MET exon 14 juxtamembrane splicing mutations: clinical and therapeutical perspectives for cancer therapy
Introduction
Met belongs to a family of receptor tyrosine kinases (RTK) and it plays a crucial role in tissue remodeling and morphogenesis (1). Met RTK is physiologically activated by the binding of its specific ligand hepatocyte growth factor (HGF) (2), leading to the downstream induction of phosphoinositide 3-kinase (PI3K)/mammalian target of rapamycin (mTOR), signal transducer and activator of transcription (STAT) and mitogen-activated protein kinases (MAPK) pathways (3).
Aberrant Met/HGF regulation has been demonstrated in a wide variety of human cancers, enhancing various oncogenic processes including cell proliferation, survival, epithelial-mesenchymal transition (EMT), invasion and tumor angiogenesis (4-6).
The MET-associated aberrant signaling could be triggered by a variety of mechanisms, such as mutations, gene amplification and high gene copy number, increased Met/HGF protein expression and by the crosstalk with other dysregulated pathways affecting Met activation (7). Although rare, MET mutations have been detected in several types of cancer and can involve different domains of the gene, including the kinase part, the juxtamembrane (JM) and the extracellular domain (8,9).
MET exon 14 alterations include a heterogeneous group of mutations (affecting MET exon 14 but also its adjacent intronic regions), some of them altering the process of splicing producing a Met variant that lacks the exon 14 (10). The alternative splicing represents a physiological process that leads to the production of multiple protein isoforms from the same genetic information codified by a single gene (10). Although carefully regulated, a series of pathological mechanisms (as gene fusions, splice site mutations or mutations in genes encoding splicing factors) can trigger the production of alternative RNA transcripts sustaining the development of different types of disease, such as genetic disease (11) and cancer (12).
Unlike the majority of splice site mutations that lead to protein truncation and loss of function, MET exon 14 skipping mutations, inhibit the degradation of Met receptor, prolonging its oncogenic activity. This alteration might represent a novel class of actionable oncogenic event with potential clinical impact and therapeutical perspectives in patients affected by different cancer types.
Discovery and oncogenic potential of MET exon 14 alternative variants
The suggested role of the JM domain as a negative regulatory region of Met activity was initially examined by interpreting the emerging knowledge about TPR-MET fusion gene. The rearrangement between sequences encoding the translocated promoter region (TPR) and the Met kinase domain, results in a hybrid gene with oncogenic behavior, missing the JM domain that includes the Y1003 phosphorylation site (13,14). The phosphorylation of the tyrosine residue (Y1003) is a prerequisite for the recruitment of the Casitas B-lineage lymphoma (Cbl) E3 ubiquitin-ligase. The direct and irreplaceable relationship between Y1003 and Cbl binding was clearly demonstrated when the replacement of Y1003 by a phenylalanine residue abrogated the ubiquitination (15-17). Thus, in the absence of the JM domain, Cbl is unable to initiate the ubiquitination process of Met and therefore, the receptor remains constitutively active acquiring eventually an oncogenic activity (18,19).
Specific alterations in the MET exon 14 RNA splicing acceptor and donor sites are able to induce the exon 14 skipping with in-frame deletion of the JM domain. The MET gene transcript that misses the JM domain is 141 base-pair (bp) shorter than the normal transcript, producing a 47 amino acid smaller Met receptor (L964-D1010) (20,21).
The existence of somatic mutations involving the splicing sites of MET exon 14 was first described in primary lung cancer samples and lung cancer cell lines (20,22,23). In 2003, a novel sequence alteration in the JM domain, involving a 2-bp insertion in the pre-JM intron 13, was initially reported in two cases of small cell lung cancer (SCLC). The introduction of the MET JM alterations in SCLC cell lines has been demonstrated to have a crucial role in modulating cytoskeletal functions, enhancing cell proliferation and motility with significant implications in tumorigenicity and metastatic potential (22). Two years later, DNA sequencing analysis of non-small cell lung cancer (NSCLC) tumor tissues and cell lines revealed the existence of novel alterations harbored in different domains of Met (semaphorin and JM) along with an alternative skipping splice variant of the exon 14 due to an in-frame deletion of 141-bp. The selective targeting with a prototype small molecule inhibiting Met (SU11274) demonstrated a strong inhibitory activity on NSCLC cell proliferation through the blockade of Met/HGF signaling pathway (23).
In 2006, a MET mutational analysis on NSCLC samples and cell lines led to the identification of additional intronic alterations flanking exon 14, describing also the biological mechanism leading to the increased oncogenic potential of Met receptor (20). Cell lines transfected with MET exon 14 skipping variant exhibited attenuated ubiquitination and degradation of Met, resulting in an increased accumulation of the receptor compared with MET wild-type (WT) cells. A MET exon 14-deleted NSCLC cell line (H596) exhibited prolonged, HGF-dependent signaling activation in in vitro and in vivo models and was potentially druggable with Met inhibitors (20).
Further analyses reported a group of heterogeneous alterations that could induce MET exon 14 skipping, including base substitutions and deletions disrupting the splicing sites of introns 13 or 14 (24).
Although initially described and mostly investigated in lung cancer, the presence of MET exon 14 alterations might trigger oncogenic events also in other malignancies such as sarcomatoid tumor, gastrointestinal cancer, brain and nervous system cancer and other tumor types. To date, several tumor samples series have been analyzed for MET exon 14 alternative splicing variants (Table 1).
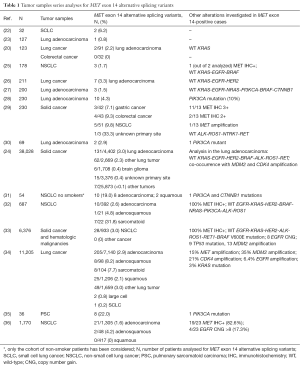
Full table
Lung cancer
In lung cancer, the deeper understanding of its biology and molecular pathways over years of basic and translational research led to the validation of MET proto-oncogene as a crucial player in term of both incidence and biological impact on the tumorigenic process (37). Nevertheless, although the promising clinical activity observed with anti-Met kinase inhibitors (as tivantinib) and monoclonal antibodies (onartuzumab) in early phase clinical trials (38,39), no clinically impactful benefit has been achieved to date in the context of larger phase III studies (40,41). The lack of activity regardless of Met protein overexpression suggested that its reliability as a predictive biomarker in targeted treatment with Met inhibitors is still debatable.
To date, MET exon 14 oncogenic alterations have been reported in approximately the 4% of lung cancer (25-27). According to the Cancer Genome Atlas (TCGA) project, the 4.3% of lung adenocarcinoma harbors DNA alterations with MET exon 14 skipping, confirmed by RNA analysis (28). The comprehensive clinical genomic profiling of 38,028 advanced cancer patients identified 221 cases with MET exon 14 skipping mutations (0.6%), astoundingly encompassing 126 different genomic sequence variants, that were distributed most commonly in lung adenocarcinoma (3.0%), other lung tumors (2.3%), brain glioma (0.4%) and tumors of unknown primary origin (0.4%) (24). Splice donor site alterations (base substitutions and indels) represent the most common cause of MET exon 14 skipping (approximately the 45% of the cases). Interestingly, MET exon 14 mutations are mutually exclusive with other frequently occurring genomic alterations in lung cancer (such as activating mutations in KRAS, EGFR, HER2, BRAF and rearrangement in ALK, ROS1 and RET), suggesting the oncogenic relevance of this specific Met alteration. In vitro studies demonstrated that cells harboring MET exon 14 alterations are strongly sensitive to capmatinib (INC280), a highly selective and potent Met inhibitor. Conversely, cell lines carrying both MET and HRAS mutation resulted in resistance to capmatinib and sensitivity to trametinib, a MEK inhibitor, confirming the selectivity of capmatinib in inhibiting the growth of Met-dependent cells (24).
In another recent study, lung adenocarcinoma cell lines with ALK rearrangement, ROS1 rearrangement or MET amplification showed in vitro response to crizotinib and ceritinib (an ALK/ROS1 inhibitor) (30). The evaluation of a cohort of patients affected by lung adenocarcinoma performed in the same analysis revealed that putative genomic aberrations potentially targetable by crizotinib (ALK and ROS1 rearrangements, high level MET amplification or MET exon 14 skipping mutations) are present in the 10% of cases. MET exon 14 skipping mutation was reported to predict response to anti-Met tyrosine kinase inhibitor (TKI) in lung adenocarcinomas (30).
Another series of 687 NSCLC patients has been comprehensively analyzed for MET alterations (MET exon 14 skipping mutations, MET gene copy number and protein expression), focusing on the potential clinical implication of MET alterations in different histological subgroups of NSCLC (32). MET exon 14 skipping mutations were identified in the 2.6% of NSCLC with a differential frequency according to histology: 2.6% in adenocarcinoma, 4.8% in adenosquamous carcinoma and 31.8% in sarcomatoid carcinoma. Although MET mutations occur mutually exclusively with other validated oncogenic drivers, a coexistence with MET amplification and high gene copy number has been demonstrated. Globally considered, MET mutation and high-level amplification can be detected in the 3.3% of NSCLC (associated with protein overexpression) and both represent independent prognostic factors predicting poor survival at the multivariable analysis (32).
A recent study explored the clinico-pathologic and genomic features of patients affected by MET exon 14-mutant cancer (33). MET exon 14 mutations were identified only in non-squamous NSCLC, of which they represent 3% of cases. Although the results are strongly limited in terms of generalizability by the small size of the patients’ cohort (n=28), the MET exon 14 splicing alterations seem to occur in older adults (median age: 72.5 years) with a history of tobacco use (64% of cases) (33). Nevertheless, in the context of a small cohort of 54 never-smoker patients affected by lung cancers that were WT for other validated driver alterations, MET exon 14 skipping mutations were detected in 19% of the cases (31).
A comprehensive genomic profiling approach was applied in a large set of 11,205 lung tumor samples and 298 (2.7%) cases were identified harboring genetic alterations, including base substitutions and indels, which could determine the production of the MET exon 14 skipping variant. These alterations were most frequently described in adenosquamous histology (8.2%), followed by the sarcomatoid subtype (7.7%). Interestingly, this is the first study reporting MET exon 14 genetic alterations in squamous cell lung cancer (2.1%). Concurrent MET amplification was observed in the 15% of MET exon 14-deleted cases with further differences in term of mutational load and type of MET alterations between amplified and not amplified cases. Impressive symptomatic and radiographic responses were reported in patients upon treatment with crizotinib, regardless of the MET amplification status (34).
Another recent study cohort of 1,770 patients was designed in order to recognize those clinical and pathological parameters that characterize the NSCLC MET exon 14 skipping patients. The genetic screening identified 23 (1.3%) patients positive for MET exon 14 skipping alterations, all displaying similar features (female, non-smoker, older age and earlier pathology stage) along with a longer survival than the KRAS positive patients. Additionally, the 80% of the positive cases had EGFR copy gains. Interestingly, a patient with MET exon 14 skipping along with EGFR copy number gain (CNG) relapsed and received an EGFR inhibitor, obtaining a significant response (36).
Globally considered, available preclinical and clinical data provide a strong rationale supporting the use of TKI to target human cancer with MET exon 14 skipping mutations.
In this regard, clinically significant tumor response or stable disease have been reported with off-label use of anti-Met TKI, such as crizotinib or cabozantinib, in a series of patients affected by metastatic NSCLC harboring MET exon 14 skipping mutations (24,42-46). The clinical features of the patients described in the published case reports are heterogeneous and MET exon 14 skipping mutations have been described not only in patients affected by lung adenocarcinoma (42,43,45,46), but also with squamous cell carcinoma (24,44), large cell carcinoma (24) and histiocytic sarcoma (24), all benefiting from the targeted treatment with Met inhibitors.
Further supporting the notion that MET exon 14 skipping-mutant lung adenocarcinoma might represent an oncogene-addicted disease, impressive examples of “Lazarus-type” response have been reported with the use of crizotinib in such patients, regardless of the performance status of the patient (47). This clinically-astonishing phenomenon, associated with a rapid clinico-radiographic disease improvement, was firstly described in EGFR-mutant and ALK-rearranged lung cancer patients treated with the specific genomically-matched TKI (48). The emerging belief is that MET exon 14 mutations might represent a similarly strong oncogenic stimulus with an equally robust clinical relevance and therapeutic consequence.
Recently, the preliminary activity and safety data regarding 17 patients with MET exon 14-altered NSCLC, enrolled into the expansion cohort of the PROFILE 1001 study and treated with crizotinib, became available. Median age was 68 years, the prevalent tumor histology was adenocarcinoma (71%) and all the patients were former or never smokers. Crizotinib demonstrated antitumor activity in 10 out of 15 patients with a generally tolerable adverse events profile (49).
Innovative clinical trials are currently ongoing evaluating the activity and safety of novel Met inhibitors (as capmatinib and tepotinib) in patients affected by NSCLC harboring MET exon 14 skipping alterations.
Sarcomatoid tumors
Sarcomatoid carcinoma is a rare type of malignant tumor, composed by a combination of spinal and epithelial cells. Considering the poor efficacy of chemotherapy and radiotherapy in this type of cancer, the identification of potentially druggable molecular alterations represents an important unmet need (50). Preliminary evidence demonstrated that, although no mutations were reported in exons 14–21 of MET gene, after screening seven cell lines and 32 tumor samples of rhabdomyosarcoma, high MET RNA and protein level was identified, supporting the oncogenic contribution of MET in the rhabdomyosarcoma progression (51).
Sarcomatoid carcinoma of the lung is a rare, poorly differentiated, subtype of lung cancer and constitutes approximately the 1% of NSCLC (52). In comparison with the relative low frequency of detection observed in “classic” lung cancer (numerically comparable to the ALK rearrangement frequency), a strikingly high rate of MET exon 14 skipping mutations, mutually exclusive with other validated driver alterations, was most recently detected in pulmonary sarcomatoid carcinoma (PSC) (22%) (35,52,53). Moreover, an impressive clinico-radiological response was described in a woman affected by an advanced chemotherapy resistant PSC harboring a MET exon 14 skipping mutation, providing further strong proof of the clinical relevance of the findings (35). Similarly, in another series of lung cancer patients, MET exon 14 skipping mutations were identified in 31.8% of the sarcomatoid carcinoma (32).
Gastrointestinal cancer
Among gastrointestinal malignancies, particularly in gastric cancer the aberrant signaling of MET by overexpression or gene amplification has been detected and correlated with tumor progression and patients’ survival (54,55). To date, some evidence of activity has been reported with anti-Met antibodies (onartuzumab and rilotumumab) in patients affected by gastric cancer, particularly if stratified for Met positivity by immunohistochemistry (IHC) (56,57).
Further research in gastrointestinal malignancies demonstrates for the first time the existence of MET exon 14 deletion in gastric and colon cancer. A series of 230 solid tumor specimens, including 42 gastric and 43 colon cancer samples, was examined using multiplexed fusion transcript detection assay, confirmed with reverse transcription PCR (RT-PCR) and then correlated with Met protein overexpression (29). The data generated by quantitative RT-PCR confirmed the presence of the MET exon 14 skipping mutations in three cases (7.1%) in gastric cancer and five cases (9.3%) in colon cancer. Interestingly, all the MET exon 14 positive cases showed also Met protein overexpression, without the coexistence of a MET amplification (reported in only one case). Moreover, as described in lung cancer (24), MET exon 14 skipping mutations occur mutually exclusively with other validated drivers, thus supporting its oncogenic implication and defining a distinct molecular subgroup of gastrointestinal malignancies (29). In the preclinical analysis included in the study, the growth of two patient-derived tumor cell lines harboring MET exon 14 deletion (one from gastric and one from colon cancer) were strongly inhibited by both Met TKI and an anti-Met monoclonal antibody (29). Furthermore, two independent preclinical studies reported the presence of MET exon 14 deletion in gastric cancer cell lines (58,59). In particular, a genome-wide analysis of 34 gastric cancer cell lines showed that only one cell line (Hs746T) has a splice site mutation of MET exon 14, concurrent with MET amplification and protein overexpression (58). These results provide preliminary evidence that MET exon 14 skipping alterations might act as a driver mutation in some gastrointestinal malignancies and put the bases for further research in the field.
Brain and nervous system cancer
Different studies have reported the existence of a MET aberrant activity in human neuroblastoma tissue samples and cell lines (60-62). An analysis of human neuroblastoma samples aimed to identify different MET-spliced isoforms, revealed the presence of MET exon 14-deleted isoforms in approximately the 2% of samples (63). The available preliminary findings support the correlation of MET gene with neuroblastoma tumorigenesis, providing additional information about MET as a potential therapeutic target in this cancer.
Although MET exon 14 splicing variants have not been identified to date in glioblastoma (GBM), alterations in the MET gene (such as amplifications and gene fusions), resulting in the constitutive activation of the Met receptor, have been reported (64,65). Moreover, a novel intragenic variant of Met-deleted receptor was found overexpressed in the 6% of high-grade gliomas. This protein results from an aberrant splice event connecting the splice donor site in exon 6 with the splice acceptor site in exon 9, skipping part of the extracellular domain encoded by exon 7 and 8. The resulting MET exon 7–8 skipping variant is constitutively active and lacks membranous expression, therefore it is not targetable with antibodies against Met and/or HGF, but efficiently deactivated by Met-specific TKIs (as cabozantinib) (66).
Other tumors
Hereditary renal cell carcinoma (RCC) with papillary histotype represents the first solid tumor where MET mutations were identified (67). The majority of missense mutations were located in the tyrosine kinase domain (exons 16–21) of the MET gene, both in patients affected by hereditary papillary RCC and in a small subgroup of patients with sporadic papillary RCC (67,68). Moreover, the duplication of chromosome 7 represents an alternative mechanism enhancing the MET signaling in most of sporadic papillary RCC cases (69). Recently, the comprehensive molecular characterization (TCGA Research Network) of 161 primary papillary RCC reported that MET mutations are characteristic of type 1 tumors and confirmed that the mutations were mainly located in the tyrosine kinase domain (70). With regard to exon 14, this analysis reported a germline C to T sequence change (T1010I) in a patient with a family history of papillary RCC. Nevertheless, this sequence change has been considered a potential rare polymorphism instead of an oncogenic event since the change did not segregate with the disease (69). Considering the potential oncogenic role of MET in this disease, patients with advanced papillary RCC treated with foretinib, demonstrated an overall response rate of 13.5%, although it did not meet the 25% predefined rate, with a remarkable activity in patients with germline MET mutations (71). In clear-cell RCC, the interest about MET alterations is related to the potential role of Met protein overexpression in the development of resistance to antiangiogenic TKI (72). In this regard, US Food and Drug Administration has approved cabozantinib for patients with advanced RCC who have received a prior antiangiogenic therapy (73,74).
Similarly to clear-cell RCC, MET mutations (and specifically in the exon 14) have not been identified in prostate cancer, while Met expression has been correlated with the castration-resistant tumor proliferation (75,76). Although pre-clinical and early phase trials suggested a potential activity of Met inhibitors in prostate cancer, phase III trials, such as the COMET-1, failed to demonstrate positive results (77).
For what concerns breast cancer (BC), tumors with Met overexpression are associated with faster tumor progression and worse prognosis compared to tumors without MET alterations (78,79). Moreover, this abnormality more frequently characterized basal-like BC subtypes instead of luminal and HER2-positive tumors (80). Although few evidence is available regarding the frequency and role of MET alterations in BC, in vitro and in vivo models suggest that they are actually uncommon (81). In this regard, the analysis of a cohort of 104 patients with advanced BC identified MET mutations and amplifications in the 9% and 4.7% of patients, respectively. Two out of eight patients with MET mutation harbored a missense MET sequence alteration (T1010I), located in exon 14 (82). A previous study, screening for MET exons 14 and 16–20 mutations in 30 samples of primary BC, found one tumor with a T1010I mutation (83). The potential role of MET pathway in tumor progression together with the need of novel therapeutic strategy, led to investigate anti-Met drugs in BC, especially in triple-negative and basal-like subtypes. Although some encouraging results have been obtained in preclinical studies (84), to date no reliable evidence of clinical activity are available for Met inhibitors in BC (85,86).
Differently from normal thyroid tissue and non-neoplastic conditions, thyroid carcinomas, papillary in particular, express high level of MET RNA and protein, suggesting that increased MET signaling might be important in the molecular pathogenesis of differentiated thyroid carcinoma (87-89). The analysis of the mutational profile of MET exons 2–21 performed in 104 thyroid samples revealed six cases harboring the same genetic alteration. All the cases were characterized by a missense mutation, which was a base substitution in the codon 1010 (T1010I) of exon 14. According to previously evidence (22), T1010I mutation induces oncogenic characteristics in tumor cell lines and therefore the presence of this alteration in thyroid cancer patients, even if it does not produce a JM-missing variant, might be considered as a MET exon 14 actionable mutation rather than a single nucleotide polymorphism (90).
Conclusions
Globally considered, the available data support that MET exon 14 alterations are likely to represent a novel and actionable oncogenic target, by driving the development of a small (but clinically significant) proportion of patients affected by different tumor types. Moreover, similarly to the MET amplification, it is already demonstrated that MET exon 14 skipping alteration confers sensitivity to Met inhibitors (such as crizotinib, cabozantinib and capmatinib).
In lung cancer, MET exon 14 skipping mutations represent the next largest actionable subgroups in NSCLC (approximately 4%), after EGFR activating mutations. Interestingly, the available data supports the correlation between MET driver mutations and the development of malignancies with a biologically impactful sarcomatoid component, related to a highly aggressive behavior with resistance to standard treatment. This observation raises intriguing scientific perspectives for the evaluation of MET alterations in sarcomatoid malignancies arising from different organs, which are likely to have important therapeutic implications. A potential oncogenic role of MET exon 14-skipping variant is also under investigation in gastrointestinal and nervous system cancer. Moreover, although preliminary evidence does not support the oncogenic role of MET exon 14 in other types of cancer, such as BC and urologic malignancies, further analyses in larger tumor samples series are mandatory in order to clarify this issue. Nevertheless, the impressive clinical benefit observed in MET exon 14-mutant lung cancer patients treated with selective TKI, has not yet been observed in other malignancies, supporting a potential different impact of this alterations according to the type of tumor.
According to this evidence, the identification of this featured patients’ subgroup, affected by cancer strongly depending from the MET signaling, is clinically and ethically relevant in order to match patients to their effective treatment in the era of precision medicine. Thus, patients affected by lung cancer and other malignancies harboring these MET exon 14 alterations should be prioritized to be enrolled in clinical trials with anti-Met targeted therapy.
The heterogeneity of MET exon 14 alterations requires the development and validation of reliable detection methods for diagnostic and clinical trials selection. Moreover, with the growing list of effective oncogenic targets potentially druggable with an increasing amount of innovative molecules, a comprehensive genomic profile of the tumor would soon become necessary and essential to stratify patients for the most appropriate and effective treatment.
To date, the exon profiling has provided the major knowledge about the biological mechanisms underlying oncogenic processes, allowing impressive advances in cancer treatment. In this regard, besides the identification of a new oncogenic driver, the discovery of MET exon 14 skipping mutations revealed the unseen clinical potential to explore the whole genome with comprehensive platforms, in order to study also those mutations affecting RNA processing.
Acknowledgements
Funding: This work was partially supported by a grant of the Italian Association for Cancer Research (AIRC-MFAG 14282) and a fellowship award of the International Association for Lung Cancer (IASLC).
Footnote
Conflicts of Interest: The authors have no conflicts of interest to declare.
References
- Jeffers M, Rong S, Vande Woude GF. Hepatocyte growth factor/scatter factor-Met signaling in tumorigenicity and invasion/metastasis. J Mol Med (Berl) 1996;74:505-13. [Crossref] [PubMed]
- Fajardo-Puerta AB, Mato Prado M, Frampton AE, et al. Gene of the month: HGF. J Clin Pathol 2016;69:575-9. [Crossref] [PubMed]
- Organ SL, Tsao MS. An overview of the c-MET signaling pathway. Ther Adv Med Oncol 2011;3:S7-S19. [Crossref] [PubMed]
- Gherardi E, Birchmeier W, Birchmeier C, et al. Targeting MET in cancer: rationale and progress. Nat Rev Cancer 2012;12:89-103. [Crossref] [PubMed]
- Appleman LJ. MET signaling pathway: a rational target for cancer therapy. J Clin Oncol 2011;29:4837-8. [Crossref] [PubMed]
- Gentile A, Trusolino L, Comoglio PM. The Met tyrosine kinase receptor in development and cancer. Cancer Metastasis Rev 2008;27:85-94. [Crossref] [PubMed]
- Maroun CR, Rowlands T. The Met receptor tyrosine kinase: a key player in oncogenesis and drug resistance. Pharmacol Ther 2014;142:316-38. [Crossref] [PubMed]
- Ma PC, Tretiakova MS, MacKinnon AC, et al. Expression and mutational analysis of MET in human solid cancers. Genes Chromosomes Cancer 2008;47:1025-37. [Crossref] [PubMed]
- Feng Y, Thiagarajan PS, Ma PC. MET signaling: novel targeted inhibition and its clinical development in lung cancer. J Thorac Oncol 2012;7:459-67. [Crossref] [PubMed]
- Drilon A. MET Exon 14 Alterations in Lung Cancer: Exon Skipping Extends Half-Life. Clin Cancer Res 2016;22:2832-4. [Crossref] [PubMed]
- Tuffery-Giraud S, Chambert S, Demaille J, et al. Point mutations in the dystrophin gene: evidence for frequent use of cryptic splice sites as a result of splicing defects. Hum Mutat 1999;14:359-68. [Crossref] [PubMed]
- Srebrow A, Kornblihtt AR. The connection between splicing and cancer. J Cell Sci 2006;119:2635-41. [Crossref] [PubMed]
- Cooper CS, Park M, Blair DG, et al. Molecular cloning of a new transforming gene from a chemically transformed human cell line. Nature 1984;311:29-33. [Crossref] [PubMed]
- Soman NR, Correa P, Ruiz BA, et al. The TPR-MET oncogenic rearrangement is present and expressed in human gastric carcinoma and precursor lesions. Proc Natl Acad Sci U S A 1991;88:4892-6. [Crossref] [PubMed]
- Peschard P, Fournier TM, Lamorte L, et al. Mutation of the c-Cbl TKB domain binding site on the Met receptor tyrosine kinase converts it into a transforming protein. Mol Cell 2001;8:995-1004. [Crossref] [PubMed]
- Peschard P, Ishiyama N, Lin T, et al. A conserved DpYR motif in the juxtamembrane domain of the Met receptor family forms an atypical c-Cbl/Cbl-b tyrosine kinase binding domain binding site required for suppression of oncogenic activation. J Biol Chem 2004;279:29565-71. [Crossref] [PubMed]
- Weidner KM, Sachs M, Riethmacher D, et al. Mutation of juxtamembrane tyrosine residue 1001 suppresses loss-of-function mutations of the met receptor in epithelial cells. Proc Natl Acad Sci U S A 1995;92:2597-601. [Crossref] [PubMed]
- Lee JH, Gao CF, Lee CC, et al. An alternatively spliced form of Met receptor is tumorigenic. Exp Mol Med 2006;38:565-73. [Crossref] [PubMed]
- Peschard P, Park M. Escape from Cbl-mediated downregulation: a recurrent theme for oncogenic deregulation of receptor tyrosine kinases. Cancer cell 2003;3:519-23. [Crossref] [PubMed]
- Kong-Beltran M, Seshagiri S, Zha J, et al. Somatic mutations lead to an oncogenic deletion of met in lung cancer. Cancer Res 2006;66:283-9. [Crossref] [PubMed]
- Lee CC, Yamada KM. Identification of a novel type of alternative splicing of a tyrosine kinase receptor. Juxtamembrane deletion of the c-met protein kinase C serine phosphorylation regulatory site. J Biol Chem 1994;269:19457-61. [PubMed]
- Ma PC, Kijima T, Maulik G, et al. c-MET mutational analysis in small cell lung cancer: novel juxtamembrane domain mutations regulating cytoskeletal functions. Cancer Res 2003;63:6272-81. [PubMed]
- Ma PC, Jagadeeswaran R, Jagadeesh S, et al. Functional expression and mutations of c-Met and its therapeutic inhibition with SU11274 and small interfering RNA in non-small cell lung cancer. Cancer Res 2005;65:1479-88. [Crossref] [PubMed]
- Frampton GM, Ali SM, Rosenzweig M, et al. Activation of MET via diverse exon 14 splicing alterations occurs in multiple tumor types and confers clinical sensitivity to MET inhibitors. Cancer Discov 2015;5:850-9. [Crossref] [PubMed]
- Okuda K, Sasaki H, Yukiue H, et al. Met gene copy number predicts the prognosis for completely resected non-small cell lung cancer. Cancer Sci 2008;99:2280-5. [Crossref] [PubMed]
- Onozato R, Kosaka T, Kuwano H, et al. Activation of MET by gene amplification or by splice mutations deleting the juxtamembrane domain in primary resected lung cancers. J Thorac Oncol 2009;4:5-11. [Crossref] [PubMed]
- Seo JS, Ju YS, Lee WC, et al. The transcriptional landscape and mutational profile of lung adenocarcinoma. Genome Res 2012;22:2109-19. [Crossref] [PubMed]
- Cancer Genome Atlas Research Network. Comprehensive molecular profiling of lung adenocarcinoma. Nature 2014;511:543-50. [Crossref] [PubMed]
- Lee J, Ou SH, Lee JM, et al. Gastrointestinal malignancies harbor actionable MET exon 14 deletions. Oncotarget 2015;6:28211-22. [Crossref] [PubMed]
- Jorge SE, Schulman S, Freed JA, et al. Responses to the multitargeted MET/ALK/ROS1 inhibitor crizotinib and co-occurring mutations in lung adenocarcinomas with MET amplification or MET exon 14 skipping mutation. Lung cancer 2015;90:369-74. [Crossref] [PubMed]
- Heist RS, Shim HS, Gingipally S, et al. MET Exon 14 Skipping in Non-Small Cell Lung Cancer. Oncologist 2016;21:481-6. [Crossref] [PubMed]
- Tong JH, Yeung SF, Chan AW, et al. MET Amplification and Exon 14 Splice Site Mutation Define Unique Molecular Subgroups of Non-Small Cell Lung Carcinoma with Poor Prognosis. Clin Cancer Res 2016;22:3048-56. [Crossref] [PubMed]
- Awad MM, Oxnard GR, Jackman DM, et al. MET Exon 14 Mutations in Non-Small-Cell Lung Cancer Are Associated With Advanced Age and Stage-Dependent MET Genomic Amplification and c-Met Overexpression. J Clin Oncol 2016;34:721-30. [Crossref] [PubMed]
- Schrock AB, Frampton GM, Suh J, et al. Characterization of 298 Patients with Lung Cancer Harboring MET Exon 14 Skipping Alterations. J Thorac Oncol 2016;11:1493-502. [Crossref] [PubMed]
- Liu X, Jia Y, Stoopler MB, et al. Next-Generation Sequencing of Pulmonary Sarcomatoid Carcinoma Reveals High Frequency of Actionable MET Gene Mutations. J Clin Oncol 2016;34:794-802. [Crossref] [PubMed]
- Zheng D, Wang R, Ye T, et al. MET exon 14 skipping defines a unique molecular class of non-small cell lung cancer. Oncotarget 2016;7:41691-702. [PubMed]
- Sadiq AA, Salgia R. MET as a possible target for non-small-cell lung cancer. J Clin Oncol 2013;31:1089-96. [Crossref] [PubMed]
- Sequist LV, von Pawel J, Garmey EG, et al. Randomized phase II study of erlotinib plus tivantinib versus erlotinib plus placebo in previously treated non-small-cell lung cancer. J Clin Oncol 2011;29:3307-15. [Crossref] [PubMed]
- Spigel DR, Ervin TJ, Ramlau RA, et al. Randomized phase II trial of Onartuzumab in combination with erlotinib in patients with advanced non-small-cell lung cancer. J Clin Oncol 2013;31:4105-14. [Crossref] [PubMed]
- Scagliotti G, von Pawel J, Novello S, et al. Phase III Multinational, Randomized, Double-Blind, Placebo-Controlled Study of Tivantinib (ARQ 197) Plus Erlotinib Versus Erlotinib Alone in Previously Treated Patients With Locally Advanced or Metastatic Nonsquamous Non-Small-Cell Lung Cancer. J Clin Oncol 2015;33:2667-74. [Crossref] [PubMed]
- Spigel DR, Edelman MJ, O'Byrne K, et al. Onartuzumab plus erlotinib versus erlotinib in previously treated stage IIIb or IV NSCLC: Results from the pivotal phase III randomized, multicenter, placebo-controlled METLung (OAM4971g) global trial. J Clin Oncol 2014;32:abstr 8000.
- Paik PK, Drilon A, Fan PD, et al. Response to MET inhibitors in patients with stage IV lung adenocarcinomas harboring MET mutations causing exon 14 skipping. Cancer Discov 2015;5:842-9. [Crossref] [PubMed]
- Jenkins RW, Oxnard GR, Elkin S, et al. Response to Crizotinib in a Patient With Lung Adenocarcinoma Harboring a MET Splice Site Mutation. Clin Lung Cancer 2015;16:e101-4. [Crossref] [PubMed]
- Mendenhall MA, Goldman JW. MET-Mutated NSCLC with Major Response to Crizotinib. J Thorac Oncol 2015;10:e33-4. [Crossref] [PubMed]
- Waqar SN, Morgensztern D, Sehn J. MET Mutation Associated with Responsiveness to Crizotinib. J Thorac Oncol 2015;10:e29-31. [Crossref] [PubMed]
- Mahjoubi L, Gazzah A, Besse B, et al. A never-smoker lung adenocarcinoma patient with a MET exon 14 mutation (D1028N) and a rapid partial response after crizotinib. Invest New Drugs 2016;34:397-8. [Crossref] [PubMed]
- Shea M, Huberman MS, Costa DB. Lazarus-Type Response to Crizotinib in a Patient with Poor Performance Status and Advanced MET Exon 14 Skipping Mutation-Positive Lung Adenocarcinoma. J Thorac Oncol 2016;11:e81-2. [Crossref] [PubMed]
- Langer CJ. The "lazarus response" in treatment-naive, poor performance status patients with non-small-cell lung cancer and epidermal growth factor receptor mutation. J Clin Oncol 2009;27:1350-4. [Crossref] [PubMed]
- Drilon AE, Camidge DR, Ou SH, et al. Efficacy and safety of crizotinib in patients (pts) with advanced MET exon 14-altered non-small cell lung cancer (NSCLC). J Clin Oncol 2016;34:abstr 108.
- Zhu CC, Li MR, Lin TL, et al. Sarcomatoid carcinoma of the stomach: A case report and literature review. Oncol Lett 2015;10:1385-9. [PubMed]
- Chen Y, Takita J, Mizuguchi M, et al. Mutation and expression analyses of the MET and CDKN2A genes in rhabdomyosarcoma with emphasis on MET overexpression. Genes Chromosomes Cancer 2007;46:348-58. [Crossref] [PubMed]
- Wislez M, Domblides C, Cortot A, et al. Mutations at the splice sites of exon 14 of MET gene: a new target for sarcomatoid carcinomas? Ann Transl Med 2016;4:96. [Crossref] [PubMed]
- Lee C, Usenko D, Frampton GM, et al. MET 14 Deletion in Sarcomatoid Non-Small-Cell Lung Cancer Detected by Next-Generation Sequencing and Successfully Treated with a MET Inhibitor. J Thorac Oncol 2015;10:e113-4. [Crossref] [PubMed]
- Lee HE, Kim MA, Lee HS, et al. MET in gastric carcinomas: comparison between protein expression and gene copy number and impact on clinical outcome. Br J Cancer 2012;107:325-33. [Crossref] [PubMed]
- Lee J, Seo JW, Jun HJ, et al. Impact of MET amplification on gastric cancer: possible roles as a novel prognostic marker and a potential therapeutic target. Oncol Rep 2011;25:1517-24. [PubMed]
- Catenacci DV, Henderson L, Xiao SY, et al. Durable complete response of metastatic gastric cancer with anti-Met therapy followed by resistance at recurrence. Cancer Discov 2011;1:573-9. [Crossref] [PubMed]
- Iveson T, Donehower RC, Davidenko I, et al. Rilotumumab in combination with epirubicin, cisplatin, and capecitabine as first-line treatment for gastric or oesophagogastric junction adenocarcinoma: an open-label, dose de-escalation phase 1b study and a double-blind, randomised phase 2 study. Lancet Oncol 2014;15:1007-18. [Crossref] [PubMed]
- Asaoka Y, Tada M, Ikenoue T, et al. Gastric cancer cell line Hs746T harbors a splice site mutation of c-Met causing juxtamembrane domain deletion. Biochem Biophys Res Commun 2010;394:1042-6. [Crossref] [PubMed]
- Zang ZJ, Ong CK, Cutcutache I, et al. Genetic and structural variation in the gastric cancer kinome revealed through targeted deep sequencing. Cancer Res 2011;71:29-39. [Crossref] [PubMed]
- Crosswell HE, Dasgupta A, Alvarado CS, et al. PHA665752, a small-molecule inhibitor of c-Met, inhibits hepatocyte growth factor-stimulated migration and proliferation of c-Met-positive neuroblastoma cells. BMC Cancer 2009;9:411. [Crossref] [PubMed]
- Hecht M, Papoutsi M, Tran HD, et al. Hepatocyte growth factor/c-Met signaling promotes the progression of experimental human neuroblastomas. Cancer Res 2004;64:6109-18. [Crossref] [PubMed]
- Scorsone K, Zhang L, Woodfield SE, et al. The novel kinase inhibitor EMD1214063 is effective against neuroblastoma. Invest New Drugs 2014;32:815-24. [Crossref] [PubMed]
- Yan B, Lim M, Zhou L, et al. Identification of MET genomic amplification, protein expression and alternative splice isoforms in neuroblastomas. J Clin Pathol 2013;66:985-91. [Crossref] [PubMed]
- Bao ZS, Chen HM, Yang MY, et al. RNA-seq of 272 gliomas revealed a novel, recurrent PTPRZ1-MET fusion transcript in secondary glioblastomas. Genome Res 2014;24:1765-73. [Crossref] [PubMed]
- Cancer Genome Atlas Research Network. Comprehensive genomic characterization defines human glioblastoma genes and core pathways. Nature 2008;455:1061-8. [Crossref] [PubMed]
- Navis AC, van Lith SA, van Duijnhoven SM, et al. Identification of a novel MET mutation in high-grade glioma resulting in an auto-active intracellular protein. Acta Neuropathol 2015;130:131-44. [Crossref] [PubMed]
- Schmidt L, Duh FM, Chen F, et al. Germline and somatic mutations in the tyrosine kinase domain of the MET proto-oncogene in papillary renal carcinomas. Nat Genet 1997;16:68-73. [Crossref] [PubMed]
- Albiges L, Guegan J, Le Formal A, et al. MET is a potential target across all papillary renal cell carcinomas: result from a large molecular study of pRCC with CGH array and matching gene expression array. Clin Cancer Res 2014;20:3411-21. [Crossref] [PubMed]
- Schmidt L, Junker K, Nakaigawa N, et al. Novel mutations of the MET proto-oncogene in papillary renal carcinomas. Oncogene 1999;18:2343-50. [Crossref] [PubMed]
- Cancer Genome Atlas Research Network, Linehan WM, Spellman PT, et al. Comprehensive Molecular Characterization of Papillary Renal-Cell Carcinoma. N Engl J Med 2016;374:135-45. [Crossref] [PubMed]
- Choueiri TK, Vaishampayan U, Rosenberg JE, et al. Phase II and biomarker study of the dual MET/VEGFR2 inhibitor foretinib in patients with papillary renal cell carcinoma. J Clin Oncol 2013;31:181-6. [Crossref] [PubMed]
- Xie Z, Lee YH, Boeke M, et al. MET Inhibition in Clear Cell Renal Cell Carcinoma. J Cancer 2016;7:1205-14. [Crossref] [PubMed]
- Choueiri TK, Escudier B, Powles T, et al. Cabozantinib versus Everolimus in Advanced Renal-Cell Carcinoma. N Engl J Med 2015;373:1814-23. [Crossref] [PubMed]
- Choueiri TK, Escudier B, Powles T, et al. Cabozantinib versus everolimus in advanced renal cell carcinoma (METEOR): final results from a randomised, open-label, phase 3 trial. Lancet Oncol 2016;17:917-27. [Crossref] [PubMed]
- Ariyawutyakorn W, Saichaemchan S, Varella-Garcia M. Understanding and Targeting MET Signaling in Solid Tumors - Are We There Yet? J Cancer 2016;7:633-49. [Crossref] [PubMed]
- Miyata Y, Asai A, Mitsunari K, et al. Met in urological cancers. Cancers (Basel) 2014;6:2387-403. [Crossref] [PubMed]
- Smith M, De Bono J, Sternberg C, et al. Phase III Study of Cabozantinib in Previously Treated Metastatic Castration-Resistant Prostate Cancer: COMET-1. J Clin Oncol 2016;34:3005-13. [Crossref] [PubMed]
- Beviglia L, Matsumoto K, Lin CS, et al. Expression of the c-Met/HGF receptor in human breast carcinoma: correlation with tumor progression. Int J Cancer 1997;74:301-9. [Crossref] [PubMed]
- Lengyel E, Prechtel D, Resau JH, et al. C-Met overexpression in node-positive breast cancer identifies patients with poor clinical outcome independent of Her2/neu. Int J Cancer 2005;113:678-82. [Crossref] [PubMed]
- Gastaldi S, Comoglio PM, Trusolino L. The Met oncogene and basal-like breast cancer: another culprit to watch out for? Breast Cancer Res 2010;12:208. [Crossref] [PubMed]
- Ho-Yen CM, Jones JL, Kermorgant S. The clinical and functional significance of c-Met in breast cancer: a review. Breast Cancer Res 2015;17:52. [Crossref] [PubMed]
- de Melo Gagliato D, Jardim DL, Falchook G, et al. Analysis of MET genetic aberrations in patients with breast cancer at MD Anderson Phase I unit. Clin Breast Cancer 2014;14:468-74. [Crossref] [PubMed]
- Lee JH, Han SU, Cho H, et al. A novel germ line juxtamembrane Met mutation in human gastric cancer. Oncogene 2000;19:4947-53. [Crossref] [PubMed]
- Sameni M, Tovar EA, Essenburg CJ, et al. Cabozantinib (XL184) Inhibits Growth and Invasion of Preclinical TNBC Models. Clin Cancer Res 2016;22:923-34. [Crossref] [PubMed]
- Diéras V, Campone M, Yardley DA, et al. Randomized, phase II, placebo-controlled trial of onartuzumab and/or bevacizumab in combination with weekly paclitaxel in patients with metastatic triple-negative breast cancer. Ann Oncol 2015;26:1904-10. [Crossref] [PubMed]
- Rayson D, Lupichuk S, Potvin K, et al. Canadian Cancer Trials Group IND197: a phase II study of foretinib in patients with estrogen receptor, progesterone receptor, and human epidermal growth factor receptor 2-negative recurrent or metastatic breast cancer. Breast Cancer Res Treat 2016;157:109-16. [Crossref] [PubMed]
- Di Renzo MF, Olivero M, Ferro S, et al. Overexpression of the c-MET/HGF receptor gene in human thyroid carcinomas. Oncogene 1992;7:2549-53. [PubMed]
- Huang Y, Prasad M, Lemon WJ, et al. Gene expression in papillary thyroid carcinoma reveals highly consistent profiles. Proc Natl Acad Sci U S A 2001;98:15044-9. [Crossref] [PubMed]
- Wasenius VM, Hemmer S, Kettunen E, et al. Hepatocyte growth factor receptor, matrix metalloproteinase-11, tissue inhibitor of metalloproteinase-1, and fibronectin are up-regulated in papillary thyroid carcinoma: a cDNA and tissue microarray study. Clin Cancer Res 2003;9:68-75. [PubMed]
- Wasenius VM, Hemmer S, Karjalainen-Lindsberg ML, et al. MET receptor tyrosine kinase sequence alterations in differentiated thyroid carcinoma. Am J Surg Pathol 2005;29:544-9. [Crossref] [PubMed]