develop new drugs and treatment strategies that can also target CSCs. Members of the aldehyde dehydrogenase (ALDH) family of proteins comprise a particularly interesting new class of potential targets. Humans have 19 ALDH proteins (5) and many have at least been determined to possess cancer-related functions [reviewed in (6)]. ALDHs are spread throughout the body, catalyze oxidation of aldehydes and function in cellular detoxification, retinoic acid (RA) metabolism and signaling, development, protection from reactive oxygen species (ROS), and maintenance of the eye and vision (7-11). Many ALDHs have been implicated in stem cell regulation where ALDH-dependent RA signaling is involved in gene expression and morphogenesis and is crucial for normal development (Figure 2) (6,12-14).
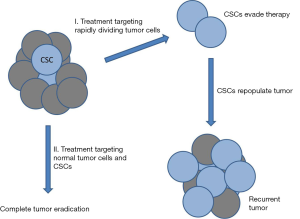

In a recent study which is the focus of this perspective, Cojoc et al. (15) examined the regulation of ALDH in prostate cancer stem cells (PCSCs) and its role in prostate tumor radioresistance. One of the major factors contributing to prostate cancer patient mortality is relapse following radiotherapy (16); therefore, it is crucial to identify the mechanisms that surviving cells, presumably CSCs, utilize to evade this lethal treatment and reestablish the tumor. The primary translational goal of this study was to evaluate ALDH activity as an effective prognostic biomarker for prostate cancer curability. Prostate cancer is the second most common cancer in American men with approximately 15% diagnosed during their lifetime (17). More than 26,000 American men die each year, and the majority of those from recurrent or metastatic disease. Thus, it is important to diagnose those at higher risk for secondary tumors and develop new treatments to combat this problem. Despite CSCs typically being lumped together as one entity, there are many variables among CSCs, even from the same tumor type, that can alter efficacy of radiotherapy as well as chemotherapeutic treatments (18). Currently, PCSCs are typically characterized by CD44+, CD133+, integrin α2β1+, ALDHhi cells (19,20). Identifying a single prognostic marker for PCSCs, such as ALDH activity, would aid in clinical evaluation.
ALDH proteins and general activity have been implicated in radiation resistance and tumor recurrence in several cancers including breast and esophageal (21,22). In order to identify genes expressed in tumor PCSCs, these cells were enriched for and isolated from a mouse xenograft tumor and genome expression analyzed (15). These cells showed a correlation between CD44+/CD133+ PCSCs and levels of ALDH1A1, ALDH3A1, ALDH3B1, and ALDH6A1; with ALDH1A1 and ALDH6A1 also being significantly enriched in prostate carcinomas compared to normal prostate tissue. Consistent with previous reports (20,23), ALDH+ cells had increased capacity to form spheres and colonies and had significantly greater frequency of tumor-initiating cells. ALDH+ cells were capable of regenerating prostate cancer tumor cell types in culture and in mouse xenograft. This confirms and expands upon the findings from earlier studies indicating that ALDH activity and expression of ALDH1A1 both correlate with tumor stage and patient survival and tumorigenicity and metastasis in animal models (24,25). Together this indicates that ALDH, or at least ALDH1A1, may be useful as a marker for PCSCs.
In order to examine the relation between ALDH and irradiation, prostate cancer cell lines and primary prostate tumor cells were exposed to varying doses of X-rays (15). There was a dose-dependent increase in ALDH activity and expression of CSC markers and β-catenin in the cancer cells but not in normal, immortalized prostate epithelial cell line (RWPE-1). This is consistent with previous studies showing irradiation triggers enrichment of CSC populations (22,26). Interestingly, prostate cells selected for enhanced radioresistance maintained greater ALDH activity and stem cell marker expression, and exhibited a higher baseline activation of DNA damage response (DDR) as indicated by phosphorylation of kinases AKT (Ser 473) and CHK2 (Thr 68). These proteins activate other DDR proteins and are essential for proper regulation of the DNA damage checkpoint (27). The high basal activation of CHK2 correlated with ALDH activity in radioresistant prostate cancer cells. Upon irradiation these cells had fewer γH2AX foci after one hour and 24 hours, indicating that there were both less DNA double strand breaks formed and more efficient repair of breaks. We previously found that depleting ALDH1A1 caused decreased phosphorylation/activation of CHK1 (Ser 317), PARP and Fanconi Anemia DNA repair pathway proteins and increased γH2AX levels (28) in response to carboplatin treatment in ovarian cancer cells lines. Loss of ALDH1A1 also disrupted the cell cycle profile of ovarian cancer cells. These findings indicate that ALDH1A1 enhances activation of DNA strand break resistance and repair, suggesting an important role for this protein in resistance to DNA damage induced by X-ray irradiation and certain chemotherapeutic drugs.
It appears, however, that enhanced DNA repair may only be part of the story. Radioresistant prostate cancer cells had lower baseline ROS, and ALDH+-derived xenograft tumor cells had high ALDH1A1 levels and low ROS production (15). ROS production is stimulated by irradiation and causes DNA damage (29-31). Interestingly, ROS-induced DNA damage has been implicated in gene regulation (32,33); thus it would be interesting to determine how ALDHs affect transcription of genes regulated in this manner. CSCs also appear to be protected from radiation-induced DNA damage by residing in a hypoxic niche inside prostate tumors, as ALDH1A1 and HIF1α expression correlated (15). The combination of protective niche, increased DNA repair and decreased ROS levels would be effective to protect ALDH+ PCSCs from the effects of anti-tumor radiotherapy.
Multiple WNT proteins and the pathway effector transcription factor, β-catenin, were found to be upregulated in irradiated prostate cancer cells (15). The WNT signaling pathway has been implicated in carcinogenesis and in maintenance of CSCs in many cancers and correlates with epithelial mesenchymal transition (EMT), metastasis and resistance to treatment (34-36). Furthermore, CSCs that survive radiation treatment display characteristics of EMT (37-40). The current study found that ALDH+ cells had both higher levels of β-catenin and a higher percentage in the nucleus where it functions, than were seen in ALDH- cells (15). To determine if the WNT pathway regulates expression of ALDHs, cells were treated with the tankyrase inhibitor XAV939 which stimulates degradation of β-catenin or siRNA targeting β-catenin. Inhibiting the WNT pathway diminished ALDH+ population and induced radiosensitization in multiple prostate cancer cell lines. There was also a concomitant loss of ALDH1A1 expression, and examination of the ALDH1A1 promoter region yielded two consensus β-catenin/TCF recognition sites. Chromatin immunoprecipitation analysis found that the β-catenin/TCF transcriptional complex binds the ALDH1A1 promoter and a luciferase reporter assay indicated β-catenin directly promotes expression from the ALDH1A1 promoter. This is an important finding as WNT/β-catenin is considered a high potential target for chemotherapy (41,42). Another regulatory pathway controlling expression of ALDH1A1 was recently elucidated by Alam et al. They found that in breast cancer cells the oncogenic MUC1-C induces the extracellular signal-regulated kinase (ERK) signaling pathway leading to phosphorylation and activation of the transcriptional regulator C/EBPβ (43). A complex of MUC1-C and C/EBPβ then binds to the ALDH1A1 promoter and promotes expression. This indicates that there are multiple regulatory mechanisms promoting expression of ALDH1A1 in cancer cells making it difficult to target its expression as a cancer treatment.
The findings of this study indicate that the WNT signaling pathway regulates ALDH1A1 expression and that ALDH activity promotes a CSC phenotype and resistance to radiation through enhanced DNA repair and decreased ROS (15). PCSCs with high ALDH activity are very tumorigenic and can reproduce multiple tumor cell types, suggesting that these cells are likely to evade radiotherapy and promote tumor recurrence. The evidence presented strongly suggests that ALDH is a likely prognostic indicator for efficacy of radiation treatment and susceptibility for prostate cancer recurrence and should be thoroughly examined in clinical trials. This is consistent with studies indicating that ALDH activity or expression of one or more ALDH protein correlate with tumor aggressiveness, treatment resistance, metastasis, and poor patient prognosis (6,25,44). ALDH has been proposed as a prognostic marker for many cancers including lung (45), breast (46), ovarian (47), pancreatic (24), and colon (48). There are many excellent recent reviews of ALDHs in cancer and CSCs (6,49-52) and these topics will not be discussed in detail here. ALDH has been most extensively studied in breast cancer where it correlates with tumor grade, ER negativity, HER2 positivity and poor patient survival (44,53,54). ALDH1+ breast cancer cells are at least 100 fold more tumorigenic than ALDH- cells in mice (44). Curiously, unlike most other tumors studied, in melanoma, high ALDH activity actually correlates with good patient prognosis (55). ALDHs have been proposed as a universal marker for identification for both normal stem cells as well as CSCs (6). And ALDH activity is commonly utilized to enrich stem cell populations from tumors, cell lines and normal tissues (24,56).
While ALDH appears to be an effective prognostic marker for many tumors, the important question remains: is ALDH a good therapeutic target for eliminating CSCs and preventing tumor recurrence? This remains a controversial subject for several reasons. Firstly, many of the studies examining the roles of ALDHs failed to identify specific proteins involved. There are 19 different ALDHs and most studies have not examined the roles of individual proteins. This is due to a lack of specific antibodies to differentiate the ALDHs and the ease and widespread use of the ALDEFLUOR assay, a simple and rapid technique to monitor and isolate cells by ALDH activity using FACS. Multiple ALDH isoforms can oxidize the substrate used in this assay and therefore, it is impossible to determine which enzymes are contributing to measured activity, and many ALDHs are not measured by this method (57). Therefore, more detailed, in depth analysis needs to be conducted to determine which ALDHs are the primary contributors to stemness, treatment resistance, tumor proliferation and aggression and metastasis; which may be tumor type or even patient specific. There is also evidence suggesting significant overlap between ALDHs and thus inhibiting one could result in others compensating for its activity (58). Furthermore, ALDHs are widely distributed throughout the body, have vital functions, and are highly expressed in certain normal organ tissues such as the liver and kidney (6,50) making it more difficult to specifically target tumors.
Recently, Hurley has had some early success designing specific small molecule inhibitors for ALDH1A1, ALDH2, and ALDH3A1 (59), indicating that this strategy is possible. ALDH1A1 has been frequently identified as being clinically relevant and as a potential chemotherapeutic target (6). Inhibiting ALDH1 has been shown to sensitize ALDHhi breast cells to paclitaxel and epirubicin (22) indicating it is a plausible target. Interestingly, ALDH1A1 promotes expression of the kinase NEK2, which directly increases the activity of multidrug efflux pumps (3). These proteins are expressed at high levels in CSCs and protect cells by removal of xenobiotic compounds, including many chemotherapeutic agents and serve as a primary cause of chemoresistance in CSCs (60). This further complicates targeting ALDH1A1 as many drugs may be ineffective because they cannot reach their intended target.
One particularly intriguing way to target ALDHs involves treatment with vitamin A-related compounds, known as retinoids. RA signaling is derived from vitamin A (retinol), crucial for embryogenesis and development (61) and functions in cellular proliferation, differentiation, and apoptosis and survival (6,49,51). ALDHs convert retinaldehyde to RA. RA is the signaling effector molecule and binds retinoic acid receptors (RAR) or retinoid X receptors (RXR) (62), which then bind specific DNA sequences in promoters to regulate gene expression (13,63). Several retinoids have previously been investigated for treating cancers to increase effectiveness of standard chemotherapy (64-66). These drugs activate RA signaling which decreases expression of stemness markers promoting cellular differentiation, promotes cell cycle arrest and decreases cellular proliferation and reduces tumor growth in mice (67).
All-trans retinoic acid (ATRA) is an RA signaling molecule that has been extensively tested as a therapeutic agent. It decreases ALDH1A1 and ALDH3A1 activity and drives differentiation of CSCs (68). ATRA was approved by the United States Food and Drug Administration for acute promyelocytic leukemia (APL) in 1995 (69). Combining ATRA with standard chemotherapy has led to a greater than 90% remission rate for AML (70) and is considered a major success story. Sadly, studies using ATRA and other RA compounds for various tumors have thus far yielded mixed results (49), likely due to high incidence of acquired retinoid resistance seen in many solid tumors. However, the limited success achieved using RAs in combination with other chemotherapeutic drugs is sufficient to warrant further studies.
There have been a few studies suggesting that certain dietary compounds are capable of targeting ALDH+ tumors and CSCs. Circumin and piperine are natural chemicals produced in plants and can be found in various foods including turmeric and black pepper, respectively. Treating breast cancer cells with these compounds led to a decrease in the percentage of ALDH+ cells and impaired mammosphere formation (a measure of stemness in breast cancer cells) (71). Sulforaphane is an isothiocyanate (ITC) compound found in many cruciferous vegetable, such as broccoli, cabbage and Brussels sprouts that also acts to reduce numbers of ALDH+ cells and mammosphere formation and slow tumor growth in breast cancer (72). These three compounds have been extensively tested and are still actively being examined as anticancer agents and have some potential for clinical applications (73).
While current cancer treatment strategies, including surgical resection, chemotherapy and radiation are quite effective at eliminating the primary tumor, many patients experience disease relapse or metastasis. It is these secondary tumors which are now responsible for the majority of cancer deaths in the United States. CSCs are typically highly resistant to anticancer treatments and are thought to be responsible for most cases of tumor recurrence and metastasis. It is significant that several recent studies have found that treating cancer cells with X-rays or chemotherapeutic agents can actually increase the expression of stem cell markers and associated stemness characteristics in surviving cells (22,26,74,75). This strongly indicates that CSCs are a roadblock to successful tumor resolution and a major threat to patient survival. To this end, there is currently a great deal of work investigating new drugs that can kill CSCs or trigger their differentiation into normal tumor cells which can then be targeted by conventional treatments (Figure 1). ALDHs are a family of proteins that are highly expressed in both normal stem cells and CSCs and important for regulating differentiation of these cells, making them intriguing targets. ALDHs appear to have great value as prognostic indicators for tumor aggressiveness, resistance and survival. While there have been some preliminary success using drugs that regulate ALDHs or retinoic acid signaling, much work remains to be done to develop effective chemotherapeutic drugs to improve patient survival. Variations in different ALDHs, tissues, tumors and other conditions will have to be addressed in order to maximize the efficiency of drugs targeting these proteins of the RA signaling pathway in which they function. The excellent studies by Cojoc et al. (15) discussed here and others bring clarity to a complex and murky field and may someday benefit many cancer patients.
Acknowledgements
Funding: The Palle lab is supported by the Abraham Mitchell Cancer Research Scholar Endowment and by National Institutes of Health grant (R01GM098956).
Footnote
Conflicts of Interest: The authors have no conflicts of interest to declare.
References
- Bonnet D, Dick JE. Human acute myeloid leukemia is organized as a hierarchy that originates from a primitive hematopoietic cell. Nat Med 1997;3:730-7. [Crossref] [PubMed]
- Wicha MS, Liu S, Dontu G. Cancer stem cells: an old idea--a paradigm shift. Cancer Res 2006;66:1883-90; discussion 1895-6.
- Abdullah LN, Chow EK. Mechanisms of chemoresistance in cancer stem cells. Clin Transl Med 2013;2:3. [Crossref] [PubMed]
- Khan IN, Al-Karim S, Bora RS, et al. Cancer stem cells: a challenging paradigm for designing targeted drug therapies. Drug Discov Today 2015;20:1205-16. [Crossref] [PubMed]
- Vasiliou V, Nebert DW. Analysis and update of the human aldehyde dehydrogenase (ALDH) gene family. Hum Genomics 2005;2:138-43. [PubMed]
- Pors K, Moreb JS. Aldehyde dehydrogenases in cancer: an opportunity for biomarker and drug development? Drug Discov Today 2014;19:1953-63. [Crossref] [PubMed]
- Singh S, Brocker C, Koppaka V, et al. Aldehyde dehydrogenases in cellular responses to oxidative/electrophilic stress. Free Radic Biol Med 2013;56:89-101. [Crossref] [PubMed]
- Chen Y, Thompson DC, Koppaka V, et al. Ocular aldehyde dehydrogenases: protection against ultraviolet damage and maintenance of transparency for vision. Prog Retin Eye Res 2013;33:28-39. [Crossref] [PubMed]
- Kiefer FW, Orasanu G, Nallamshetty S, et al. Retinaldehyde dehydrogenase 1 coordinates hepatic gluconeogenesis and lipid metabolism. Endocrinology 2012;153:3089-99. [Crossref] [PubMed]
- Marchitti SA, Deitrich RA, Vasiliou V. Neurotoxicity and metabolism of the catecholamine-derived 3,4-dihydroxyphenylacetaldehyde and 3,4-dihydroxyphenylglycolaldehyde: the role of aldehyde dehydrogenase. Pharmacol Rev 2007;59:125-50. [Crossref] [PubMed]
- Ambroziak W, Pietruszko R. Metabolic role of aldehyde dehydrogenase. Adv Exp Med Biol 1993;328:5-15. [Crossref] [PubMed]
- Chanda B, Ditadi A, Iscove NN, et al. Retinoic acid signaling is essential for embryonic hematopoietic stem cell development. Cell 2013;155:215-27. [Crossref] [PubMed]
- Duester G, Mic FA, Molotkov A. Cytosolic retinoid dehydrogenases govern ubiquitous metabolism of retinol to retinaldehyde followed by tissue-specific metabolism to retinoic acid. Chem Biol Interact 2003;143-144:201-10. [Crossref] [PubMed]
- Appel B, Eisen JS. Retinoids run rampant: multiple roles during spinal cord and motor neuron development. Neuron 2003;40:461-4. [Crossref] [PubMed]
- Cojoc M, Peitzsch C, Kurth I, et al. Aldehyde Dehydrogenase Is Regulated by β-Catenin/TCF and Promotes Radioresistance in Prostate Cancer Progenitor Cells. Cancer Res 2015;75:1482-94. [Crossref] [PubMed]
- Agarwal PK, Sadetsky N, Konety BR, et al. Treatment failure after primary and salvage therapy for prostate cancer: likelihood, patterns of care, and outcomes. Cancer 2008;112:307-14. [Crossref] [PubMed]
- SEER Cancer Statistics Review (CSR) 1975-2013. Available online: https://seer.cancer.gov/csr/1975_2013/
- Tang DG. Understanding cancer stem cell heterogeneity and plasticity. Cell Res 2012;22:457-72. [Crossref] [PubMed]
- Collins AT, Berry PA, Hyde C, et al. Prospective identification of tumorigenic prostate cancer stem cells. Cancer Res 2005;65:10946-51. [Crossref] [PubMed]
- Tang DG, Patrawala L, Calhoun T, et al. Prostate cancer stem/progenitor cells: identification, characterization, and implications. Mol Carcinog 2007;46:1-14. [Crossref] [PubMed]
- Ajani JA, Wang X, Song S, et al. ALDH-1 expression levels predict response or resistance to preoperative chemoradiation in resectable esophageal cancer patients. Mol Oncol 2014;8:142-9. [Crossref] [PubMed]
- Croker AK, Allan AL. Inhibition of aldehyde dehydrogenase (ALDH) activity reduces chemotherapy and radiation resistance of stem-like ALDHhiCD44+ human breast cancer cells. Breast Cancer Res Treat 2012;133:75-87. [Crossref] [PubMed]
- Yu C, Yao Z, Dai J, et al. ALDH activity indicates increased tumorigenic cells, but not cancer stem cells, in prostate cancer cell lines. In Vivo 2011;25:69-76. [PubMed]
- Rasheed ZA, Yang J, Wang Q, et al. Prognostic significance of tumorigenic cells with mesenchymal features in pancreatic adenocarcinoma. J Natl Cancer Inst 2010;102:340-51. [Crossref] [PubMed]
- Li T, Su Y, Mei Y, et al. ALDH1A1 is a marker for malignant prostate stem cells and predictor of prostate cancer patients' outcome. Lab Invest 2010;90:234-44. [Crossref] [PubMed]
- Ghisolfi L, Keates AC, Hu X, et al. Ionizing radiation induces stemness in cancer cells. PLoS One 2012;7:e43628. [Crossref] [PubMed]
- Liu Q, Turner KM, Alfred Yung WK, et al. Role of AKT signaling in DNA repair and clinical response to cancer therapy. Neuro Oncol 2014;16:1313-23. [Crossref] [PubMed]
- Meng E, Mitra A, Tripathi K, et al. ALDH1A1 maintains ovarian cancer stem cell-like properties by altered regulation of cell cycle checkpoint and DNA repair network signaling. PLoS One 2014;9:e107142. [Crossref] [PubMed]
- Yamamori T, Yasui H, Yamazumi M, et al. Ionizing radiation induces mitochondrial reactive oxygen species production accompanied by upregulation of mitochondrial electron transport chain function and mitochondrial content under control of the cell cycle checkpoint. Free Radic Biol Med 2012;53:260-70. [Crossref] [PubMed]
- Wiseman H, Halliwell B. Damage to DNA by reactive oxygen and nitrogen species: role in inflammatory disease and progression to cancer. Biochem J 1996;313:17-29. [Crossref] [PubMed]
- Jena NR. DNA damage by reactive species: Mechanisms, mutation and repair. J Biosci 2012;37:503-17. [Crossref] [PubMed]
- Gillespie MN, Pastukh VM, Ruchko MV. Controlled DNA "damage" and repair in hypoxic signaling. Respir Physiol Neurobiol 2010;174:244-51. [Crossref] [PubMed]
- Pastukh V, Roberts JT, Clark DW, et al. An oxidative DNA "damage" and repair mechanism localized in the VEGF promoter is important for hypoxia-induced VEGF mRNA expression. Am J Physiol Lung Cell Mol Physiol 2015;309:L1367-75. [PubMed]
- Cadigan KM. Wnt signaling--20 years and counting. Trends Genet 2002;18:340-2. [Crossref] [PubMed]
- Miki T, Yasuda SY, Kahn M. Wnt/β-catenin signaling in embryonic stem cell self-renewal and somatic cell reprogramming. Stem Cell Rev 2011;7:836-46. [Crossref] [PubMed]
- Krasnapolski MA, Todaro LB, de Kier Joffé EB. Is the epithelial-to-mesenchymal transition clinically relevant for the cancer patient? Curr Pharm Biotechnol 2011;12:1891-9. [Crossref] [PubMed]
- Abell AN, Johnson GL. Implications of Mesenchymal Cells in Cancer Stem Cell Populations: Relevance to EMT. Curr Pathobiol Rep 2014;2:21-26. [Crossref] [PubMed]
- Ahmed N, Abubaker K, Findlay J, et al. Epithelial mesenchymal transition and cancer stem cell-like phenotypes facilitate chemoresistance in recurrent ovarian cancer. Curr Cancer Drug Targets 2010;10:268-78. [Crossref] [PubMed]
- Theys J, Jutten B, Habets R, et al. E-Cadherin loss associated with EMT promotes radioresistance in human tumor cells. Radiother Oncol 2011;99:392-7. [Crossref] [PubMed]
- Tiwari N, Gheldof A, Tatari M, et al. EMT as the ultimate survival mechanism of cancer cells. Semin Cancer Biol 2012;22:194-207. [Crossref] [PubMed]
- Takahashi-Yanaga F, Kahn M. Targeting Wnt signaling: can we safely eradicate cancer stem cells? Clin Cancer Res 2010;16:3153-62. [Crossref] [PubMed]
- King TD, Suto MJ, Li Y. The Wnt/β-catenin signaling pathway: a potential therapeutic target in the treatment of triple negative breast cancer. J Cell Biochem 2012;113:13-8. [Crossref] [PubMed]
- Alam M, Ahmad R, Rajabi H, et al. MUC1-C oncoprotein activates ERK→C/EBPβ signaling and induction of aldehyde dehydrogenase 1A1 in breast cancer cells. J Biol Chem 2013;288:30892-903. [Crossref] [PubMed]
- Ginestier C, Hur MH, Charafe-Jauffret E, et al. ALDH1 is a marker of normal and malignant human mammary stem cells and a predictor of poor clinical outcome. Cell Stem Cell 2007;1:555-67. [Crossref] [PubMed]
- Liu J, Xiao Z, Wong SK, et al. Lung cancer tumorigenicity and drug resistance are maintained through ALDH(hi)CD44(hi) tumor initiating cells. Oncotarget 2013;4:1698-711. [Crossref] [PubMed]
- Li X, Lewis MT, Huang J, et al. Intrinsic resistance of tumorigenic breast cancer cells to chemotherapy. J Natl Cancer Inst 2008;100:672-9. [Crossref] [PubMed]
- Kuroda T, Hirohashi Y, Torigoe T, et al. ALDH1-high ovarian cancer stem-like cells can be isolated from serous and clear cell adenocarcinoma cells, and ALDH1 high expression is associated with poor prognosis. PLoS One 2013;8:e65158. [Crossref] [PubMed]
- Lugli A, Iezzi G, Hostettler I, et al. Prognostic impact of the expression of putative cancer stem cell markers CD133, CD166, CD44s, EpCAM, and ALDH1 in colorectal cancer. Br J Cancer 2010;103:382-90. [Crossref] [PubMed]
- Januchowski R, Wojtowicz K, Zabel M. The role of aldehyde dehydrogenase (ALDH) in cancer drug resistance. Biomed Pharmacother 2013;67:669-80. [Crossref] [PubMed]
- Alison MR, Guppy NJ, Lim SM, et al. Finding cancer stem cells: are aldehyde dehydrogenases fit for purpose? J Pathol 2010;222:335-44. [Crossref] [PubMed]
- Tomita H, Tanaka K, Tanaka T, et al. Aldehyde dehydrogenase 1A1 in stem cells and cancer. Oncotarget 2016;7:11018-32. [PubMed]
- Xu X, Chai S, Wang P, et al. Aldehyde dehydrogenases and cancer stem cells. Cancer Lett 2015;369:50-7. [Crossref] [PubMed]
- Zhou L, Jiang Y, Yan T, et al. The prognostic role of cancer stem cells in breast cancer: a meta-analysis of published literatures. Breast Cancer Res Treat 2010;122:795-801. [Crossref] [PubMed]
- Morimoto K, Kim SJ, Tanei T, et al. Stem cell marker aldehyde dehydrogenase 1-positive breast cancers are characterized by negative estrogen receptor, positive human epidermal growth factor receptor type 2, and high Ki67 expression. Cancer Sci 2009;100:1062-8. [Crossref] [PubMed]
- Prasmickaite L, Engesaeter BØ, Skrbo N, et al. Aldehyde dehydrogenase (ALDH) activity does not select for cells with enhanced aggressive properties in malignant melanoma. PLoS One 2010;5:e10731. [Crossref] [PubMed]
- Corti S, Locatelli F, Papadimitriou D, et al. Identification of a primitive brain-derived neural stem cell population based on aldehyde dehydrogenase activity. Stem Cells 2006;24:975-85. [Crossref] [PubMed]
- Moreb JS, Ucar D, Han S, et al. The enzymatic activity of human aldehyde dehydrogenases 1A2 and 2 (ALDH1A2 and ALDH2) is detected by Aldefluor, inhibited by diethylaminobenzaldehyde and has significant effects on cell proliferation and drug resistance. Chem Biol Interact 2012;195:52-60. [Crossref] [PubMed]
- Marcato P, Dean CA, Giacomantonio CA, et al. Aldehyde dehydrogenase: its role as a cancer stem cell marker comes down to the specific isoform. Cell Cycle 2011;10:1378-84. [Crossref] [PubMed]
- Kimble-Hill AC, Parajuli B, Chen CH, et al. Development of selective inhibitors for aldehyde dehydrogenases based on substituted indole-2,3-diones. J Med Chem 2014;57:714-22. [Crossref] [PubMed]
- Han L, Shi S, Gong T, et al. Cancer stem cells: therapeutic implications and perspectives in cancer therapy. Acta Pharm Sin B 2013;3:65-75. [Crossref]
- Haselbeck RJ, Hoffmann I, Duester G. Distinct functions for Aldh1 and Raldh2 in the control of ligand production for embryonic retinoid signaling pathways. Dev Genet 1999;25:353-64. [Crossref] [PubMed]
- Alvarez S, Germain P, Alvarez R, et al. Structure, function and modulation of retinoic acid receptor beta, a tumor suppressor. Int J Biochem Cell Biol 2007;39:1406-15. [Crossref] [PubMed]
- Elizondo G, Medina-Díaz IM, Cruz R, et al. Retinoic acid modulates retinaldehyde dehydrogenase 1 gene expression through the induction of GADD153-C/EBPbeta interaction. Biochem Pharmacol 2009;77:248-57. [Crossref] [PubMed]
- Formelli F, Cleris L. Synthetic retinoid fenretinide is effective against a human ovarian carcinoma xenograft and potentiates cisplatin activity. Cancer Res 1993;53:5374-6. [PubMed]
- Shalinsky DR, Bischoff ED, Gregory ML, et al. Enhanced antitumor efficacy of cisplatin in combination with ALRT1057 (9-cis retinoic acid) in human oral squamous carcinoma xenografts in nude mice. Clin Cancer Res 1996;2:511-20. [PubMed]
- Pettersson F, Colston KW, Dalgleish AG. Retinoic acid enhances the cytotoxic effects of gemcitabine and cisplatin in pancreatic adenocarcinoma cells. Pancreas 2001;23:273-9. [Crossref] [PubMed]
- Ying M, Wang S, Sang Y, et al. Regulation of glioblastoma stem cells by retinoic acid: role for Notch pathway inhibition. Oncogene 2011;30:3454-67. [Crossref] [PubMed]
- Moreb JS, Gabr A, Vartikar GR, et al. Retinoic acid down-regulates aldehyde dehydrogenase and increases cytotoxicity of 4-hydroperoxycyclophosphamide and acetaldehyde. J Pharmacol Exp Ther 2005;312:339-45. [Crossref] [PubMed]
- Tallman MS, Andersen JW, Schiffer CA, et al. All-trans-retinoic acid in acute promyelocytic leukemia. N Engl J Med 1997;337:1021-8. [Crossref] [PubMed]
- Sanz MA, Lo-Coco F. Modern approaches to treating acute promyelocytic leukemia. J Clin Oncol 2011;29:495-503. [Crossref] [PubMed]
- Kakarala M, Brenner DE, Korkaya H, et al. Targeting breast stem cells with the cancer preventive compounds curcumin and piperine. Breast Cancer Res Treat 2010;122:777-85. [Crossref] [PubMed]
- Li Y, Zhang T, Korkaya H, et al. Sulforaphane, a dietary component of broccoli/broccoli sprouts, inhibits breast cancer stem cells. Clin Cancer Res 2010;16:2580-90. [Crossref] [PubMed]
- Scarpa ES, Ninfali P. Phytochemicals as Innovative Therapeutic Tools against Cancer Stem Cells. Int J Mol Sci 2015;16:15727-42. [Crossref] [PubMed]
- Hermann PC, Huber SL, Herrler T, et al. Distinct populations of cancer stem cells determine tumor growth and metastatic activity in human pancreatic cancer. Cell Stem Cell 2007;1:313-23. [Crossref] [PubMed]
- Dylla SJ, Beviglia L, Park IK, et al. Colorectal cancer stem cells are enriched in xenogeneic tumors following chemotherapy. PLoS One 2008;3:e2428. [Crossref] [PubMed]