C9orf72 role in myeloid cells: new perspectives in the investigation of the neuro-immune crosstalk in amyotrophic lateral sclerosis and frontotemporal dementia
Although the central nervous system (CNS) has been regarded as an immune privilege organ for many years, we now know that the CNS is immunologically active and that the CNS and the immune system modulate and regulate each other in health, aging, and disease (1). The primary role is played by microglia, the CNS resident immune cells, which, besides their surveillance role, support synaptic plasticity and maturation by secreting trophic factors (1). Microglia are also at the base of the finely orchestrated multi-cellular response to CNS inflammation, which features the participation of cells within the CNS and the recruitment of cells from the periphery (2). Many neurodegenerative diseases (NDs) are characterized by neuroinflammation, in which cells such as astrocytes and microglia secrete neurotoxic cytokines contributing to chronic inflammation, exacerbating the severe tissue damage characteristic of all NDs (3). Lately, more and more studies are reporting the recruitment of immune cells from the periphery during the development of different NDs, strengthening the connection between these two processes (2).
Amyotrophic lateral sclerosis (ALS) and frontotemporal dementia (FTD) are two clinically distinct neurodegenerative disorders that are often co-diagnosed (4). ALS primarily affects motor neurons in the spinal cord and motor cortex and is characterized by the gradual weakening and loss of voluntary muscle movements. Patients typically present symptoms such as muscle weakness, spasticity, and respiratory difficulties (4). FTD impacts the frontal and temporal lobes of the brain, leading to profound changes in behavior, personality, and language. The mutation in the C9ORF72 gene, which accounts for many sporadic and inherited cases of both diseases, bridges ALS and FTD within a common genetic framework. The mutation is a GGGGCC hexanucleotide repeat expansion in the intron 1a of the C9ORF72 gene. In patients with ALS and FTD, this sequence aberrantly repeats hundreds to thousands of times, far exceeding normal variation (5,6). Identifying this mutation has deepened our understanding of ALS and FTD and opened new avenues for developing diagnostic tests and targeted therapies (5,6). The C9ORF72 gene product plays a crucial role in the health and function of various cell types. The expansion leads to reduced levels of the C9ORF72 protein, the formation of toxic RNA foci, and the aberrant translation of the repeat, known as repeat associated non-AUG translation (RAN-T). These three mechanisms gradually disrupt neuronal function, leading to neurodegeneration, astrocyte activation, and hyperreactive microglia (7).
Many critical studies have begun to assess the molecular role of C9ORF72, tying it to autophagy and endosomal pathways (8). At the cellular level, the role of C9ORF72 has been linked mainly to specific mechanisms of immunity and proper macrophage function. Mice knock-out for C9orf72 enables the characterization of one of the three toxic mechanisms (C9ORF72 haploinsufficiency) disjointed by the other two gain-of-function toxic mechanisms. Ablation of the C9orf72 is characterized by splenomegaly, an early indicator of inflammatory disease progression, and bone marrow-derived macrophages from C9orf72-/- mice show dysregulated responses to stimuli (9). Furthermore, C9orf72 ablation disrupts autophagy and reduces the timely degradation of the stimulator of interferon response cGAMP interactor 1 (STING) after stimulation, revealing a role for C9ORF72 in innate immunity and STING homeostasis (10). The study of C9ORF72 has thus brought attention to the importance of the immune system in ALS/FTD. Still, more needs to be done to address specifically its role in the relationship between immunity and CNS function during disease development. One hint comes from a study reporting that the impact of dysregulated immunity on the overall survival of the C9orf72-/- mice depends on the housing conditions of the mice. C9orf72-/- mice originating from different vivaria had varying compositions of microbiomes in their gut, significantly impacting their survival and microglial fate in the CNS. These findings have important implications for our comprehension of the systemic aspect of ALS/FTD and suggest a shift in our understanding of the pathology of these diseases.
In this context, the findings of Limone and colleagues significantly advance our understanding of C9ORF72 biological function in the CNS (11). Their research aimed to answer two crucial questions: first, to what extent are the immune phenotypes observed in C9ORF72-deficient mice due to the role of the protein in hematopoietic cells, and second, how does C9ORF72 promote organismal health?
To address these questions, the authors knocked out the murine C9orf72 gene in seven different immune cell lineages by conditional mutagenesis (Figure 1). Their study characterized the role of C9orf72 in immune lineage determination by deleting it at various myeloid and lymphoid differentiation stages. Interestingly, the deletion of C9orf72 from all hematopoietic stem cells through Vav1-dependent Cre recombinase led to spleen enlargement, a characteristic seen also in the C9orf72 global knock-out mice. Moreover, these mice exhibited a decreased lifespan. However, C9orf72 within the myeloid lineage, particularly in macrophages and monocytes, reduced the onset of systemic autoimmune inflammation in mice and promoted a longer life span. This discovery raises a crucial question about which other cells expressing C9ORF72 might also have a protective effect, in addition to myeloid cells. The authors also showed increased plasma autoantibodies, decreased platelet count, and increased neutrophil count when C9orf72 was deleted in all hematopoietic cells. The effect is diluted when C9orf72 is deleted from macrophages alone (LysM-Cre) and notably is absent when C9orf72 ablation is restricted to B (CD19-Cre) and T (CD2-Cre) cells. These results confirm that C9ORF72 has the most significant impact on myeloid function. Mice that lacked C9orf72 in all their hematopoietic cells significantly increased several cytokines in their plasma, including interleukin 17A (IL-17A). IL-17A, also known as IL-17, is a cytokine mainly associated with a subpopulation of helper T cells (TH17) (12). When this cytokine binds to its receptor, it triggers a cascade mediated by TRAF6 ubiquitination, which leads to NF-kB phosphorylation and translocation to the nucleus. Physiologically, IL-17 is involved in tissue repair and defense against pathogens. However, constant expression and release of this cytokine are associated with pathological events such as autoimmunity and cancer progression.
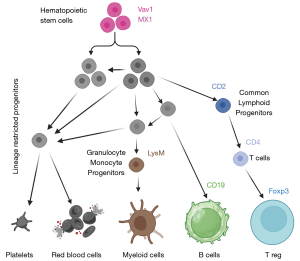
Limone and colleagues used mass cytometry, also known as cytometry by time of flight (CyTOF), to carry out complete immunophenotypic profiling of mice that lack C9orf72. They compared the results from the spleen of mice lacking C9orf72 with the control group, which had no C9orf72 ablation. CyTOF combines fluorescence-free cytometry and mass spectrometry, enabling the identification of up to 60 markers at a single-cell level resolution. This experiment provided vast data and insights that could lead to many discoveries. The authors focused their report on the increased abundance of CD80 on the surface of macrophages, monocytes, CD8 T cells, CD4 T cells, and B cells of 8-month-old C9orf72 knock-out mice. CD80 is a costimulatory molecule that usually works in concert with CD86 (13). After MHCII, present on antigen-presenting cells (APCs), is recognized by CD4 T cells, CD80 and CD86 are expressed on APCs like the dendritic cells (DCs) upon inflammatory stimuli. This mechanism grants faster T-cell activation. The continuous exposure of CD80 by different immune cells, but especially by DCs in C9orf72-/- mice, can thus lead to T cell hyperactivation. Intriguingly, CD86 expression was higher in C9orf72-/- mice at two months. Still, no significant differences were found at eight months of age, pointing to an age-dependent function of C9ORF72 in regulating these molecules. To answer the question about the molecular pathway by which C9ORF72 regulates CD80 exposure, the authors hypothesized the involvement of the endosomal pathway. Employing in vitro experiments on primary macrophage cell lines, they observed that CD80 exposure was explicitly dependent on Toll-like receptor 2 (TLR2) and 7/8 activation (but not on TLR3 and 4) in conditional, wild-type, and C9orf72 ablated mice. This effect was rescued by inhibiting the endosomal pathway, proving the relevance of this pathway to CD80 exposure. They then found that IL-17A alone cannot cause CD80 exposure but that the costimulation with INFγ led to high levels of CD80 on C9orf72-/- macrophages. This increase is significant but only partially reverted by the exogenous reintroduction of C9orf72 transcripts. These complex data indicate that the C9ORF72 role is crucial during stimulation. Indeed, without stimulation, the absence of C9ORF72 does not lead to CD80 exposure.
The authors then investigated the systemic relevance of IL-17A signaling in C9orf72-/- mice, implementing two different approaches to reduce IL-17A signaling. First, they showed that knock-out of IL-17A in C9orf72-/- mice reduces CD80 exposure on myeloid cells in the mesenteric lymph node, microglia, and monocytes in the spinal cord. They failed to observe similar results in the spleen, pointing to the notion that in splenic cells, CD80 exposure is not strongly dependent on the IL-17A signaling pathway. Secondly, they used an antibody against IL-17A in C9orf72-/- mice and showed a marked reduction to basal level in neutrophil count in blood, paralleled by a mild increase in motor functions. In addition, by single-cell RNA transcripts profiling the brain and spinal cord at 2, 4, and 8 months of age of C9orf72 depleted mice, the authors showed age-related increased expression of Apoe transcripts in microglia compared to their age-matched controls. Apoe encodes a protein that helps transport cholesterol and other types of fat in the blood, whose dysregulation may contribute to Alzheimer’s disease (AD) development (14). ApoE has also been linked to dysfunction in microglial function in AD (14).
Interestingly, IL-17A antibody treatment reduces the levels of Apoe transcript in C9orf72-/- microglia to resting levels. Microglial cells are the resident macrophages of the CNS and participate in brain health and diseases. A general feature of microglia in CNS diseases is an irreversible hyperactive state, usually defined as the M1 state. This state is characterized by the hyperproduction of cytokines, a different metabolic program, and increased phagocytic activity. In CNS diseases, M1 microglia cannot revert to M2 microglia (anti-inflammatory state). Owing to single-cell profiling techniques, we now know that the picture of microglia in brain health and disease is not binary. Indeed, it features many different microglia populations with potentially different roles and functions (1). The authors report that microglia in the brain and spinal cord of C9orf72-/- mice display increased CD80 exposure compared to heterozygous and non-transgenic controls. In agreement with these findings and in support of them, the authors also describe increased CD80 on the surface of microglial cells in three C9-ALS compared to three control cases.
The study presents an insightful analysis of the role of C9ORF72 in regulating cytokine signals in macrophages, which can pave the way for future studies specifically addressing macrophages in the CNS. Macrophage function is tightly related to proper signaling reception and transduction, while a growing body of evidence points to the notion that the absence of C9ORF72 affects these pathways by impairing autophagy. In this report, the focus is on the trafficking of CD80 following IL-17A signaling. This trafficking is impaired due to dysfunctional endosomal pathways. Interestingly, these results are paralleled by the findings related to the degradation of the STING protein. Overall, it appears critical for the correct function of macrophages to have a properly functional degradative system. A further contribution this report brings is the relevance of the molecule studied. CD80 is an important co-stimulatory molecule on APCs that initiates T cell response. The role of the immune system in the development and progression of ALS/FTD is not yet fully understood and requires further investigation. However, the malfunctioning of immune cells may contribute to the progression of the disease. Several studies have explored this possibility, showing promising results. In 2016, a report showed a decreased potency of Treg cells, which were ineffective in reverting the hyperactive status of microglia (15). Another possibility raised by Limone et al. is that microglia might also have a role in priming T cell response due to the aberrant exposure of CD80 in the condition of C9orf72 haploinsufficiency. An intriguing study recently published showed that in AD, microglia were responsible for priming T cell invasion of the CNS and that ablation of microglia could restore the cognitive functions of the mice (2). It remains to be elucidated whether this is also the case in ALS/FTD, precisely due to the increased exposure of CD80 on the surface of microglia. The hypothesis is very intriguing and can lead to many related studies. Another critical finding that remains to be further elucidated is the source of IL-17A increase in C9orf72 knock-out condition. From which cells is it produced, and why only in the absence of C9ORF72? Is C9ORF72 impacting cytokines production due to the skewed endosomal pathway? Another relevant aspect of these findings is the relationship between immunity and CNS functions. How are they related? Which cells bring about the damage, and by which mechanisms? Finally, can these results be applied to patients in a clinical setting?
This study suggests that IL-17A neutralizing antibodies can be used to decrease the signal of IL-17A, reducing the exposure of CD80 on macrophages. Studies have shown an elevated exposure of CD80/CD86 in microglia during inflammation (16). Combined with other research highlighting increased IL-17A levels in the patient’s serum and CSF (17), this validates the potential of therapeutically targeting this pathway. In order to establish an effective therapeutic approach, it is essential to address several critical issues. These include the method of best delivering the therapeutic antibodies to the CNS, the potential impact of such treatment, and the relevance of this pathway to the entirety of the disease in patients. It is essential to address these unanswered questions to ensure that any potential treatment is safe and effective for those with the disease.
Overall, the report authored by Limone and colleagues underscores the critical role of studying the immune system in NDs. The involvement of the immune system in ALS has already been assessed in many papers (18-21), but the specific role and mechanisms remain to be described. In a very recent work, regulatory T cells were found to be dysregulated in sporadic ALS cases (18). It was thus postulated that a low functionality of these cells was contributing to the sustained neuroinflammation present in ALS (18,19,22). An ongoing clinical trial has developed from these studies (NCT04055623) (20). The links between ALS progression and immune system reactivity are numerous, making this theory very robust. This paper adds and proposes a target that can be harnessed for the design of new therapies.
The novelty of this paper is the description of a new possible mechanism that involves CD80 molecules exposed by microglia. Microglial cells have been shown to work as APCs in neurodegenerative processes (1). They express major histocompatibility complex II (MHC-II) and recruit CD4 T cells. This paper offers further validation, solidifying the notion that microglia are at the center of the neuroinflammatory processes not only by becoming reactive, dysfunctional, and toxic but also by affecting the cell fate of other cell types in the CNS like astrocytes or priming peripheral mediators of inflammation like T cells.
The role of the immune cells is pivotal for understanding disease progression and devising potential treatments for other NDs, such as AD, Huntington disease (HD), Parkinson disease (PD), and immune-mediated neuroinflammatory diseases, such as multiple sclerosis (MS) (23). Some of the findings correlate with ALS/FTD shifting the paradigm for understating neurodegeneration from a brain-centric to a multi-systemic process. In AD, myeloid cells like microglia perform a dual role, as observed in ALS. These cells are integral to clearing β-amyloid plaques (24), a characteristic of AD pathology, but can also drive harmful inflammation if chronically activated. Moreover, as demonstrated by Chen and colleagues, microglia can prime T cell infiltration in the brain during disease (2). Therapeutic exploration in AD aims to enhance the plaque-clearing functions of microglia while mitigating their inflammatory and immunogenic responses. Such nuanced approaches could help slow or modify AD progression by leveraging the protective aspects of immune function while limiting harm. In PD, it has been suggested that specific epitopes present on α-synuclein can elicit T cell response. Other studies indicate low CD8 T cell blood count is associated with disease severity. Changes in migration of CD4 T cells and alterations in CD4 T cell profiling have been observed in PD patients. Intriguingly, in a time course transcriptomic analysis of the CD4 T cells from PD patients, the IL-17 pathway is dysregulated (23), mirroring the results obtained in the Limone et al. study (for comprehensive review see DeMaio et al.). In HD, some studies have described a priming effect of the huntingtin protein on microglia, likely shaping its activation, and macrophages from HD models have been found hyper-reactive (25). MS, at last, comes as an exciting term of comparison. In MS, therapeutic strategies primarily focus on modulating the immune system to curb the autoimmunity at the heart of the disease (23). The involvement of lymphoid cells, such as T and B cells, in initiating and sustaining autoimmune attacks against myelin makes them primary targets. Disease-modifying therapies for MS typically aim to diminish the activity of these cells or inhibit their migration into the CNS. Although NDs are not yet seen as autoimmune diseases, some of the therapeutic interventions used to reduce neuroinflammation in MS can be devised as possibilities for the treatment of neurodegeneration. In general, it may be possible to discover new targeted therapies by understanding how myeloid and lymphoid cells interact with neural tissues and among themselves. Advanced imaging techniques and developing specific biomarkers to monitor immune cell activity could provide more accurate diagnosis and treatment strategies. Investigating genetic determinants influencing immune responses could lead to personalized treatments tailored to individual genetic profiles and specific disease manifestations.
Acknowledgments
Funding: None.
Footnote
Provenance and Peer Review: This article was commissioned by the editorial office, Annals of Translational Medicine. The article has undergone external peer review.
Peer Review File: Available at https://atm.amegroups.com/article/view/10.21037/atm-24-86/prf
Conflicts of Interest: Both authors have completed the ICMJE uniform disclosure form (available at https://atm.amegroups.com/article/view/10.21037/atm-24-86/coif). The authors have no conflicts of interest to declare.
Ethical Statement: The authors are accountable for all aspects of the work in ensuring that questions related to the accuracy or integrity of any part of the work are appropriately investigated and resolved.
Open Access Statement: This is an Open Access article distributed in accordance with the Creative Commons Attribution-NonCommercial-NoDerivs 4.0 International License (CC BY-NC-ND 4.0), which permits the non-commercial replication and distribution of the article with the strict proviso that no changes or edits are made and the original work is properly cited (including links to both the formal publication through the relevant DOI and the license). See: https://creativecommons.org/licenses/by-nc-nd/4.0/.
References
- Balak CD, Han CZ, Glass CK. Deciphering microglia phenotypes in health and disease. Curr Opin Genet Dev 2024;84:102146. [Crossref] [PubMed]
- Chen X, Firulyova M, Manis M, et al. Microglia-mediated T cell infiltration drives neurodegeneration in tauopathy. Nature 2023;615:668-77. [Crossref] [PubMed]
- Liddelow SA, Marsh SE, Stevens B. Microglia and Astrocytes in Disease: Dynamic Duo or Partners in Crime? Trends Immunol 2020;41:820-35. [Crossref] [PubMed]
- Cividini C, Basaia S, Spinelli EG, et al. Amyotrophic Lateral Sclerosis-Frontotemporal Dementia: Shared and Divergent Neural Correlates Across the Clinical Spectrum. Neurology 2022;98:e402-15. [Crossref] [PubMed]
- Renton AE, Majounie E, Waite A, et al. A hexanucleotide repeat expansion in C9ORF72 is the cause of chromosome 9p21-linked ALS-FTD. Neuron 2011;72:257-68. [Crossref] [PubMed]
- DeJesus-Hernandez M, Mackenzie IR, Boeve BF, et al. Expanded GGGGCC hexanucleotide repeat in noncoding region of C9ORF72 causes chromosome 9p-linked FTD and ALS. Neuron 2011;72:245-56. [Crossref] [PubMed]
- Gitler AD, Tsuiji H. There has been an awakening: Emerging mechanisms of C9orf72 mutations in FTD/ALS. Brain Res 2016;1647:19-29. [Crossref] [PubMed]
- Beckers J, Van Damme P. Toxic gain-of-function mechanisms in C9orf72 ALS-FTD neurons drive autophagy and lysosome dysfunction. Autophagy 2024;20:2102-4. [Crossref] [PubMed]
- O'Rourke JG, Bogdanik L, Yáñez A, et al. C9orf72 is required for proper macrophage and microglial function in mice. Science 2016;351:1324-9. [Crossref] [PubMed]
- McCauley ME, O'Rourke JG, Yáñez A, et al. C9orf72 in myeloid cells suppresses STING-induced inflammation. Nature 2020;585:96-101. [Crossref] [PubMed]
- Limone F, Couto A, Wang JY, et al. Myeloid and lymphoid expression of C9orf72 regulates IL-17A signaling in mice. Sci Transl Med 2024;16:eadg7895. [Crossref] [PubMed]
- Li X, Bechara R, Zhao J, et al. IL-17 receptor-based signaling and implications for disease. Nat Immunol 2019;20:1594-602. [Crossref] [PubMed]
- Soskic B, Jeffery LE, Kennedy A, et al. CD80 on Human T Cells Is Associated With FoxP3 Expression and Supports Treg Homeostasis. Front Immunol 2020;11:577655. [Crossref] [PubMed]
- Raulin AC, Doss SV, Trottier ZA, et al. ApoE in Alzheimer's disease: pathophysiology and therapeutic strategies. Mol Neurodegener 2022;17:72. [Crossref] [PubMed]
- Appel SH, Zhao W, Beers DR, et al. The microglial-motoneuron dialogue in ALS. Acta Myol 2011;30:4-8. [PubMed]
- Abellanas MA, Zamarbide M, Basurco L, et al. Midbrain microglia mediate a specific immunosuppressive response under inflammatory conditions. J Neuroinflammation 2019;16:233. [Crossref] [PubMed]
- Fu J, Huang Y, Bao T, et al. The role of Th17 cells/IL-17A in AD, PD, ALS and the strategic therapy targeting on IL-17A. J Neuroinflammation 2022;19:98. [Crossref] [PubMed]
- Beers DR, Zhao W, Wang J, et al. ALS patients' regulatory T lymphocytes are dysfunctional, and correlate with disease progression rate and severity. JCI Insight 2017;2:e89530. [Crossref] [PubMed]
- Beers DR, Henkel JS, Zhao W, et al. CD4+ T cells support glial neuroprotection, slow disease progression, and modify glial morphology in an animal model of inherited ALS. Proc Natl Acad Sci U S A 2008;105:15558-63. [Crossref] [PubMed]
- Thonhoff JR, Beers DR, Zhao W, et al. Expanded autologous regulatory T-lymphocyte infusions in ALS: A phase I, first-in-human study. Neurol Neuroimmunol Neuroinflamm 2018;5:e465. [Crossref] [PubMed]
- Murdock BJ, Famie JP, Piecuch CE, et al. NK cells associate with ALS in a sex- and age-dependent manner. JCI Insight 2021;6:e147129. [PubMed]
- Beers DR, Henkel JS, Zhao W, et al. Endogenous regulatory T lymphocytes ameliorate amyotrophic lateral sclerosis in mice and correlate with disease progression in patients with amyotrophic lateral sclerosis. Brain 2011;134:1293-314. [Crossref] [PubMed]
- DeMaio A, Mehrotra S, Sambamurti K, et al. The role of the adaptive immune system and T cell dysfunction in neurodegenerative diseases. J Neuroinflammation 2022;19:251. [Crossref] [PubMed]
- Feng W, Zhang Y, Wang Z, et al. Microglia prevent beta-amyloid plaque formation in the early stage of an Alzheimer's disease mouse model with suppression of glymphatic clearance. Alzheimers Res Ther 2020;12:125. [Crossref] [PubMed]
- Träger U, Andre R, Magnusson-Lind A, et al. Characterisation of immune cell function in fragment and full-length Huntington's disease mouse models. Neurobiol Dis 2015;73:388-98. [Crossref] [PubMed]