Idolizing the clearance of Amyloid-β by microglia
Introduction
With an increasingly aging population, the prevalence of dementia is on the rise. Alzheimer’s disease (AD) is the most common form of dementia, accounting for 60–70% of all cases. AD is characterized by the formation of neurofibrillary tangles of hyper-phosphorylated tau and of beta amyloid (Aβ) aggregates, referred to as plaques. Both are thought to incite neuroinflammation and neurodegeneration, resulting in progressive cognitive decline. The identification of genes involved in Aβ processing, and accumulation of Aβ in familial AD has triggered efforts to develop Aβ-based therapies. However, these have suffered so far from undesired side effects and limited efficacy (1). The lack of a significant decrease in cognitive decline in patients with reduced Aβ plaque-load following immunization against Aβ (2), emphasizes therefore the need to consider other possible pathophysiological mechanisms in AD.
ApoE and ApoE receptors in AD
One of the earliest identified, and still strongest, genetic association with general AD is that of Apolipoprotein E (ApoE). ApoE is a component of lipoproteins such as very low density lipoprotein and high density lipoprotein and has been implicated in cholesterol transport. Its transcription is regulated by liver X receptor (LXR) transcription factors in a tissue-dependent manner (3). Several ApoE isoforms exist, and it is now established that the ApoE2 allele is associated with a reduced AD risk, while carriers of the ApoE4 allele are at an increased risk to develop AD. Moreover, healthy ApoE4 carriers show accelerated aging of the central nervous system (CNS) as assessed by cognitive decline and reduced hippocampal volume (4,5).
Several mechanisms underlie the detrimental role of ApoE4 in AD. Aside from the suggested direct toxic effects of ApoE4 (4), decreased lipidation of ApoE might be also involved. ApoE is lipidated by the ATP binding cassette subfamily A member 1 (ABCA1)—which similar to ApoE is under transcriptional control by LXRs. Lipidation by ABCA1 is a central determinant of ApoE-mediated degradation of Aβ (6), and accordingly decreased lipidation of ApoE4 by ABCA1 has been linked to decreased clearance of Aβ (5). In contrast, stimulation of ApoE lipidation enhances Aβ degradation by microglia (6).
The lipidation status of ApoE also affects its affinity to its receptors, as more extensively reviewed in (7). The major ApoE receptors in the CNS are members of the Low Density Lipoprotein Receptor (LDLR) family, and binding of ApoE to its receptors is required for cellular clearance and transport of Aβ across the blood brain barrier (7). Evidence for the importance of the LDLR in Aβ clearance was recently demonstrated in a mouse model of AD in which overexpression of the LDLR lead to a reduction of ApoE levels, inhibition of Aβ accumulation, and reduced neuroinflammation (8). These effects appear to rely, at least in part, on Aβ transport across the blood brain barrier (9).
Given its well-studied role in peripheral cholesterol metabolism, it is surprising that the function of the LDLR in the CNS is less well characterized. In the periphery, the LDLR is the main receptor for hepatic uptake of low-density lipoprotein from the blood and is a central determinant of lipoprotein metabolism. Accordingly, mutations in this receptor are the leading cause of familial hypercholesterolemia and ensuing atherosclerosis. The level and activity of the LDLR are subject to both transcriptional and post-transcriptional regulation. Transcription of the LDLR is controlled by the Sterol Response Element Binding Protein (SREBP) transcription factors, whereas regulated degradation of the LDLR is induced by proprotein convertase subtilisin kexin 9 (PCSK9) and by the LXR-regulated gene Inducible Degrader of the LDLR (IDOL) (10).
The LXR-IDOL-LDLR axis in AD
The sterol-responsive LXRs are members of the nuclear receptor family of transcription factors. LXRs play an important role in maintenance of sterol homeostasis in the CNS (3), and loss of either LXRα or LXRβ exacerbates AD-like pathology in an Aβ amyloidosis mouse model (11). Conversely, pharmacological activation of LXRs has been shown to decrease amyloidosis, reduce neuroinflammation and improve cognitive function in mouse models of AD (3,6,12,13). The beneficial outcome of LXR activation in AD has been attributed to (I) repression of proinflammatory signaling; (II) increased expression of ApoE and ABCA1 and (III) increased lipidation of ApoE by ABCA1, which supports enhanced Aβ clearance (Figure 1). IDOL, like ABCA1 and ApoE, is a transcriptional target of LXRs (14). However, whether IDOL plays a role in the actions of LXRs in AD is not known. IDOL is an E3 ubiquitin ligase that stimulates ubiquitylation of the intracellular tail of the LDLR, and the closely related receptors VLDLR and APOER2 that are important for Reelin signaling, thereby targeting these receptors for lysosomal degradation (10,14,15). Reciprocally, genetic silencing of IDOL increases the level and activity of these receptors (14-16). Therefore, if IDOL inhibition increases LDLR, and higher levels of LDLR result in enhanced Aβ clearance and improved AD-like pathology (8), could inhibiting IDOL activity lead to beneficial outcomes in AD?
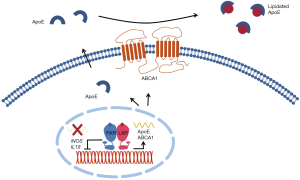
To address this question Choi and colleagues examined the role of IDOL in a mouse model of AD (17). In their paper, they show that when crossed with APP/PS1 mice, a model for Aβ amyloidosis, Idol(−/−) mice have higher levels of LDLR in the frontal cortex, less Aβ (both soluble and insoluble) and less neuroinflammation. The authors demonstrate that as a result of Idol loss the level of the LDLR, particularly in microglia, is increased. As a result, Idol(−/−) microglia display enhanced clearance and degradation of Aβ-bound ApoE-containing lipoproteins. Thus, the authors propose that enhanced microglial clearance of Aβ attenuates AD-like pathology in this mouse model (Figure 2). This study provides a plausible explanation for the conflicting reports on the role of LXR on Aβ clearance, as reviewed in (3). While global activation of the LXR program is beneficial in AD models (6,12,13), the current study suggests that LXR-stimulated induction of IDOL may actually increase Aβ deposition by limiting LDLR-mediated Aβ clearance thus counteracting the beneficial effects of LXR activation. Inhibiting IDOL activity may therefore provide a therapeutic opportunity to enhance Aβ clearance in AD. With this in mind, it is noteworthy that the effect of IDOL on several aspects of amyloidosis is gene-dose dependent, as APP/PS1/Idol(+/−) mice exhibit intermediate amyloidosis. From a therapeutic standpoint this is promising, as it suggests that partial inhibition of IDOL activity within the CNS may result in a beneficial outcome. As the first study to directly evaluate the role of Idol in AD this work raises several questions that need to be taken into account before this finding can be further developed.
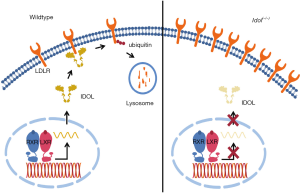
- Transcriptional regulation of IDOL and its activity shows strong, species-dependent differences, as also pointed out by the authors (18). For example, in primates the LXR-IDOL-LDLR axis strongly regulates hepatic lipoprotein metabolism, while this is not the case in mice. Studies in emerging primate models of AD are thus warranted. It may be also relevant to investigate whether genetic variation in the IDOL locus in humans associates with AD risk or severity, similar to what we have recently demonstrated for the association between IDOL and circulating levels of LDL (19);
- It would be interesting to clarify whether the effects of targeting IDOL in a model of AD rely on increased abundance of the LDLR solely, or also on its effect on the VLDLR and APOER2. These two receptors bind Reelin, an extracellular matrix protein implicated in neurogenesis and synaptic plasticity. A recent study demonstrated that conditional inactivation of Reelin in adult mice increased susceptibility to Aβ toxicity without raising the levels of Aβ itself (20). Therefore, it is tempting to speculate that the effect of targeting IDOL on AD pathology might rely on the synergistic effect of increased Aβ clearance by the LDLR and protection against Aβ-induced toxicity through enhanced Reelin signaling as a result of increased abundance of the VLDLR and APOER2;
- The current study, using Idol(−/−) mice, represents life-long “IDOL inhibition”. As AD patients present cognitive problems at a late stage and after development of the pathology, it would be interesting to assess the effects of IDOL inhibition after the onset of pathophysiology. Unfortunately, no small molecule inhibitor of IDOL has been reported to allow pharmacological testing in this setting. Yet in mouse models this can be studied by inducible inactivation of Idol. Halting, or slowing down AD development in mouse models by temporal inactivation of Idol at a time point when pathology has already initiated would provide strong support for IDOL inhibition in AD;
- The lack of behavioral and cognitive data is an important limitation of the current study. Decreased amyloidosis, as shown in APP/PS1/Idol(−/−) mice is not sufficient to assume beneficial cognitive effects, as was found in ApoE(−/−) and transgenic ApoE4 mice (5). Therefore, cognitive and behavioral studies of Idol(−/−) and of the APP/PS1/Idol(−/−) mice to complement the current findings are warranted.
Conclusions
Choi and colleagues are the first to describe a role for the E3 ubiquitin ligase IDOL in the pathophysiology of AD (17). Increased central abundance of the LDLR, potentially in convergent action with increased abundance of VLDLR and ApoER2, in Idol(−/−) mice could underlie the beneficial effects on amyloidosis and neuroinflammation. However, as discussed above further studies are eagerly awaited in order to evaluate targeting of IDOL as a therapeutic strategy in AD.
Acknowledgements
We thank Irith Koster for her comments and suggestions on this commentary. This work was supported by research grant [W.OR15] from the Prinses Beatrix Spierfonds, by an ERC Consolidator grant [617376] from the European Research Council and by Stichting Zabawas to NZ, who is an Established investigator of the Dutch Heart Association [2013T111].
Footnote
Conflicts of Interest: The authors have no conflicts of interest to declare.
References
- Lannfelt L, Relkin NR, Siemers ER. Amyloid-ß-directed immunotherapy for Alzheimer's disease. J Intern Med 2014;275:284-95. [Crossref] [PubMed]
- Holmes C, Boche D, Wilkinson D, et al. Long-term effects of Abeta42 immunisation in Alzheimer's disease: follow-up of a randomised, placebo-controlled phase I trial. Lancet 2008;372:216-23. [Crossref] [PubMed]
- Courtney R, Landreth GE. LXR Regulation of Brain Cholesterol: From Development to Disease. Trends Endocrinol Metab 2016;27:404-14. [Crossref] [PubMed]
- Michaelson DM. APOE ε4: the most prevalent yet understudied risk factor for Alzheimer's disease. Alzheimers Dement 2014;10:861-8. [Crossref] [PubMed]
- Huang Y, Mahley RW. Apolipoprotein E: structure and function in lipid metabolism, neurobiology, and Alzheimer's diseases. Neurobiol Dis 2014;72 Pt A:3-12.
- Jiang Q, Lee CY, Mandrekar S, et al. ApoE promotes the proteolytic degradation of Abeta. Neuron 2008;58:681-93. [Crossref] [PubMed]
- Kanekiyo T, Xu H, Bu G. ApoE and Aβ in Alzheimer's disease: accidental encounters or partners? Neuron 2014;81:740-54. [Crossref] [PubMed]
- Kim J, Castellano JM, Jiang H, et al. Overexpression of low-density lipoprotein receptor in the brain markedly inhibits amyloid deposition and increases extracellular A beta clearance. Neuron 2009;64:632-44. [Crossref] [PubMed]
- Castellano JM, Deane R, Gottesdiener AJ, et al. Low-density lipoprotein receptor overexpression enhances the rate of brain-to-blood Aβ clearance in a mouse model of β-amyloidosis. Proc Natl Acad Sci U S A 2012;109:15502-7. [Crossref] [PubMed]
- Sorrentino V, Zelcer N. Post-transcriptional regulation of lipoprotein receptors by the E3-ubiquitin ligase inducible degrader of the low-density lipoprotein receptor. Curr Opin Lipidol 2012;23:213-9. [Crossref] [PubMed]
- Zelcer N, Khanlou N, Clare R, et al. Attenuation of neuroinflammation and Alzheimer's disease pathology by liver x receptors. Proc Natl Acad Sci U S A 2007;104:10601-6. [Crossref] [PubMed]
- Fitz NF, Castranio EL, Carter AY, et al. Improvement of memory deficits and amyloid-β clearance in aged APP23 mice treated with a combination of anti-amyloid-β antibody and LXR agonist. J Alzheimers Dis 2014;41:535-49. [PubMed]
- Fitz NF, Cronican A, Pham T, et al. Liver X receptor agonist treatment ameliorates amyloid pathology and memory deficits caused by high-fat diet in APP23 mice. J Neurosci 2010;30:6862-72. [Crossref] [PubMed]
- Zelcer N, Hong C, Boyadjian R, et al. LXR regulates cholesterol uptake through Idol-dependent ubiquitination of the LDL receptor. Science 2009;325:100-4. [Crossref] [PubMed]
- Hong C, Duit S, Jalonen P, et al. The E3 ubiquitin ligase IDOL induces the degradation of the low density lipoprotein receptor family members VLDLR and ApoER2. J Biol Chem 2010;285:19720-6. [Crossref] [PubMed]
- Scotti E, Hong C, Yoshinaga Y, et al. Targeted disruption of the idol gene alters cellular regulation of the low-density lipoprotein receptor by sterols and liver x receptor agonists. Mol Cell Biol 2011;31:1885-93. [Crossref] [PubMed]
- Choi J, Gao J, Kim J, et al. The E3 ubiquitin ligase Idol controls brain LDL receptor expression, ApoE clearance, and Aβ amyloidosis. Sci Transl Med 2015;7:314ra184. [Crossref] [PubMed]
- Hong C, Marshall SM, McDaniel AL, et al. The LXR-Idol axis differentially regulates plasma LDL levels in primates and mice. Cell Metab 2014;20:910-8. [Crossref] [PubMed]
- Sorrentino V, Fouchier SW, Motazacker MM, et al. Identification of a loss-of-function inducible degrader of the low-density lipoprotein receptor variant in individuals with low circulating low-density lipoprotein. Eur Heart J 2013;34:1292-7. [Crossref] [PubMed]
- Lane-Donovan C, Philips GT, Wasser CR, et al. Reelin protects against amyloid β toxicity in vivo. Sci Signal 2015;8:ra67. [Crossref] [PubMed]