Exosomes as delivery vehicles: a commentary on “Amoxicillin haptenates intracellular proteins that can be transported in exosomes to target cells”
Exosomes are lipoproteic nanosize vesicles of endocytic origin that are released in the extracellular space by several cell types when a multivesicular body fuses with the plasma membrane (1). To date, accumulating evidences highlight the potential of exosomes as messenger in intercellular communication. Indeed, several studies reported the presence of lipids, proteins, metabolites and nucleic acid (DNA, mRNA, microRNA, lncRNA) in exosomes and highlight their functional role in physiological and pathological processes (2).
These specialized nanovesicles behave as shuttles among cells; it is now largely accepted that exosomes influence tumor formation, progression as well as its dissemination by spreading cancer cell properties (3). In addition, exosomes are involved in several neurological diseases, by affecting neuronal communication (4), and are able to induce immunosuppressive or immune-activating effects on different steps of the immune response (5). Furthermore, accumulating evidences highlight the possibility that exosomes can modulate the response to therapy, inducing drug resistance, by exporting chemotherapeutic agents (6).
In addition to animal cells, exosome-like vesicles are released by plant (7) and bacteria (8). It has been observed that bacteria-derived extracellular vesicles have a role in the development of antibiotic resistance; indeed, Ciofu and colleagues showed that β-lactamase, the enzyme responsible for β-lactam resistance, is a constituent of P. aeruginosa derived membrane vesicles (9).
Recent studies provided evidences of the use of exosomes for therapeutical applications. Several groups demonstrated that, based on the molecules expressed on their surface, exosomes can be used as drug delivery systems to target specific cells. It has been shown, for example, that exosomes can deliver drugs or small RNA molecules, inhibiting cancer cells proliferation in vitro and in vivo animal models. Furthermore, exosomes have been considered in the development of vesicle-based vaccines in particular to induce an immune response against tumors or in some infectious diseases (10,11).
However, further studies are needed for the clinical translations of exosome-based therapeutics.
In addition, due to their content, that reflects that one of the cell of origin, exosomes represent a rich source of information. Exosome lipid bilayer confers stability to the vesicle content in biological fluids; thanks to the advances in exosome detection and purification, as well as to the sensitive technologies applied to the analysis of their content, exosomes are now considered as biomarker transporters in several pathological conditions.
Several drugs, and in particular β-lactams, have been shown to stimulate allergic reactions. Among the causes of the allergic response observed in β-lactams treated patients, there is the hapten hypothesis. Specifically, β-lactams are responsible for the formation of drug-protein adducts, a protein complex that is generated when electrophilic drugs or reactive drug metabolites covalently bind to protein molecules. Indeed, usually, drugs bind to plasma or tissue proteins in a reversible way, when this binding is covalent, an immune response may be induced.
β-lactams have high capacity to form covalent adducts with proteins through the opening of the β-lactam ring by the free amino groups of the protein; in particular, amoxicillin mainly forms adducts with albumin, transferrin, and with heavy and light chains of immunoglobulin.
Among β-lactams, amoxicillin is a wide spectrum antibiotic largely used for several bacterial infections and is among the drugs that most frequently induces allergic reactions (12).
To date haptenated proteins have been found in serum and several methods have been developed to detect and quantify them, aiming to a better understanding of drug toxicity. Identification approaches can be divided in global analysis and targeted analysis. The global approach is based on the use of radiolabeled drugs combined with 2D-gel electrophoresis and mass spectrometry in order to identify protein target of drug metabolites in in vitro experiments or in vivo animal models. A targeted method is a more sensitive approach and can be applied to patient samples; specifically it is based on the identification of a single protein target, giving specific information on how drugs modify the target, altering its function.
However, monitoring drug-protein adducts still remain one of the challenge in the field, and in particular for pharmaceutical industries. One of the major problems associated with adducts detection is its half-live and the in vivo concentration which is generally very low; indeed, around 1% of the protein is generally adducted. Therefore, efforts have been made for the development of more sensitive methods for haptenated proteins identification with a consequent improvement of the diagnostic procedures applied to avoid drug allergic reactions.
In the past, the most used approaches to identify β-lactam-protein adducts were based on immunological methods. Carey et al. reported that the treatment with flucloxacillin results in the formation of hepatic protein adducts (13), while Magi showed that ampicillin treatment induced the formation of adducts with albumin and transferrin (14).
Recent advances in proteomic techniques allowed to apply mass spectrometry analysis in order to study β-lactams adducts with human serum albumin (15).
In 2012 Ariza et al. used for the first time high resolution MS analyzer (orbitrap) coupled to nanoscale capillary liquid chromatography to an in-depth identification of amoxicillin-protein adducts. In addition to human serum albumin, authors identified other proteins as amoxicillin target; among these proteins, transferrin appeared highly modified by the drug (16).
More recently, the same research group developed a new sensitive tool for the study of protein haptenation by amoxicillin, by creating a biotinylated amoxicillin (AX-B) analog (17). Authors found that AX-B is able to form protein adducts similarly to the drug alone; in particular the two forms of the drug interacts with the same serum targets. By using the same approach, authors recently observed that amoxicillin binds to proteins present in exosome and that amoxicillin-protein adducts are transported by the vesicular carriers (18).
In their recent paper “Amoxicillin haptenates intracellular proteins that can be transported in exosomes to target cells” (18), Sánchez-Gómez and coworkers provided the first evidence of the presence of drug-adducts in circulating exosomes. In particular they showed that several intracellular proteins are haptenated by amoxicillin (AX) and released by cells in the soluble fractions as well as in exosomes.
Specifically, authors treated a lymphocytic B cell line with penicillin, amoxicillin and AX-B analog (17); they found that the treatment with the drugs induced the haptenation of several proteins that were present in the cell lysate as well as in the three fractions of the conditioned medium corresponding to membrane, extracellular vesicles and soluble proteins. These data suggested that modified proteins could be secreted from cells both in soluble and exosomal fractions. Mass spectrometry analysis allowed to identify proteins haptenated by amoxicillin; among these, authors found HSP70, EF-2, actin and α-enolase. In addition they found that haptenated proteins present in the exosome fraction could be delivered among cells and internalized by endothelial cells, contributing to the allergic response observed after amoxicillin treatment (18).
Overall, the presence of drug-protein adducts in exosomes could represent a new way to detect haptenated proteins in the circulation.
At the same time this study strongly highlights, as noted by the authors, a new mechanism for drug-protein adduct transfer among cells, thus providing the evidences of a vesicular sorting (Figure 1).
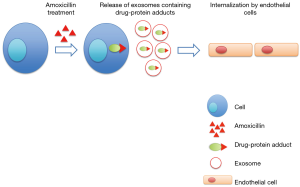
However, further studies are required in order to better understand the role of exosome-enclosed drug-protein adducts in contributing to the in vivo allergic responses observed in amoxicillin-treated patients.
Acknowledgements
The author kindly thanks Prof. Riccardo Alessandro (University of Palermo) for critical suggestions on the manuscript. Stefania Raimondo is supported by a Fondazione Italiana Ricerca sul Cancro (FIRC) fellowship.
Footnote
Conflicts of Interest: The author has no conflicts of interest to declare.
References
- Raposo G, Stoorvogel W. Extracellular vesicles: exosomes, microvesicles, and friends. J Cell Biol 2013;200:373-83. [Crossref] [PubMed]
- Corrado C, Raimondo S, Saieva L, et al. Exosome-mediated crosstalk between chronic myelogenous leukemia cells and human bone marrow stromal cells triggers an interleukin 8-dependent survival of leukemia cells. Cancer Lett 2014;348:71-6. [Crossref] [PubMed]
- Peinado H, Aleckovic M, Lavotshkin S, et al. Melanoma exosomes educate bone marrow progenitor cells toward a pro-metastatic phenotype through MET. Nat Med 2012;18:883-91. [Crossref] [PubMed]
- Alvarez-Erviti L, Seow Y, Schapira AH, et al. Lysosomal dysfunction increases exosome-mediated alpha-synuclein release and transmission. Neurobiol Dis 2011;42:360-7. [Crossref] [PubMed]
- Robbins PD, Morelli AE. Regulation of immune responses by extracellular vesicles. Nat Rev Immunol 2014;14:195-208. [Crossref] [PubMed]
- Safaei R, Larson BJ, Cheng TC, et al. Abnormal lysosomal trafficking and enhanced exosomal export of cisplatin in drug-resistant human ovarian carcinoma cells. Mol Cancer Ther 2005;4:1595-604. [Crossref] [PubMed]
- Raimondo S, Naselli F, Fontana S, et al. Citrus limon-derived nanovesicles inhibit cancer cell proliferation and suppress CML xenograft growth by inducing TRAIL-mediated cell death. Oncotarget 2015;6:19514-27. [Crossref] [PubMed]
- Kim JH, Lee J, Park J, et al. Gram-negative and Gram-positive bacterial extracellular vesicles. Semin Cell Dev Biol 2015;40:97-104. [Crossref] [PubMed]
- Ciofu O, Beveridge TJ, Kadurugamuwa J, et al. Chromosomal beta-lactamase is packaged into membrane vesicles and secreted from Pseudomonas aeruginosa. J Antimicrob Chemother 2000;45:9-13. [Crossref] [PubMed]
- Yao Y, Chen L, Wei W, et al. Tumor cell-derived exosome-targeted dendritic cells stimulate stronger CD8+ CTL responses and antitumor immunities. Biochem Biophys Res Commun 2013;436:60-5. [Crossref] [PubMed]
- Hosseini HM, Fooladi AA, Nourani MR, et al. Role of Exosome in infectious disease. Inflamm Allergy Drug Targets 2012. [Epub ahead of print]. [PubMed]
- Torres MJ, Blanca M. The complex clinical picture of beta-lactam hypersensitivity: penicillins, cephalosporins, monobactams, carbapenems, and clavams. Med Clin North Am 2010;94:805-20. xii. [Crossref] [PubMed]
- Carey MA, van Pelt FN. Immunochemical detection of flucloxacillin adduct formation in livers of treated rats. Toxicology 2005;216:41-8. [Crossref] [PubMed]
- Magi B, Marzocchi B, Bini L, et al. Two-dimensional electrophoresis of human serum proteins modified by ampicillin during therapeutic treatment. Electrophoresis 1995;16:1190-2. [Crossref] [PubMed]
- Whitaker P, Meng X, Lavergne SN, et al. Mass spectrometric characterization of circulating and functional antigens derived from piperacillin in patients with cystic fibrosis. J Immunol 2011;187:200-11. [Crossref] [PubMed]
- Ariza A, Garzon D, Abanades DR, et al. Protein haptenation by amoxicillin: high resolution mass spectrometry analysis and identification of target proteins in serum. J Proteomics 2012;77:504-20. [Crossref] [PubMed]
- Ariza A, Collado D, Vida Y, et al. Study of protein haptenation by amoxicillin through the use of a biotinylated antibiotic. PLoS One 2014;9:e90891. [Crossref] [PubMed]
- Sánchez-Gómez FJ, González-Morena JM, Vida Y, et al. Amoxicillin haptenates intracellular proteins that can be transported in exosomes to target cells. Allergy 2017;72:385-96. [PubMed]