Surgical applications of three-dimensional printing: a review of the current literature & how to get started
Introduction
Three dimensional printing technology has been adopted by surgeons at an impressive rate and in a large variety of applications. Nearly every part of human anatomy that can be operated on has had a 3D model printed of it. Furthermore, surgeons have gone beyond printing these impressive patient-specific anatomic models to printing patient-specific medical hardware, such as implants, prosthetics, external fixators, splints, surgical instrumentation, and surgical cutting guides. The recent explosion in popularity of 3D printing is a testament to the promise of this technology and its profound utility in surgery.
Historically, the concept of using 3D medical imaging, specifically computed tomography (CT) data, to reconstruct a physical model was first suggested in 1979 (1). At that time, there were no rapid prototyping (RP)/3D printing systems available, however subtractive manufacturing, or milling, was a possibility. Prior to the advent of 3D printers the main method of fabricating a unique part for prototyping was with the use of Computer Numerical Controlled (CNC) machine. The computer controls the tools needed for fabrication of the part by controlling the lathes, mills routers, and grinders used in the process. This technique usually starts with a blank block of material that is slowly shaped into the final part. However, sometimes there is additional work done on the part by hand to further refine it. The first anatomic model constructed with the use of medical imaging was done that same year. It was a model pelvis that was milled from a polystyrene block (2). With the introduction of the first commercial 3D printing machine in addition to increasing access to 3D medical imaging techniques in the late 1980s, applications for 3D printing in the medical field began to be seriously considered. Stereolithography (SLA) is the technique by which a computer controlled laser been is used to harden a liquid polymer or resin, creating a structure layer by layer. SLA was the first 3D printing technique available and the first used in the biomedical field, in 1994 (3). Orthopedic surgery and Oral and Maxillofacial surgery were among the first specialties to adopt this technology. It has been suggested this is largely because 3D printing is more suitable to fields that handle hard tissue since the first 3D printers could only print with hard materials (4). Currently, per our review of the literature, nearly every surgical specialty has published applications for 3D printing.
Rapid prototyping (RP) is an additive manufacturing technique that works by building a model from the ground up, depositing material layer by layer. It was first used in the late 1980s and was developed to apply the precision and functionality of computer assisted design (CAD) to manufacturing (5). With this technology, a prototype could be quickly produced that accurately represented the engineers draft. Furthermore, if changes needed to be made, the engineer could easily digitally alter the draft and print the redesigned part. This concept was quickly adopted by the aircraft and automobile industries for the rapid production of prototype parts. Hence the name, RP. The first commercial three-dimensional (3D) printing machine was introduced in 1987, which dramatically increased access to RP. The more recent innovation in 3-D printing has been with the development of more affordable printers for hobbyists or small start-up companies. For a fraction of the cost of a large 3-D printer, one can now bring original CAD into the practical world of engineering. In 2014 the movie Print the Legend brought to light the rise of affordable 3-D printers and its wide spread use by all.
RP and 3D printing, in the medical field, requires a multidisciplinary team. Most surgeons are not familiar with the techniques involved in 3D printing and most affordable printers require some engineering background for troubleshooting and CAD design. The purpose of this review is to provide a current overview of the published applications of 3D printing in surgery as well as to provide background information that would be helpful to those interested in introducing 3D printing to their practice. We will walk through the process of 3D printing in a chronologic stepwise fashion, including imaging modalities, the use of medical imaging for 3D modeling, 3D printing techniques, available materials, and the time and cost involved in 3D printing. Finally, we will also provide a brief overview of the published surgical applications for 3D printing.
Methods
Eligibility criteria
We searched for studies that addressed the clinical application of 3D Printing in all surgical fields. Special attention was paid to identifying information that would be useful for those interested in introducing themselves to 3D Printing and taking the first steps to start using this technology. We excluded studies based on the criteria listed in Table 1.
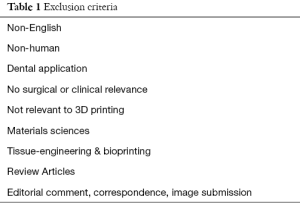
Full table
Study identification and selection
PubMed was searched on 15 May 2016 without limitation on date of publication or article type. Both Mesh terms and key terms were used to capture any publications not yet indexed. Search terms used include: “Printing, Three-Dimensional” [Mesh], “3D Printing”, “Three-Dimensional Printing”, “3DP”, and “Surgery”. A flow diagram detailing our literature search and screening is shown in Figure 1.
Data extraction
Data was manually extracted using a standardized spreadsheet. The categories of the data extracted from each study are listed in Table 2. Note that the extracted information was collected as available, as many studies did not report all or our variables of interest.
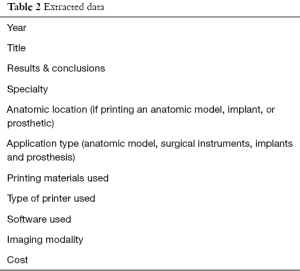
Full table
Results and discussion
Imaging modalities used
A 3D digital image is an essential part of 3D printing. The most commonly used modalities used are conventional CT and MRI. However, a number of other 3D imaging options have been used in 3D printing, such as: Cone Beam CT, CTA, MRA, PET, MRCP, 3D echocardiography, 3D laser scanning systems, and even images captured on an iPhone (6-10). However, even simple drawing and physical models can be used to render a 3D digital CAD image of the part needed. In addition, digital data can be incorporated from multiple sources to create a unique CAD models. Gillaspie et al., 2016, combined CT and PET data to produce models that depict physiologic activity in addition to anatomy. Furthermore, this group performed imaging and 3D printing over time to capture the response of a tumor to induction therapy. Gillaspie et al. refer to this as 5-Dimensional printing, since they add both time and physiologic activity the 3D model (11).
3D modeling with medical imaging data
Working with software is inherent to the process of 3D printing, since the 3D Printer requires a digital representation of whatever is to be printed. Thankfully, 3D medical imaging is a convenient way to obtain a data set for digital 3D modeling and subsequent printing. Although the steps undergone to produce a digital 3D model will vary by application, what follows is a general summary of what producing a file that can then be used by a 3D printer entails. These steps have been adapted from Lambrecht et al., 2009, since the software employed in their workflow has been shown to be some of the most commonly used software in 3D printing, both in this review and by Byrne et al., 2016 (6,12). It should be noted that free open source software is available and its use in the 3D Printing of medical instruments has previously been described by Fuller et al., 2014 (13).
- Data acquisition, this includes building a digital model either de-novo with CAD software or with the use of a 3D imagining modality. For constructing an anatomic model, CT or MRI data are typically used and the data set is characteristically stored as a DICOM (Digital Imaging and Communications in Medicine) file;
- 3D Visualization and selection. At this stage a volumetric dataset is calculated from the available DICOM data. This can be done with a number of software programs, including accuitomo software (i-Dixel images);
- Creation of a virtual 3D representation from the previously acquired 2D layers and subsequent image segmentation. Creation of 3D model from a 2D layer set is automatically done by software. Image segmentation involves the dividing of the image into distinct anatomic parts and requires that the user have an understanding of the anatomy of interest. MIMICS (Materialise’s Interactive Medical Image Control System, Materialise, Belgium) is overwhelmingly the most common program used to accomplish these steps (12). The dataset is then exported in STL (STereoLithography, Standard Triangle Language, or Standard Tessellation Language) format, which is supported by all 3D printers available (14);
- Geometric surface preparation. At this stage the virtual 3D representation is crude and the rough surfaces need to be smoothed. Other surface refinement can also be done, such as checking for and correcting any overlying surfaces, and simplifying the image file for more efficient printing;
- Print.
To state the process more simply, 3D imaging data is collected, often with a CT or MRI. The DICOM data is then converted to an SLA file. In the SLA format, the image can undergo segmentation and surface preparation. See Figure 2 for a graphic representation of this process and our recommendations. The SLA file is then suitable to be used by a 3D printer. The use of CAD to design de novo parts is beyond the scope of this review and not discussed.
Printing techniques
There are a number of RP techniques now used. A brief summary of RP techniques is shown in Table 3.
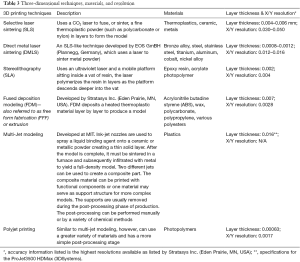
Full table
In addition to the additive manufacturing techniques described in Table 3, subtractive manufacturing should also be mentioned. In contrast to additive manufacturing, where material is selectively deposited to build a model, subtractive manufacturing employs milling, or the removal of material from a pre-existing block, typically of polystyrene. Although subtractive manufacturing uses less costly materials, additive manufacturing is superior in both geometric accuracy and versatility (15). With milling techniques, the interior of the model is always solid. With RP, the designer has control over the internal structure (5). This is relevant, for example, in the RP of surgical instruments. It has been shown that instruments with a honeycombed internal structure are more durable than their solid counterpart. Additionally, having control over the internal structure of the model means that models can be designed with an internal structure that requires less material and printing time (16).
Materials used in 3D printing
The materials commonly used in 3D printing are listed in Table 3. Additionally, commercial 3D printing system manufactures provide a number of variations of these materials that can differ in color, density, flexibility, texture, durability, and tensile strength. The point is that there exists a large palate of potential materials. In addition to the materials already discussed, however, it is worth noting the use of materials novel to 3D printing. In a study by Okumoto et al., 2015, craniofacial bone models were printed with salt by a 3D inkjet printer (17). Their goal was to create a 3D printed bone model that closely represented the tactile sensation of human bone when cut. They found that models printed with salt accurately reproduced the sensation.
Sterilization of the printed model is a common concern. Nevertheless, most of the materials used in 3D printing are compatible with acceptable sterilization methods for surgical instruments as listed by the Center for Disease Control (CDC), such as steam sterilization and low temperature techniques like hydrogen peroxide, peracetic acid, and ethylene glycol. For example, although polylactic acid (PLA) is a thermoplastic, it has a high-melting point (250 °C) and can therefore be subjected to steam sterilization (121 °C) (16). Additionally, it has been demonstrated that 3D printed PLA models can be sufficiently sterilized by immersion in glutaraldehyde (16). There are, however, practical limitations regarding a few materials, for example, some thermoplastics have prohibitively low melting temperatures. Regardless of some of these minor limitations, given the range of available printing materials, that many are compatible with current sterilization methods, and the numerous examples of the intra-operative use of 3D printed models, sterilization of 3D printing materials does not appear to be a barrier to intra-operative use.
More than the sterilization of anatomic models or surgical instruments, the sterilization of implants may be of particular concern. Adequate sterilization of materials used for implantation is possible, however, may present an additional regulatory hurdle. It has been suggested that implantable devices may need to be validated on a case-by case basis and will likely require consultation from the FDA (18). Although the variety of printing materials and model architecture can present a challenge, the sterilization of materials not traditionally implanted has been reported (19). Even more so, this problem is negated when implants are 3D printed with metal, as they were in the majority of studies reviewed.
Occasionally, there are applications that call for a material that is not compatible with 3D printing but still requires the use of CAD. This problem can be solved by printing a model of the desired implant, using that model to create a mold, and then using the mold to create the true implant out of the desired material (20).
Cost
Most 3D printing systems are now off-patent, therefore, the cost of these systems has been recently decreasing (3). The use of a commercial service for 3D printing is not uncommon and can be cost effective especially with low volume printing. With high volume printing, it is advantageous to purchase a printing system since although the initial cost of the printing system and software is high, the cost associated with each unit can be relatively low (4). 3D Printing systems used for surgical applications range in cost, beginning at $2,000 for entry level FDM, SLA, and PolyJet systems to $150,000–$900,000 for the most accurate SLS, FDM, SLA, and PolyJet systems. (14). Interestingly, a new kickstarter campaign is offering a SLA 3D printer for $100. Software costs range from $500–$10,000 depending on the software package. As previously mentioned, however, free open-source software is available and its use has been previously reported (13). The cost of materials is also variable. One group has printed army/navy surgical retractors from PLA with FDM for $0.46 per each PLA retractor (21). On the other hand, printing with metal can be substantially more expensive. One group spent $1,200 on the printing of a stainless steel bone reduction clamp (13).
Time requirement
The process of 3D printing can be time intensive, especially the processing of 3D imaging data and/or the design of digital models with CAD. With advancements in software and greater workflow automation, however, this process is becoming increasingly user friendly and streamlined. Furthermore, a recent review considered that the large amount of time required for 3D printing was well worth the benefit. Noting that 10 minutes saved in the operating room would be equivalent in cost to 1 hour of work spent in the production of a 3D printed object (22). Even more so, direct benefit to the patient such as less anesthesia exposure, decrease blood loss, and improved surgical outcome could potentially be invaluable (23-26).
Overview of the applications of 3D printing in surgery
The use of 3D printing in surgery has increased exponentially since 2013 (Figure 3). The variety of applications is broad and has likewise increased. Previous reviews have divided the applications of 3D printing in surgery into three categories: (I) anatomic models; (II) implants and prostheses; and (III) surgical instruments (4). In this review, we will adhere to the categories previously proposed but also expand where necessary to reflect an updated review of the literature. Our expanded categories include: (I) anatomic models; (II) implants, prostheses, splints, and external fixators; and (III) surgical instrumentation and guides.
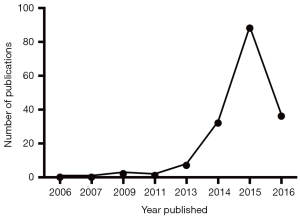
Anatomic models
Anatomic model fabrication is overwhelmingly the most common use of 3D printing in surgery. In our review, there were 126 publications reporting the use of 3D printed anatomic models compared to 18 publications on implants, prosthesis, splints, and external fixators, and 36 publications on surgical instruments and guides. The 3D printing of anatomic models is largely done for two purposes, pre-operative planning and education. Pre-operative planning is the more common use. We found that 83 studies used anatomic models for pre-operative planning compared to 36 studies that used the models for education and 7 studies that used the models for both. In the majority of these studies, 3D printing was found to be a valuable tool in both the pre-operative planning stage as well as in educating surgical residents and medical students. Below, we have comprehensively outlined how 3D printed anatomic models have been used in surgery. Figure 4 depicts the range and commonality of anatomic models that have been printed.
Anatomic models: pre-surgical planning
Preoperative planning has been heavily impacted by 3D printing. In the pre-surgical planning stage, 3D models have been used to represent vital anatomy, simulate the procedure, and pre-shape or test surgical tools. Studying a patient’s anatomy with a life size replica of the structures in hand has obvious benefit compared to viewing images on a computer monitor. A recent study compared the utility of 3D printed models to 3D rendered images for preoperative planning (27). Surgical residents were asked to develop and review either 3D computer models or 3D printed models and subsequently formulate a pre-operative plan. The residents who reviewed the 3D printed models scored significantly higher in the quality of their surgical plan. The authors concluded that the use of 3D printed models may result in improved quality of pre-operative planning for novice surgeons and be useful for improving skill outside the operating room. This is a summary of how anatomic models have been applied across the surgical specialties in pre-surgical planning.
Neurosurgeons have used anatomic models of complex skull base and craniovertebral junction deformities (28) in their planning. Within Spine surgery, models have been used to plan for corrective surgery in adolescent scoliosis patients (29). In fact, the use of 3D printed models to plan for surgery in patients with scoliosis, atlas neoplasms, and atlantoaxial dislocation have been shown to reduce operating time and intraoperative blood loss (23).
Vascular surgeons have printed patient-specific celiac trunk aneurysms (30), patient-specific aortic models to guide graft fenestration placement for branch vessels (31), and techniques have been developed to print whole aortic models that most accurately represent the patient’s anatomy (32). In this last case, the calcified plaque was digitally removed before printing to patient a more anatomically correct model.
Cardiothoracic surgeons have strongly embraced the printing of 3D models for pre-operative planning. Published applications include printing anatomic models of patient anatomy for planning for correction of congenital heart diseases, such as transposition of the great arteries, ventricular septal defect, pulmonary stenosis, and double outlet right ventricle correction (33-36). As well as printing models to prepare for primary cardiac tumor resection (11,37), septal myectomy (38), cardiac schwannoma resection (39), pediatric cardiac transplantation in a patient with a univentricular heart (40), correction of intracardiac defects (41), ectopic thymoma resection (42), complete aortic arch replacement (43), pulmonary subsegmentectomy (44), lung cancer resection (45), and complex esophageal pathology (46). The use of 3D printing in cardiothoracic surgery has been especially helpful in patients with aberrant vascular anatomy (45). Some have even sterilized the anatomic models and used them intra-operatively for anatomic orientation, for example, one group printed the vascular anatomy of a patient with a history of coronary artery bypass grafting (CABG) who was undergoing aortic valve replacement surgery for intraoperative use (47).
In hepatobiliary surgery, 3D printing has also been employed as a method of studying variant anatomy before surgery. A group printed a patient-specific life-size liver model representing tumor and variant vasculature prior to hepatectomy (48). Furthermore, 3D printed models have been used to identify hepatic tumors intraoperatively that would otherwise be invisible on ultrasound (49). In transplant surgery, the use of 3D printed models of living donor and recipient vascular and biliary tract anatomy in pre-operative planning has been efficacious (9,50).
Using 3D printed models to plan for operations involving rare or abnormal anatomy has also been used in orthopedic surgery. For example, in planning for revision lumbar discectomy, this study reported decreased operative time and intraoperative blood loss (24). Other published orthopedic applications include planning for the correction of a large scapular osteochondroma (51), pelvic surgery (52), and surgical correction of recurrent anterior shoulder instability (53).
In urology, 3D printing of anatomic models has been used for the pre-surgical planning of partial adrenalectomy (54), partial nephrectomy in the resection of renal tumors (55), and for educating patients about the anatomy of their kidney and tumor before resection (56).
Oral and maxillofacial surgeons have used 3D printing to plan for correction of orbital hypertelorism (57) and maxillary reconstruction (58). In craniofacial surgery, 3D printed models have been used to assist pre-operative flap design in the treatment of Parry-romberg syndrome (59) as well as to plan for split calvarial bone grafting (60).
Plastic Surgeons have used 3D printing in pre-operative planning to depict the boney anatomy of the thumb in various orientations (61), to assist flaps design and harvest by printing a “reverse” model of the soft tissue wound defect (62), and to print soft tissue and skeletal models of the hand used to determine the size of the graft in toe-to-hand reconstruction (63).
Anatomic models: pre-surgical simulation
In addition to using 3D printed anatomic models to simply gain a more accurate perception of the patient’s anatomy, these models have also been used to perform mock surgeries pre-operatively with patient-specific anatomy. This can be highly valuable in the planning of surgery in a patient with highly complex or rare surgical pathology (64).
In cardiothoracic surgery, published examples of 3D printed patient-specific models for pre-operative simulation included the following: complex aortic arch obstruction (65), hypoplastic aortic arch model in preparation for endovascular stenting (66), tracheoesophageal fistula model (67), lung vasculature model for thoracoscopic segmentectomy (68), models for simulation of transcatheter valve replacement (69), and airway models for simulation of airway stent insertion (70).
In pediatric surgery, published examples of pre-surgical patient specific simulations include laparoscopic choledocal cyst excision (64) and thoracoscopic infant lobectomy (71). These authors found pre-surgical simulation to be a valuable tool. These anatomic models were also used in teaching residents, who found the simulations valuable for clinical practice and as a learning tool (64).
Hepatobiliary surgeons have printed models of hepatic vasculature to simulate parts of the surgery before cholangiocarcinoma resection (72) and, in another case, hepatoblastoma resection in a child (73).
In transplant surgery, patient-specific models have been printed to simulate and plan for lobar lung transplantation (74) and kidney transplantation (75). Before lobar lung transplantation, models of the donor and recipient pulmonary vasculature were printed and all vascular anastomosis were simulated pre-operatively. In the kidney transplantation, a complete pelvic cavity replica was printed.
In orthopedic surgery, it has been found that simulating intramedullary nailing in severely bowed femurs and atypical fractures with patient-specific models can be valuable in the pre-operative stage (76).
In Otolaryngology, 3D printing has been used to print temporal bone models for otologic surgery simulation (77,78) as well as cricoid cartilage models that nearly identically represent the physical characteristics of human cricoid cartilage in vivo. The later models were used to assess the likelihood of cricoid fracture with balloon dilation procedures (79).
In plastic surgery, 3D printing has been used for simulation of auricular framework reconstruction involved in the treatment of microtia (80). The printed costal cartilage model was more representative of pediatric rib geometry and texture then the currently available simulations.
Anatomic models: pre-surgical manipulation and selection of surgical equipment
In addition to the use of 3D printed anatomic models to study the patient’s anatomy or simulate the surgery pre-operatively, patient specific anatomic models have also been used to physically shape and/or direct the appropriate choice of surgical instrumentation and implants. An example is shown in Figure 5. In general, this has been an advantageous practice since it allows the surgeon to modify or shape this equipment outside of the operating room in a controlled environment. This can further streamline the operation and decrease operative time while increasing implant or instrument accuracy.
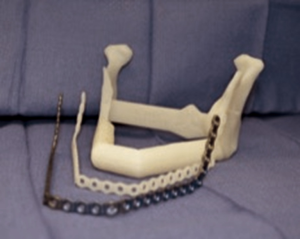
Neurosurgeons have printed aneurysm models for the purpose of forming a microcatheter shaping mandrel to line the curvature of the parent vessel. They found that the pre-operatively patient-specific shaped microcatheters matched the vessel and aneurysm anatomy intraoperatively (81).
In vascular surgery, aortic aneurysm models have been used to assist in choosing an appropriate devise for aneurysm repair (82).
In orthopedic surgery, published examples of pre-shaping titanium plates with anatomic models include use of a calcaneus model in planning for calcaneal fracture reduction (83) and clavicular models for clavicle fracture reduction (84,85).
This concept has been extensively applied in Oral Maxillofac Surg For example, published applications include printing anatomical models of a variety of cranial and facial bones to shape titanium implants (86-88), printing a skull and orbital model for the molding of synthetic scaffolds in the correction of post-traumatic orbital deformity (89), printing a mandibular defect to design a surgical guide (90,91), and using a model of the pelvis to design a surgical guide for pelvic graft harvest (92). Beyond simply using the anatomic model to physically shape the implant, these models have also been used to engineer implant structure and analyze the stress distribution transferred to the implant (93).
Published applications in craniofacial surgery include shaping titanium plates pre-operatively with models of the orbit (94), mandible (95), and skull (96). In addition to titanium plate modification, 3D models have also been used to design screws and of predetermined length to assist intra-operatively in contouring surgery in a patient with severe fibrous dysplasia (97). In craniofacial surgery anatomic models have also been used to shape a frontal sinus template to guide osteoplastic flap surgery (98). An example that takes advantage of the power of CAD in 3D printing is the use of patient-specific maxillary models that were digitally designed to predict the molding stages in the treatment of children with cleft lip and palate. The predicted anatomy was 3D printed and used to design appliances for pre-surgical nasoalveolar molding of the cleft lip and plate (99).
Anatomic models: education
3D printed anatomic models are a valuable tool in medical education, both for medical students and resident physicians. Medical students sometimes do not have access to cadaveric anatomic examples, in which these physical models can be useful. Also, these models have been useful for the teaching of anatomic pathology not commonly seen in cadaveric samples. Such as a variety of ventricular septal defects (100). 3D printed models have been especially beneficial for surgical resident education. The technology has been applied extensively to produce surgical simulations that more accurately represent the anatomic pathology encountered, the tactile feedback of truly operating, and practice operating on rare surgical pathology.
Neurosurgeons have used 3D printing for this purpose prolifically. Published examples include skull base surgery simulations with a temporal bone model (101), clipping cerebral aneurysms with a patient specific models (102-104), brain retraction with a brain model that accurately represented the consistency of brain tissue (105), endoscopic surgery simulation in a model with hydrocephalus (106), simulating ultrasound surgery (107), and use of printed models to simulate EVD placement (108). Simulating appropriate tactile sensation has also been explored in neurosurgery, a brain model with realistic tactile feedback and force transducers has been developed to train neurosurgery residents how to atraumatically retract brain tissue (109).
In general surgery, there have been a variety of publications on this topic. For example, 3D printing has been used to fabricate components for the surgical simulation of laparoscopic pyloroplasty (110), endoscopic ampullectomy (111), and EUS guided biliary drainage (7).
In the surgical training of Otolaryngology residents, temporal bone models in surgical simulation and pre-operative planning have been found to be highly useful. So much so, that there has been interest in developing a low cost model that adequately represented realistic feedback to drilling for resident training. This bone simulation model was developed and can be printed on a desktop 3D printer for as little as $1.92 per model (112). Additionally, a temporal bone model for other dissection exercises has been developed and validated (113). A similar temporal bone model representing pediatric anatomy have also been developed and found to be extremely useful given the even greater cost and low availability of pediatric cadaveric temporal bones (114). Other published examples of the use of 3D printing in training otolaryngology residents include printing representations of nasal cavity, paranasal sinuses, and intrasellar/pituitary pathology for surgical simulation (115), skull base pathology for simulated practice performing endoscopic skull base surgery (116), and development of a simulation system for mastoidectomy with 3D printed anatomic structures (117).
In oral and maxillofacial surgery, there has also been work to create models that accurately recreate tactile feedback, as previously mentioned, in this study a mandibular model was printed from salt (17).
Plastic surgeons have used cleft lip and palate models to teach chelioplasty via simulation (118). The silicone models for chelioplasty cost less than $50 and were found to be useful by the residents. Ophthalmology resident physicians have used 3D printed orbital models for anatomic instruction and surgical simulation (8,119).
Implants, prosthesis, splints, and external fixators
RP provides a unique ability to digitally customize implants, prosthetics, splints, and external fixators to be patient-specific. Furthermore, computer based surgical simulations have been used to recreate optimal surgical outcome and then design and print the appropriate hardware (120,121). This technology presents the ability to customize these devices in a way not previously possible.
In neurosurgery, published examples include the printing of a customized titanium vertebral body for reconstruction of the upper cervical spine in an adolescent with Ewing sarcoma (122). Neurosurgeons have also used 3D printing to print polymethylmethacrylate (PMMA) implants for reconstruction of skull defects (20). They found that use of the 3D printed implant resulted in low complication rate and high patient and/or parent satisfaction. In cardiothoracic surgery, titanium rib prosthetics have been 3D printed for chest wall reconstruction status post large resection of a non-small cell lung cancer (123).
This application has been especially endorsed by a variety of orthopedic surgery groups. For example, the complex anatomy of the pelvis precludes straightforward reconstructive techniques. 3D printing has been used to fill this need. A custom titanium pelvic implant, that included the acetabular cup has been 3D printed to reconstruct a complex defect status post pelvic chondrosarcoma resection (124). In fact, there has been a small-center series of 24 patients who underwent 3D printed custom acetabular cage implantation (125). The authors considered this approach efficacious and ready for evaluation in a larger population. 3D printed plates for fixation of intercondylar humeral fractures have also been used (126). Within orthopedic surgery, 3D printing has also been used to fabricate custom external fixation hardware. Computer-assisted reduction technology was used to design the hardware, including mounting hole placement, for fixation of tibial fractures (120,121). The authors named this device the ‘Q Fixator’ and found that it was easily manipulated and that it enabled a successful reduction and fixation independent of the surgeon’s experience.
In urology, they have printed ureteral stents with a rubber like material for the long-term treatment of vesicoureteral reflux (127). The stents have yet to be used in animals, but are worth noting to demonstrate the utility of CAD and 3D printing in the design, RP, and in vitro study of a stent.
In otolaryngology, customized splints have been printed for the treatment of tracheobronchomalacia in infants (19).
In oral and maxillofacial surgery, published examples include printing of a titanium mesh for reconstruction of maxillary defect (128), computer aided design and 3D printing of Cobalt-chrome alloy arch bars for the treatment of mandibular and/or maxillary fractures (129), and printing of a custom spacer for temporomandibular joint resection and reconstruction that was impregnated with antibiotics (130).
In craniofacial surgery, auricular implants have been used successfully in the ear reconstruction of those with acquired or congenital loss of the ear. Two studies have assessed the role of CAD and 3D printing in design and fabrication of these implants. It has been reported that the use of 3D printing for this application is a possibility (131). Auricular prosthesis have also been constructed with the use of CAD and 3D printing, they found that CAD and 3D printing actually saved time in the prosthetic fabrication process (132). Tissue engineering is beyond the scope of this review, however, it is worth noting that there is work to print tissue bioscaffolds impregnated with growth factors for auricular reconstruction (133). Aside for ear reconstruction, craniofacial surgeons have also used 3D printing to fabricate titanium implants for the reconstruction of large cranial defects (87). This group not only utilized 3D printing to fabricate the titanium implant, but also printed a model of the patient’s skull to assess the fit of the implant pre-operatively.
In ophthalmology, they have printed a customized ocular prosthesis (134) and intraocular lenses for implantation status post cataract surgery (135). It should be noted however, that the printed lens did not meet current clinical standards, but showed promise.
Surgical instrumentation and guides
The 3D printing of surgical instrumentation and surgical guides has great potential value. Given that it enables the use of customized surgical instruments, precise patient-specific surgical guides, and even the RP of surgical equipment in areas were acquisition of such equipment by conventional means is not a possibility. An example two surgical cutting guides are shown in Figures 6 and 7.
Neurosurgeons have used 3D printed surgical templates for fronto-orbital advancement surgery (136), which they found to be accurate, easy to use, efficient, and reproducible.
In general surgery, several have published on the printing of standard surgical instruments (16,21). Beyond this, RP has provided a platform for the fabrication of unique and complex instruments. Although having only been tested in animals thus far, it is worth noting that a group has employed CAD and 3D printing in the production of a novel endoscopic device (137). Not only did 3D printing enable the fabrication of the device, but also a quick turnaround for improvements in design.
In orthopedic surgery, patient-specific surgical guiding templates have been printed for the precise resection of an osteosarcoma (138), corrective osteotomy in treatment of a pronation deformity (139), for unicompartmental knee arthroplasties (140), and to guide midcervical pedicle screw insertion (25), screw trajectory for complicated tibial plateau fracture fixation (141), and lumbar pedicle screw fixation (26). The use of 3D printed surgical guides in orthopedic application has resulted in shortened operating time and less intra-operative blood loss.
A urology group has printed trocars, in addition to ureteral stents, for laparoscopic surgery (142).
An especially novel surgical application of CAD and 3D printing employed by urologists is worth mentioning in greater detail. The correlation between magnetic resonance imaging and the histology of prostate cancer is important for the improvement of the predictive accuracy of MRI for this disease. Preister et al., 2014, employed CAD and 3D printing to construct a mold from MRI data that would fit around the extirpated tumor. The mold contained a series of parallel slits that each correlated with an MRI slice. After the surgery, the tumor could then be sectioned in accordance with the mold and the histologic sections compared to the corresponding MRI slice for MR-histology correlation (143).
The majority of publications on 3D printing surgical instruments and guides have been in Oral Maxillofac Surgery. Perhaps because the use of surgical guides in orthognathic surgery is not uncommon. RP has been used to fabricate maxillomandibular repositioners/splints (144) in addition to a relatively large number of interocclusal wafers for orthognathic surgery that were designed with the use of CAD (145-148). From these examples, it is clear that the development of patient-specific devices in oral maxillofacial surgery has enabled even more accurate orthognathic surgery. Regarding the use of surgical guides and templates, published examples include the 3D printing of surgical templates for the treatment of osteochondroma of the mandibular condyle (149), mandibular reconstruction with a free flap (150), genioplasty (151), cutting guides for maxillary repositioning (152), cutting guides for other maxillary surgeries (153), and surgical guides for fracture reduction of the mandible (148,154) and condyle (155). 3D printed drilling guides have also been employed (156).
In craniofacial surgery, 3D printing has been used to print a mold of an orbital floor defect which was then used to sculpt an autologous bone implant (157). Patient-specific surgical guides have also been printed for mandibular osteotomy in the treatment of hemifacial macrosomia (158), mandibular distraction in the correction of mandibular hypoplasia (159), and for 3D tissue contouring in the treatment of aggressive fibrous dysplasia (160).
Additionally, in plastic surgery, 3D printing has been used to construct a bone reduction clamp for hand surgery (13) and a surgical guide for mandibular angle ostectomy (161). 3D printed surgical guides have also been adopted in ophthalmology and have proven especially useful for technically difficult surgery, such as collection of the donor cornea for Descemet stripping automated endothelial keratoplasty (162). Finally, an obstetrics and gynecology group published a proof-of-concept study to demonstrate that surgical equipment could be 3D printed, where they printed a suction tubing connector that was later used in a surgical procedure (163).
Conclusions
In this review, the diverse and increasing applications of 3D printing have been presented. In addition, we have provided a background on RP and some of the considerations when getting started with 3D printing, such as imaging, software, printing materials, sterilization of printing materials, and cost and time requirements. The implementation of 3D printing in surgery has increased exponentially, as evident by the number of publications over the past 10 years. We believe that this is a result of the impressive utility and large array of potential applications for 3D printing, in addition to decreasing cost. The road to implementing this technology in clinical practice can initially appear daunting, with the necessary use of unfamiliar software and the large number of 3D printing modalities available. With the use of a multidisciplinary team and rapid advancements in the field, incorporating 3D printing into a suitable application can be a highly rewarding process.
Acknowledgements
None.
Footnote
Conflicts of Interest: The authors have no conflicts of interest to declare.
References
- Alberti C. Three-dimensional CT and structure models. Br J Radiol 1980;53:261-2. [Crossref] [PubMed]
- Frame M, Huntley JS. Rapid prototyping in orthopaedic surgery: a user's guide. ScientificWorldJournal 2012;2012:838575.
- Kim GB, Lee S, Kim H, et al. Three-Dimensional Printing: Basic Principles and Applications in Medicine and Radiology. Korean J Radiol 2016;17:182-97. [Crossref] [PubMed]
- Malik HH, Darwood AR, Shaunak S, et al. Three-dimensional printing in surgery: a review of current surgical applications. J Surg Res 2015;199:512-22. [Crossref] [PubMed]
- McGurk M, Amis AA, Potamianos P, et al. Rapid prototyping techniques for anatomical modelling in medicine. Ann R Coll Surg Engl 1997;79:169-74. [PubMed]
- Lambrecht JT, Berndt DC, Schumacher R, et al. Generation of three-dimensional prototype models based on cone beam computed tomography. Int J Comput Assist Radiol Surg 2009;4:175-80. [Crossref] [PubMed]
- Dhir V, Itoi T, Fockens P, et al. Novel ex vivo model for hands-on teaching of and training in EUS-guided biliary drainage: creation of "Mumbai EUS" stereolithography/3D printing bile duct prototype (with videos). Gastrointest Endosc 2015;81:440-6. [Crossref] [PubMed]
- Adams JW, Paxton L, Dawes K, et al. 3D printed reproductions of orbital dissections: a novel mode of visualising anatomy for trainees in ophthalmology or optometry. Br J Ophthalmol 2015;99:1162-7. [Crossref] [PubMed]
- Baimakhanov Z, Soyama A, Takatsuki M, et al. Preoperative simulation with a 3-dimensional printed solid model for one-step reconstruction of multiple hepatic veins during living donor liver transplantation. Liver Transpl 2015;21:266-8. [Crossref] [PubMed]
- Mahmoud A, Bennett M. Introducing 3-Dimensional Printing of a Human Anatomic Pathology Specimen: Potential Benefits for Undergraduate and Postgraduate Education and Anatomic Pathology Practice. Arch Pathol Lab Med 2015;139:1048-51. [Crossref] [PubMed]
- Gillaspie EA, Matsumoto JS, Morris NE, et al. From 3-Dimensional Printing to 5-Dimensional Printing: Enhancing Thoracic Surgical Planning and Resection of Complex Tumors. Ann Thorac Surg 2016;101:1958-62. [Crossref] [PubMed]
- Byrne N, Velasco Forte M, Tandon A, et al. A systematic review of image segmentation methodology, used in the additive manufacture of patient-specific 3D printed models of the cardiovascular system. JRSM Cardiovasc Dis 2016;5:2048004016645467. [Crossref] [PubMed]
- Fuller SM, Butz DR, Vevang CB, et al. Application of 3-dimensional printing in hand surgery for production of a novel bone reduction clamp. J Hand Surg Am 2014;39:1840-5. [Crossref] [PubMed]
- Kurenov SN, Ionita C, Sammons D, et al. Three-dimensional printing to facilitate anatomic study, device development, simulation, and planning in thoracic surgery. J Thorac Cardiovasc Surg 2015;149:973-9.e1. [Crossref] [PubMed]
- Petzold R, Zeilhofer HF, Kalender WA. Rapid protyping technology in medicine--basics and applications. Comput Med Imaging Graph 1999;23:277-84. [Crossref] [PubMed]
- Yu AW, Khan M. On-demand three-dimensional printing of surgical supplies in conflict zones. J Trauma Acute Care Surg 2015;78:201-3. [Crossref] [PubMed]
- Okumoto T, Sakamoto Y, Kondo S, et al. Salt as a new colored solid model for simulation surgery. J Craniofac Surg 2015;26:680-1. [Crossref] [PubMed]
- Morrison RJ, Kashlan KN, Flanangan CL, et al. Regulatory Considerations in the Design and Manufacturing of Implantable 3D-Printed Medical Devices. Clin Transl Sci 2015;8:594-600. [Crossref] [PubMed]
- Morrison RJ, Hollister SJ, Niedner MF, et al. Mitigation of tracheobronchomalacia with 3D-printed personalized medical devices in pediatric patients. Sci Transl Med 2015;7:285ra64. [Crossref] [PubMed]
- Fiaschi P, Pavanello M, Imperato A, et al. Surgical results of cranioplasty with a polymethylmethacrylate customized cranial implant in pediatric patients: a single-center experience. J Neurosurg Pediatr 2016;17:705-10. [Crossref] [PubMed]
- Rankin TM, Giovinco NA, Cucher DJ, et al. Three-dimensional printing surgical instruments: are we there yet? J Surg Res 2014;189:193-7. [Crossref] [PubMed]
- Martelli N, Serrano C, van den Brink H, et al. Advantages and disadvantages of 3-dimensional printing in surgery: A systematic review. Surgery 2016;159:1485-500. [Crossref] [PubMed]
- Wang YT, Yang XJ, Yan B, et al. Clinical application of three-dimensional printing in the personalized treatment of complex spinal disorders. Chin J Traumatol 2016;19:31-4. [Crossref] [PubMed]
- Li C, Yang M, Xie Y, et al. Application of the polystyrene model made by 3-D printing rapid prototyping technology for operation planning in revision lumbar discectomy. J Orthop Sci 2015;20:475-80. [Crossref] [PubMed]
- Kaneyama S, Sugawara T, Sumi M. Safe and accurate midcervical pedicle screw insertion procedure with the patient-specific screw guide template system. Spine 2015;40:E341-8. [Crossref] [PubMed]
- Chen H, Wu D, Yang H, Guo K. Clinical Use of 3D Printing Guide Plate in Posterior Lumbar Pedicle Screw Fixation. Med Sci Monit 2015;21:3948-54. [Crossref] [PubMed]
- Zheng YX, Yu DF, Zhao JG, et al. 3D Printout Models vs. 3D-Rendered Images: Which Is Better for Preoperative Planning? J Surg Educ 2016;73:518-23. [Crossref] [PubMed]
- Pacione D, Tanweer O, Berman P, et al. The utility of a multimaterial 3D printed model for surgical planning of complex deformity of the skull base and craniovertebral junction. J Neurosurg 2016;125:1194-7. [Crossref] [PubMed]
- Yang M, Li C, Li Y, et al. Application of 3D rapid prototyping technology in posterior corrective surgery for Lenke 1 adolescent idiopathic scoliosis patients. Medicine 2015;94:e582. [Crossref] [PubMed]
- Salloum C, Lim C, Fuentes L, et al. Fusion of Information from 3D Printing and Surgical Robot: An Innovative Minimally Technique Illustrated by the Resection of a Large Celiac Trunk Aneurysm. World J Surg 2016;40:245-7. [Crossref] [PubMed]
- Leotta DF, Starnes BW. Custom fenestration templates for endovascular repair of juxtarenal aortic aneurysms. J Vasc Surg 2015;61:1637-41. [Crossref] [PubMed]
- Håkansson A, Rantatalo M, Hansen T, et al. Patient specific biomodel of the whole aorta - the importance of calcified plaque removal. Vasa 2011;40:453-9. [Crossref] [PubMed]
- Farooqi KM, Gonzalez-Lengua C, Shenoy R, et al. Use of a Three Dimensional Printed Cardiac Model to Assess Suitability for Biventricular Repair. World J Pediatr Congenit Heart Surg 2016;7:414-6. [Crossref] [PubMed]
- Valverde I, Gomez G, Gonzalez A, et al. Three-dimensional patient-specific cardiac model for surgical planning in Nikaidoh procedure. Cardiol Young 2015;25:698-704. [Crossref] [PubMed]
- Gosnell J, Pietila T, Samuel BP, et al. Integration of Computed Tomography and Three-Dimensional Echocardiography for Hybrid Three-Dimensional Printing in Congenital Heart Disease. J Digit Imaging 2016;29:665-9. [Crossref] [PubMed]
- Garekar S, Bharati A, Chokhandre M, et al. Clinical Application and Multidisciplinary Assessment of Three Dimensional Printing in Double Outlet Right Ventricle With Remote Ventricular Septal Defect. World J Pediatr Congenit Heart Surg 2016;7:344-50. [Crossref] [PubMed]
- Schmauss D, Gerber N, Sodian R. Three-dimensional printing of models for surgical planning in patients with primary cardiac tumors. J Thorac Cardiovasc Surg 2013;145:1407-8. [Crossref] [PubMed]
- Yang DH, Kang JW, Kim N, et al. Myocardial 3-Dimensional Printing for Septal Myectomy Guidance in a Patient With Obstructive Hypertrophic Cardiomyopathy. Circulation. 2015;132:300-1. [Crossref] [PubMed]
- Son KH, Kim KW, Ahn CB, et al. Surgical Planning by 3D Printing for Primary Cardiac Schwannoma Resection. Yonsei Med J 2015;56:1735-7. [Crossref] [PubMed]
- Sodian R, Weber S, Markert M, et al. Pediatric cardiac transplantation: three-dimensional printing of anatomic models for surgical planning of heart transplantation in patients with univentricular heart. J Thorac Cardiovasc Surg 2008;136:1098-9. [Crossref] [PubMed]
- Olivieri LJ, Krieger A, Loke YH, et al. A. Three-dimensional printing of intracardiac defects from three-dimensional echocardiographic images: feasibility and relative accuracy. J Am Soc Echocardiogr 2015;28:392-7. [Crossref] [PubMed]
- Akiba T, Nakada T, Inagaki T. A three-dimensional mediastinal model created with rapid prototyping in a patient with ectopic thymoma. Ann Thorac Cardiovasc Surg 2015;21:87-9. [Crossref] [PubMed]
- Schmauss D, Juchem G, Weber S, et al. Three-dimensional printing for perioperative planning of complex aortic arch surgery. Ann Thorac Surg 2014;97:2160-3. [Crossref] [PubMed]
- Nakada T, Akiba T, Inagaki T, et al. Thoracoscopic anatomical subsegmentectomy of the right S2b + S3 using a 3D printing model with rapid prototyping. Interact Cardiovasc Thorac Surg 2014;19:696-8. [Crossref] [PubMed]
- Akiba T, Inagaki T, Nakada T. Three-dimensional printing model of anomalous bronchi before surgery. Ann Thorac Cardiovasc Surg 2014;20 Suppl:659-62. [Crossref] [PubMed]
- Dickinson KJ, Matsumoto J, Cassivi SD, et al. Individualizing Management of Complex Esophageal Pathology Using Three-Dimensional Printed Models. Ann Thorac Surg 2015;100:692-7. [Crossref] [PubMed]
- Sodian R, Schmauss D, Markert M, et al. Three-dimensional printing creates models for surgical planning of aortic valve replacement after previous coronary bypass grafting. Ann Thorac Surg 2008;85:2105-8. [Crossref] [PubMed]
- Xiang N, Fang C, Fan Y, et al. Application of liver three-dimensional printing in hepatectomy for complex massive hepatocarcinoma with rare variations of portal vein: preliminary experience. Int J Clin Exp Med 2015;8:18873-8. [PubMed]
- Igami T, Nakamura Y, Hirose T, et al. Application of a three-dimensional print of a liver in hepatectomy for small tumors invisible by intraoperative ultrasonography: preliminary experience. World J Surg 2014;38:3163-6. [Crossref] [PubMed]
- Zein NN, Hanouneh IA, Bishop PD, et al. Three-dimensional print of a liver for preoperative planning in living donor liver transplantation. Liver Transpl 2013;19:1304-10. [Crossref] [PubMed]
- Tam MD, Laycock SD, Bell D, et al. 3-D printout of a DICOM file to aid surgical planning in a 6 year old patient with a large scapular osteochondroma complicating congenital diaphyseal aclasia. J Radiol Case Rep 2012;6:31-7. [Crossref] [PubMed]
- Wu XB, Wang JQ, Zhao CP, et al. Printed three-dimensional anatomic templates for virtual preoperative planning before reconstruction of old pelvic injuries: initial results. Chin Med J (Engl) 2015;128:477-82. [Crossref] [PubMed]
- Sheth U, Theodoropoulos J, Abouali J. Use of 3-Dimensional Printing for Preoperative Planning in the Treatment of Recurrent Anterior Shoulder Instability. Arthrosc Tech 2015;4:e311-6. [Crossref] [PubMed]
- Srougi V, Rocha BA, Tanno FY, et al. The Use of Three-dimensional Printers for Partial Adrenalectomy: Estimating the Resection Limits. Urology 2016;90:217-20. [Crossref] [PubMed]
- Zhang Y, Ge HW, Li NC, et al. Evaluation of three-dimensional printing for laparoscopic partial nephrectomy of renal tumors: a preliminary report. World J Urol 2016;34:533-7. [Crossref] [PubMed]
- Bernhard JC, Isotani S, Matsugasumi T, et al. Personalized 3D printed model of kidney and tumor anatomy: a useful tool for patient education. World J Urol 2016;34:337-45. [Crossref] [PubMed]
- Engel M, Hoffmann J, Castrillon-Oberndorfer G, et al. The value of three-dimensional printing modelling for surgical correction of orbital hypertelorism. Oral Maxillofac Surg 2015;19:91-5. [Crossref] [PubMed]
- Nkenke E, Eitner S. Complex hemimaxillary rehabilitation with a prefabricated fibula flap and cast-based vacuum-formed surgical template. J Prosthet Dent 2014;111:521-4. [Crossref] [PubMed]
- Chai G, Tan A, Yao CA, et al. Treating Parry-Romberg Syndrome Using Three-Dimensional Scanning and Printing and the Anterolateral Thigh Dermal Adipofascial Flap. J Craniofac Surg 2015;26:1826-9. [Crossref] [PubMed]
- Mendez BM, Chiodo MV, Patel PA. Customized "In-Office" Three-Dimensional Printing for Virtual Surgical Planning in Craniofacial Surgery. J Craniofac Surg 2015;26:1584-6. [Crossref] [PubMed]
- Chae MP, Hunter-Smith DJ, De-Silva I, et al. Four-Dimensional (4D) Printing: A New Evolution in Computed Tomography-Guided Stereolithographic Modeling. Principles and Application. J Reconstr Microsurg 2015;31:458-63. [Crossref] [PubMed]
- Chae MP, Lin F, Spychal RT, et al. 3D-printed haptic "reverse" models for preoperative planning in soft tissue reconstruction: a case report. Microsurgery 2015;35:148-53. [Crossref] [PubMed]
- Tan H, Yang K, Wei P, et al. A Novel Preoperative Planning Technique Using a Combination of CT Angiography and Three-Dimensional Printing for Complex Toe-to-Hand Reconstruction. J Reconstr Microsurg 2015;31:369-77. [Crossref] [PubMed]
- Burdall OC, Makin E, Davenport M, et al. 3D printing to simulate laparoscopic choledochal surgery. J Pediatr Surg 2016;51:828-31. [Crossref] [PubMed]
- Kiraly L, Tofeig M, Jha NK, et al. Three-dimensional printed prototypes refine the anatomy of post-modified Norwood-1 complex aortic arch obstruction and allow presurgical simulation of the repair. Interact Cardiovasc Thorac Surg 2016;22:238-40. [Crossref] [PubMed]
- Valverde I, Gomez G, Coserria JF, et al. 3D printed models for planning endovascular stenting in transverse aortic arch hypoplasia. Catheter Cardiovasc Interv 2015;85:1006-12. [Crossref] [PubMed]
- Barsness KA, Rooney DM, Davis LM, et al. Evaluation of Three Sources of Validity Evidence for a Synthetic Thoracoscopic Esophageal Atresia/Tracheoesophageal Fistula Repair Simulator. J Laparoendosc Adv Surg Tech A 2015;25:599-604. [Crossref] [PubMed]
- Akiba T, Nakada T, Inagaki T. Simulation of the fissureless technique for thoracoscopic segmentectomy using rapid prototyping. Ann Thorac Cardiovasc Surg 2015;21:84-6. [Crossref] [PubMed]
- Schmauss D, Schmitz C, Bigdeli AK, et al. Three-dimensional printing of models for preoperative planning and simulation of transcatheter valve replacement. Ann Thorac Surg 2012;93:e31-3. [Crossref] [PubMed]
- Miyazaki T, Yamasaki N, Tsuchiya T, et al. Airway stent insertion simulated with a three-dimensional printed airway model. Ann Thorac Surg 2015;99:e21-3. [Crossref] [PubMed]
- Barsness KA, Rooney DM, Davis LM, et al. Preliminary evaluation of a novel thoracoscopic infant lobectomy simulator. J Laparoendosc Adv Surg Tech A 2015;25:429-34. [Crossref] [PubMed]
- Takagi K, Nanashima A, Abo T, et al. Three-dimensional printing model of liver for operative simulation in perihilar cholangiocarcinoma. Hepatogastroenterology 2014;61:2315-6. [PubMed]
- Souzaki R, Kinoshita Y, Ieiri S, et al. Three-dimensional liver model based on preoperative CT images as a tool to assist in surgical planning for hepatoblastoma in a child. Pediatr Surg Int 2015;31:593-6. [Crossref] [PubMed]
- Chen F, Miyamoto E, Takemoto M, et al. Right and left inverted lobar lung transplantation. Am J Transplant 2015;15:1716-21. [Crossref] [PubMed]
- Kusaka M, Sugimoto M, Fukami N, et al. Initial experience with a tailor-made simulation and navigation program using a 3-D printer model of kidney transplantation surgery. Transplant Proc 2015;47:596-9. [Crossref] [PubMed]
- Park JH, Lee Y, Shon OJ, et al. Surgical tips of intramedullary nailing in severely bowed femurs in atypical femur fractures: Simulation with 3D printed model. Injury 2016;47:1318-24. [Crossref] [PubMed]
- Rose AS, Webster CE, Harrysson OL, et al. Pre-operative simulation of pediatric mastoid surgery with 3D-printed temporal bone models. Int J Pediatr Otorhinolaryngol 2015;79:740-4. [Crossref] [PubMed]
- Rose AS, Kimbell JS, Webster CE, et al. Multi-material 3D Models for Temporal Bone Surgical Simulation. Ann Otol Rhinol Laryngol 2015;124:528-36. [Crossref] [PubMed]
- Johnson CM, Howell JT, Mettenburg DJ, et al. Mechanical Modeling of the Human Cricoid Cartilage Using Computer-Aided Design: Applications in Airway Balloon Dilation Research. Ann Otol Rhinol Laryngol 2016;125:69-76. [Crossref] [PubMed]
- Berens AM, Newman S, Bhrany AD, et al. Computer-Aided Design and 3D Printing to Produce a Costal Cartilage Model for Simulation of Auricular Reconstruction. Otolaryngol Head Neck Surg 2016;155:356-9. [Crossref] [PubMed]
- Namba K, Higaki A, Kaneko N, et al. Microcatheter Shaping for Intracranial Aneurysm Coiling Using the 3-Dimensional Printing Rapid Prototyping Technology: Preliminary Result in the First 10 Consecutive Cases. World Neurosurg 2015;84:178-86. [Crossref] [PubMed]
- Tam MD, Laycock SD, Brown JR, et al. 3D printing of an aortic aneurysm to facilitate decision making and device selection for endovascular aneurysm repair in complex neck anatomy. J Endovasc Ther 2013;20:863-7. [Crossref] [PubMed]
- Chung KJ. Preshaping plates for minimally invasive fixation of calcaneal fractures using a real-size 3D-printed model as a preoperative and intraoperative tool. Foot Ankle Int 2014;35:1231-6. [Crossref] [PubMed]
- Jeong HS, Park KJ, Kil KM, et al. Minimally invasive plate osteosynthesis using 3D printing for shaft fractures of clavicles: technical note. Arch Orthop Trauma Surg 2014;134:1551-5. [Crossref] [PubMed]
- Kim HN, Liu XN, Noh KC. Use of a real-size 3D-printed model as a preoperative and intraoperative tool for minimally invasive plating of comminuted midshaft clavicle fractures. J Orthop Surg Res 2015;10:91. [Crossref] [PubMed]
- Fernandes N, van den Heever J, Hoogendijk C, et al. Reconstruction of an Extensive Midfacial Defect Using Additive Manufacturing Techniques. J Prosthodont 2016;25:589-94. [Crossref] [PubMed]
- Jardini AL, Larosa MA, Maciel Filho R, et al. Cranial reconstruction: 3D biomodel and custom-built implant created using additive manufacturing. J Craniomaxillofac Surg 2014;42:1877-84. [Crossref] [PubMed]
- Azuma M, Yanagawa T, Ishibashi-Kanno N, et al. Mandibular reconstruction using plates prebent to fit rapid prototyping 3-dimensional printing models ameliorates contour deformity. Head Face Med 2014;10:45. [Crossref] [PubMed]
- Park SW, Choi JW, Koh KS, et al. Mirror-Imaged Rapid Prototype Skull Model and Pre-Molded Synthetic Scaffold to Achieve Optimal Orbital Cavity Reconstruction. J Oral Maxillofac Surg 2015;73:1540-53. [Crossref] [PubMed]
- Liu YF, Xu LW, Zhu HY, et al. Technical procedures for template-guided surgery for mandibular reconstruction based on digital design and manufacturing. Biomed Eng Online 2014;13:63. [Crossref] [PubMed]
- Cohen A, Laviv A, Berman P, et al. Mandibular reconstruction using stereolithographic 3-dimensional printing modeling technology. Oral Surg Oral Med Oral Pathol Oral Radiol Endod 2009;108:661-6. [Crossref] [PubMed]
- Thomas CV, McMillan KG, Jeynes P, et al. Use of a titanium cutting guide to assist with raising and inset of a DCIA free flap. Br J Oral Maxillofac Surg 2013;51:958-61. [Crossref] [PubMed]
- De Santis R, Gloria A, Russo T, et al. Reverse engineering of mandible and prosthetic framework: Effect of titanium implants in conjunction with titanium milled full arch bridge prostheses on the biomechanics of the mandible. J Biomech 2014;47:3825-9. [Crossref] [PubMed]
- Lim CG, Campbell DI, Clucas DM. Rapid prototyping technology in orbital floor reconstruction: application in three patients. Craniomaxillofac Trauma Reconstr 2014;7:143-6. [Crossref] [PubMed]
- Man QW, Jia J, Liu K, et al. Secondary reconstruction for mandibular osteoradionecrosis defect with fibula osteomyocutaneous flap flowthrough from radial forearm flap using stereolithographic 3-dimensional printing modeling technology. J Craniofac Surg 2015;26:e190-3. [Crossref] [PubMed]
- Hatamleh MM, Cartmill M, Watson J. Management of extensive frontal cranioplasty defects. J Craniofac Surg 2013;24:2018-22. [Crossref] [PubMed]
- Kang SJ, Oh MJ, Jeon SP. A Novel and Easy Approach for Contouring Surgery in Patients With Craniofacial Fibrous Dysplasia. J Craniofac Surg 2015;26:1977-8. [Crossref] [PubMed]
- Daniel M, Watson J, Hoskison E, et al. Frontal sinus models and onlay templates in osteoplastic flap surgery. J Laryngol Otol 2011;125:82-5. [Crossref] [PubMed]
- Shen C, Yao CA, Magee W 3rd, et al. Presurgical nasoalveolar molding for cleft lip and palate: the application of digitally designed molds. Plast Reconstr Surg 2015;135:1007e-15e. [Crossref] [PubMed]
- Costello JP, Olivieri LJ, Krieger A, et al. Utilizing Three-Dimensional Printing Technology to Assess the Feasibility of High-Fidelity Synthetic Ventricular Septal Defect Models for Simulation in Medical Education. World J Pediatr Congenit Heart Surg 2014;5:421-6. [Crossref] [PubMed]
- Wanibuchi M, Noshiro S, Sugino T, et al. Training for Skull Base Surgery with a Colored Temporal Bone Model Created by Three-Dimensional Printing Technology. World Neurosurg 2016;91:66-72. [Crossref] [PubMed]
- Ryan JR, Almefty KK, Nakaji P, et al. Cerebral Aneurysm Clipping Surgery Simulation Using Patient-Specific 3D Printing and Silicone Casting. World Neurosurg 2016;88:175-81. [Crossref] [PubMed]
- Lan Q, Chen A, Zhang T, et al. Development of Three-Dimensional Printed Craniocerebral Models for Simulated Neurosurgery. World Neurosurg 2016;91:434-42. [Crossref] [PubMed]
- Mashiko T, Otani K, Kawano R, et al. Development of three-dimensional hollow elastic model for cerebral aneurysm clipping simulation enabling rapid and low cost prototyping. World Neurosurg 2015;83:351-61. [Crossref] [PubMed]
- Ploch CC, Mansi CS, Jayamohan J, et al. Using 3D Printing to Create Personalized Brain Models for Neurosurgical Training and Preoperative Planning. World Neurosurg 2016;90:668-74. [Crossref] [PubMed]
- Waran V, Narayanan V, Karuppiah R, et al. Neurosurgical endoscopic training via a realistic 3-dimensional model with pathology. Simul Healthc 2015;10:43-8. [PubMed]
- Menikou G, Dadakova T, Pavlina M, et al. MRI compatible head phantom for ultrasound surgery. Ultrasonics 2015;57:144-52. [Crossref] [PubMed]
- Ryan JR, Chen T, Nakaji P, et al. Ventriculostomy Simulation Using Patient-Specific Ventricular Anatomy, 3D Printing, and Hydrogel Casting. World Neurosurg 2015;84:1333-9. [Crossref] [PubMed]
- Mashiko T, Konno T, Kaneko N, et al. Training in Brain Retraction Using a Self-Made Three-Dimensional Model. World Neurosurg 2015;84:585-90. [Crossref] [PubMed]
- Cheung CL, Looi T, Lendvay TS, et al. Use of 3-dimensional printing technology and silicone modeling in surgical simulation: development and face validation in pediatric laparoscopic pyeloplasty. J Surg Educ 2014;71:762-7. [Crossref] [PubMed]
- Holt BA, Hearn G, Hawes R, et al. Development and evaluation of a 3D printed endoscopic ampullectomy training model (with video). Gastrointest Endosc 2015;81:1470-1475.e5. [Crossref] [PubMed]
- Mowry SE, Jammal H, Myer C 4th, et al. A Novel Temporal Bone Simulation Model Using 3D Printing Techniques. Otol Neurotol 2015;36:1562-5. [Crossref] [PubMed]
- Da Cruz MJ, Francis HW. Face and content validation of a novel three-dimensional printed temporal bone for surgical skills development. J Laryngol Otol 2015;129 Suppl 3:S23-9. [Crossref] [PubMed]
- Longfield EA, Brickman TM, Jeyakumar A. 3D Printed Pediatric Temporal Bone: A Novel Training Model. Otol Neurotol 2015;36:793-5. [Crossref] [PubMed]
- Waran V, Menon R, Pancharatnam D, et al. The creation and verification of cranial models using three-dimensional rapid prototyping technology in field of transnasal sphenoid endoscopy. Am J Rhinol Allergy 2012;26:e132-6. [Crossref] [PubMed]
- Narayanan V, Narayanan P, Rajagopalan R, et al. Endoscopic skull base training using 3D printed models with pre-existing pathology. Eur Arch Otorhinolaryngol 2015;272:753-7. [Crossref] [PubMed]
- Grunert R, Strauss G, Moeckel H, et al. ElePhant--an anatomical electronic phantom as simulation-system for otologic surgery. Conf Proc IEEE Eng Med Biol Soc 2006;1:4408-11. [Crossref] [PubMed]
- Zheng Y, Lu B, Zhang J, et al. CAD/CAM silicone simulator for teaching cheiloplasty: description of the technique. Br J Oral Maxillofac Surg 2015;53:194-6. [Crossref] [PubMed]
- Scawn RL, Foster A, Lee BW, et al. Customised 3D Printing: An Innovative Training Tool for the Next Generation of Orbital Surgeons. Orbit 2015;34:216-9. [Crossref] [PubMed]
- Qiao F, Li D, Jin Z, et al. A novel combination of computer-assisted reduction technique and three dimensional printed patient-specific external fixator for treatment of tibial fractures. Int Orthop 2016;40:835-41. [Crossref] [PubMed]
- Qiao F, Li D, Jin Z, et al. Application of 3D printed customized external fixator in fracture reduction. Injury. 2015;46:1150-5. [Crossref] [PubMed]
- Xu N, Wei F, Liu X, et al. Reconstruction of the Upper Cervical Spine Using a Personalized 3D-Printed Vertebral Body in an Adolescent With Ewing Sarcoma. Spine 2016;41:E50-4. [Crossref] [PubMed]
- Wang L, Cao T, Li X, et al. Three-dimensional printing titanium ribs for complex reconstruction after extensive posterolateral chest wall resection in lung cancer. J Thorac Cardiovasc Surg 2016;152:e5-7. [Crossref] [PubMed]
- Wong KC, Kumta SM, Geel NV, et al. One-step reconstruction with a 3D-printed, biomechanically evaluated custom implant after complex pelvic tumor resection. Comput Aided Surg 2015;20:14-23. [Crossref] [PubMed]
- Li H, Qu X, Mao Y, et al. Custom Acetabular Cages Offer Stable Fixation and Improved Hip Scores for Revision THA With Severe Bone Defects. Clin Orthop Relat Res 2016;474:731-40. [Crossref] [PubMed]
- Shuang F, Hu W, Shao Y, et al. Treatment of Intercondylar Humeral Fractures With 3D-Printed Osteosynthesis Plates. Medicine 2016;95:e2461. [Crossref] [PubMed]
- Park CJ, Kim HW, Jeong S, et al. Anti-Reflux Ureteral Stent with Polymeric Flap Valve Using Three-Dimensional Printing: An In Vitro Study. J Endourol 2015;29:933-8. [Crossref] [PubMed]
- Shan XF, Chen HM, Liang J, et al. Surgical Reconstruction of Maxillary and Mandibular Defects Using a Printed Titanium Mesh. J Oral Maxillofac Surg 2015;73:1437.e1-9. [Crossref] [PubMed]
- He W, Sun Y, Tian K, et al. Novel Arch Bar Fabricated With a Computer-Aided Design and Three-Dimensional Printing: A Feasibility Study. J Oral Maxillofac Surg 2015;73:2162-8. [Crossref] [PubMed]
- Green JM 3rd, Lawson ST, Liacouras PC, et al. Custom Anatomical 3D Spacer for Temporomandibular Joint Resection and Reconstruction. Craniomaxillofac Trauma Reconstr 2016;9:82-7. [Crossref] [PubMed]
- Bos EJ, Scholten T, Song Y, et al. Developing a parametric ear model for auricular reconstruction: a new step towards patient-specific implants. J Craniomaxillofac Surg 2015;43:390-5. [Crossref] [PubMed]
- Nuseir A, Hatamleh M, Watson J, et al. Improved Construction of Auricular Prosthesis by Digital Technologies. J Craniofac Surg 2015;26:e502-5. [Crossref] [PubMed]
- Zopf DA, Mitsak AG, Flanagan CL, et al. Computer aided-designed, 3-dimensionally printed porous tissue bioscaffolds for craniofacial soft tissue reconstruction. Otolaryngol Head Neck Surg 2015;152:57-62. [Crossref] [PubMed]
- Ruiters S, Sun Y, de Jong S, et al. Computer-aided design and three-dimensional printing in the manufacturing of an ocular prosthesis. Br J Ophthalmol 2016. [Epub ahead of print]. [Crossref] [PubMed]
- Debellemanière G, Flores M, Montard M, et al. Three-dimensional Printing of Optical Lenses and Ophthalmic Surgery: Challenges and Perspectives. J Refract Surg 2016;32:201-4. [Crossref] [PubMed]
- Soleman J, Thieringer F, Beinemann J, et al. Computer-assisted virtual planning and surgical template fabrication for frontoorbital advancement. Neurosurg Focus 2015;38:E5. [Crossref] [PubMed]
- Maeda M, Kanai N, Kobayashi S, et al. Endoscopic cell sheet transplantation device developed by using a 3-dimensional printer and its feasibility evaluation in a porcine model. Gastrointest Endosc 2015;82:147-52. [Crossref] [PubMed]
- Ma L, Zhou Y, Zhu Y, et al. 3D-printed guiding templates for improved osteosarcoma resection. Sci Rep 2016;6:23335. [Crossref] [PubMed]
- Hirao M, Ikemoto S, Tsuboi H, et al. Computer assisted planning and custom-made surgical guide for malunited pronation deformity after first metatarsophalangeal joint arthrodesis in rheumatoid arthritis: a case report. Comput Aided Surg 2014;19:13-9. [Crossref] [PubMed]
- Jaffry Z, Masjedi M, Clarke S, et al. Unicompartmental knee arthroplasties: robot vs. patient specific instrumentation. Knee 2014;21:428-34. [Crossref] [PubMed]
- Huang H, Hsieh MF, Zhang G, et al. Improved accuracy of 3D-printed navigational template during complicated tibial plateau fracture surgery. Australas Phys Eng Sci Med 2015;38:109-17. [Crossref] [PubMed]
- del Junco M, Okhunov Z, Yoon R, et al. Development and initial porcine and cadaver experience with three-dimensional printing of endoscopic and laparoscopic equipment. J Endourol 2015;29:58-62. [Crossref] [PubMed]
- Priester A, Natarajan S, Le JD, et al. A system for evaluating magnetic resonance imaging of prostate cancer using patient-specific 3D printed molds. Am J Clin Exp Urol 2014;2:127-35. [PubMed]
- Lee UL, Kwon JS, Choi YJ. Keyhole System: A Computer-Assisted Designed and Computer-Assisted Manufactured Maxillomandibular Complex Repositioner in Orthognathic Surgery. J Oral Maxillofac Surg 2015;73:2024-9. [Crossref] [PubMed]
- Shqaidef A, Ayoub AF, Khambay BS. How accurate are rapid prototyped (RP) final orthognathic surgical wafers? A pilot study. Br J Oral Maxillofac Surg 2014;52:609-14. [Crossref] [PubMed]
- Kang SH, Kim MK, Kim BC, et al. Orthognathic Y-splint: a CAD/CAM-engineered maxillary repositioning wafer assembly. Br J Oral Maxillofac Surg 2014;52:667-9. [Crossref] [PubMed]
- Adolphs N, Liu W, Keeve E, et al. RapidSplint: virtual splint generation for orthognathic surgery - results of a pilot series. Comput Aided Surg 2014;19:20-8. [Crossref] [PubMed]
- Li B, Zhang L, Sun H, et al. A novel method of computer aided orthognathic surgery using individual CAD/CAM templates: a combination of osteotomy and repositioning guides. Br J Oral Maxillofac Surg 2013;51:e239-44. [Crossref] [PubMed]
- Li Y, Jiang Y, Ye B, et al. Treatment of Dentofacial Deformities Secondary to Osteochondroma of the Mandibular Condyle Using Virtual Surgical Planning and 3-Dimensional Printed Surgical Templates. J Oral Maxillofac Surg 2016;74:349-68. [Crossref] [PubMed]
- Reiser V. V-stand--a versatile surgical platform for oromandibular reconstruction using a 3-dimensional virtual modeling system. J Oral Maxillofac Surg 2015;73:1211-26. [Crossref] [PubMed]
- Li B, Shen SG, Yu H, et al. A new design of CAD/CAM surgical template system for two-piece narrowing genioplasty. Int J Oral Maxillofac Surg 2016;45:560-6. [Crossref] [PubMed]
- Mazzoni S, Bianchi A, Schiariti G, et al. Computer-aided design and computer-aided manufacturing cutting guides and customized titanium plates are useful in upper maxilla waferless repositioning. J Oral Maxillofac Surg 2015;73:701-7. [Crossref] [PubMed]
- Kang SH, Kim MK, You TK, et al. Modification of planned postoperative occlusion in orthognathic surgery, based on computer-aided design/computer-aided manufacturing-engineered preoperative surgical simulation. J Oral Maxillofac Surg 2015;73:134-51. [Crossref] [PubMed]
- el-Gengehi M, Seif SA. Evaluation of the Accuracy of Computer-Guided Mandibular Fracture Reduction. J Craniofac Surg 2015;26:1587-91. [Crossref] [PubMed]
- Dong Z, Li Q, Bai S, et al. Application of 3-Dimensional Printing Technology to Kirschner Wire Fixation of Adolescent Condyle Fracture. J Oral Maxillofac Surg 2015;73:1970-6. [Crossref] [PubMed]
- Flügge TV, Nelson K, Schmelzeisen R, et al. Three-dimensional plotting and printing of an implant drilling guide: simplifying guided implant surgery. J Oral Maxillofac Surg 2013;71:1340-6. [Crossref] [PubMed]
- Vehmeijer M, van Eijnatten M, Liberton N, et al. A Novel Method of Orbital Floor Reconstruction Using Virtual Planning, 3-Dimensional Printing, and Autologous Bone. J Oral Maxillofac Surg 2016;74:1608-12. [Crossref] [PubMed]
- Shi L, Liu W, Yin L, et al. Surgical guide assistant mandibular distraction osteogenesis and sagittal split osteotomy in the treatment of hemifacial microsomia. J Craniofac Surg 2015;26:498-500. [Crossref] [PubMed]
- Yin L, Tang X, Shi L, et al. Mandibular distraction combined with orthognathic techniques for the correction of severe adult mandibular hypoplasia. J Craniofac Surg 2014;25:1947-52. [Crossref] [PubMed]
- Darwood A, Collier J, Joshi N, et al. Re-thinking 3D printing: A novel approach to guided facial contouring. J Craniomaxillofac Surg 2015;43:1256-60. [Crossref] [PubMed]
- Ye N, Long H, Zhu S, et al. The Accuracy of Computer Image-Guided Template for Mandibular Angle Ostectomy. Aesthetic Plast Surg 2015;39:117-23. [Crossref] [PubMed]
- Ruzza A, Parekh M, Ferrari S, et al. Preloaded donor corneal lenticules in a new validated 3D printed smart storage glide for Descemet stripping automated endothelial keratoplasty. Br J Ophthalmol 2015;99:1388-95. [Crossref] [PubMed]
- Stitely ML, Paterson H. Using Three-Dimensional Printing to Fabricate a Tubing Connector for Dilation and Evacuation. Obstet Gynecol 2016;127:317-9. [Crossref] [PubMed]