Accurately measuring and modeling Th2 and Th17 endotypes in severe asthma
In our recently published report in Science Translational Medicine (1), we provided evidence of mutually exclusive regulation of Th2 and Th17 inflammation in bronchial biopsies obtained from asthmatic patients and explored these mechanisms in mouse models. Insightful commentaries on our report from Poynter and Lynch et al. raise a number of discussion points on our study and its relevance to ongoing efforts to develop new asthma therapies. Here, we appreciate the opportunity to continue the discussion, both by clarifying some of the data and our interpretation of key findings, as well as speculate on how our work might inform future studies and apply to the developing paradigm of asthmatic endotypes. Three areas of discussion we would like to address further are: (I) the relationship of granulocyte (eosinophil and neutrophil) populations to airway inflammation; (II) the use of noninvasive biomarkers in asthma; and (III) the temporal variability of inflammatory processes in vivo.
Many studies of asthmatic subtypes have assessed granulocyte populations in induced sputum as indicators of inflammatory activity. In their commentaries, Poynter and Lynch et al. raise several important questions about the significance of fluctuations in neutrophils and eosinophils and their use as indicators of disease severity. We have concerns about the dogma regarding the presence of an increased percentage of sputum neutrophils as a sole marker of asthma severity and of Th17 skewing. Given the broad range and relative abundance of neutrophils present in blood, tissue, and sputum of healthy patients, and the disconnect between sputum neutrophil percentages and other indicators of airway inflammation (2), interpreting changes in these cells with advancing disease or in the context of steroids or other treatment is complex.
For technical reasons, sputum analysis is most commonly expressed in terms of relative proportions of cells. Without a reference denominator, increased sputum neutrophil percentage could represent an increase in overall inflammation, an increase in IL-17 driven neutrophil recruitment, a change in neutrophil partitioning between tissue and lumen, or a relative reduction in other inflammatory cells such as eosinophils and macrophages. The lack of clear differences in bronchial tissue or blood neutrophil numbers between health and the spectrum of asthma severity (1,3-7), and the strong inverse correlation we have previously reported between sputum eosinophils and neutrophils in severe asthma despite a strong positive correlation in bronchoalveolar lavage (BAL) and bronchial tissue (2) highlights this complexity. To better resolve the nature of neutrophilic inflammation in asthma, future studies should address the obscurity of neutrophilic shifts by assessing the anatomic localization (e.g., intra-epithelial, lamina propria, or luminal), activation status, and functional phenotypes of airway-associated neutrophils in the context of their numbers and proportions. Indeed, a recent study found that neutrophils express unique transcriptional profiles and promote alternatively activated macrophage polarization when present during a type 2-polarized response (8). Thus, neutrophil activation state rather than number may be a more important indicator of their contribution to disease severity.
Likewise, Lynch et al. raised concerns over apparent increases in eosinophils in our chronic HDM mouse model when we simultaneously blocked IL-13 and IL-17. Further analysis of these studies indicate that while there is a slight increase in the proportion of eosinophils in the BAL fluid of mice treated with anti-IL-13 and anti-IL-17, due to overall decreases in BAL cellularity associated with reduced neutrophils and inflammation, the number of BAL eosinophils is the same or lower than in the IL-13 treatment alone group. In other models of type-2 inflammation, when IL-13 activity is blocked, we have routinely observed increases in eosinophils, potentially resulting from increased IL-4 activity leading to more IL-5 production. However, since eosinophils both respond to and produce IL-13, in the context of blocking IL-13 there is reduced disease activity as measured by other indices (e.g., airway hyperreactivity, mucus, and fibrosis) despite continued eosinophilia. These observations are similar to those of Manni et al. as discussed by Poynter, in which the authors found that blocking IL-13 was insufficient to reduce “total inflammation” in the lung despite reducing numerous indicators of disease such as mucus production and airway hyperreactivity. Neither we nor Manni et.al. assessed the activation status of eosinophils. A recent report has described distinct populations of “inflammatory” recruited eosinophils and “regulatory” tissue resident eosinophils that are less dependent on Th2 cytokines such as IL-5 (9). Furthermore, clinical studies of IL-13 inhibitors in asthma patients have reported increases in circulating eosinophils (10), which may represent decreased trafficking to inflamed tissues due to reductions in eosinophil chemoattractant expression. Whether the presence of a persistent inflammatory response has the potential to complicate therapy over time requires further study, and mechanistic studies to assess the effects of IL-13 inhibition on airway inflammation in asthma patients are under way (11,12).
Taken together, these points highlight the somewhat simplistic nature of current reliance on granulocyte proportions in sputum as a determinant of asthma severity and disease progression. In the context of evaluating disease severity and response to therapy, the assessment of neutrophil and eosinophil proportions should be supplemented with a more thorough quantification of their absolute number, anatomic localization, activation states, and production of cytokines and other inflammatory mediators that constitute their functional contribution to disease. Cell specific deletion of important eosinophil and neutrophil-associated genes could help with these investigations.
A promising opportunity to improve our understanding of the asthmatic airway beyond cellular determinants brought up by both commentaries is the development of non-invasive biomarkers to define patient endotypes. It may be similarly important to understand the stability of these endotypes over time (13). Lynch et al. suggest that measurements of sputum cytokines may be informative; however, the use of reducing agents for mucolytic in sputum processing differentially affects the recovery of cytokines with available assays and may confound interpretation. Our use of cytokine-responsive gene signatures associated with each pathway has the advantage of identifying the net signal that cells are responding to in a complex environment. In our study, these signatures clearly demonstrate that, at a given point in time, stromal tissues in the asthmatic lung predominantly respond to one pathway or the other, or neither, but not both. We have also shown this negative regulatory correlation between the Th2 and Th17 pathways in bronchial epithelial cells in vitro in our paper, and Lynch et al. correctly point out that many cell types likely contribute to these expression patterns in vivo. In fact, two of our laboratories have demonstrated one potential mechanism of cross regulation between IL-13 and IL-17A responses via upregulation of IL-13Rα2, a decoy receptor for IL-13, by IL-17 and TNFα in human fibroblast populations (14,15). This mechanism reduces IL-13 effector function and may apply to other immune and parenchymal cells in the asthmatic lung. This transcriptional cross-regulation is clear despite documented instances in our studies and others of simultaneous production of IL-13 and IL-17 and the presence of mixed type 17 and type 2 inflammatory cells (16,17).
Biomarkers associated with both pathways may change on a slower timeframe than gene transcription. For example, temporal differences in response may explain why the Th2 signature, at a given point in time, is strongly associated with FeNO and sputum eosinophils, whereas both the Th17 and Th2 signatures are associated with serum periostin, blood eosinophils, and tissue eosinophils. Nitric oxide is produced by the action of iNOS encoded by the NOS2 gene, and eosinophils are mobilized by chemokines such as eotaxin-3 encoded by the CCL26 gene, both of which are inducible by IL-13 and highly correlated with the Th2 signature (18). The half life of blood eosinophils in humans is several days (19) and it is likely that serum periostin levels may fluctuate on a similar time scale (20), while serial bronchoscopy studies with anti-IL5 suggest that bronchial tissue, but not sputum eosinophils, may persist for weeks to months (21). Thus, FeNO and sputum eosinophils may reflect acute activity of type 2 cytokines in the airways whereas tissue eosinophils and blood biomarkers may reflect aggregate type 2 airway inflammation over longer time intervals. Because our bronchoscopy study was cross-sectional, we only captured a “snapshot” of airway inflammation. Taken together, these results suggest that environmental exposures may acutely influence the inflammatory milieu in the airways and longer-lived biomarkers may more accurately reflect the complex inflammatory states of a given asthma patient (Figure 1). To enable better long-term treatment decisions for asthma patients, future studies should aim to identify biomarkers that integrate activity of these pathways over time, akin to hemoglobin A1C levels in diabetes patients (22). Analyses of both immediate and longitudinal gene expression and inflammatory profiles in the lung should provide a more comprehensive picture of the interplay between these pathways over time, and our data suggest that subtle shifts in environmental exposures may induce switching between inflammatory pathways.
Each commentary raises important points about these inflammatory shifts and the potential emergence of compensatory inflammatory pathways, particularly regarding how these may be best replicated in animal models. In addition to various allergens, the lungs of patients are exposed to a multitude of stimuli including bacteria, virus, fungus, pollutants, and other irritants. The genetic predisposition of each individual and pattern of exposure to each of the irritants likely dictate the dominant character of the inflammatory response at any given point in time. Mouse models of asthma should also take these findings into consideration. While the Manni et al. paper discussed by Poynter complements our studies, it did not describe a neutrophilic response in the context of IL-13 blockade, which may be due to the brief time frame of their model, differences in epithelial activation or damage, and induction of inflammatory alarmins. In agreement with Lynch et al. we look forward to the potential of IL-33 targeting therapeutics, though recent work we published using the chronic HDM mouse model suggests that blocking individual alarmins might have limited efficacy when disease is well established (23). These points suggest that modeling the diversity of asthmatic endotypes might require comparison of numerous chronic mouse models with a wide range of inflammatory stimuli. Indeed, recent reports have used a range of stimuli to induce features of asthma in chronic mouse models covering a range of inflammatory profiles (24,25).
Finally, authors from both commentaries bring up the implications of our studies to ongoing and future clinical trials. Limited or failed responses to single Th2 or Th17 blocking agents (26,27) could have resulted from the inclusion of patients with the wrong type of active disease and/or emergence of compensatory inflammatory pathways. Thus, we believe that correct identification of patients that will respond to targeted therapies is critical and close monitoring of treated patients for the activation of compensatory inflammatory pathways is warranted. Furthermore, therapies targeting both Th2 and Th17 pathways simultaneously may be indicated for patients with poor asthma control on corticosteroids.
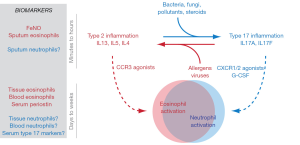
Acknowledgements
None.
Footnote
Conflicts of Interest: DFC and JRA are employees of Genentech, Inc., hold stock and/or stock options in the Roche Group, and are named inventors on patents pending related to asthma biomarkers and treatments. The other authors have no conflicts of interest to declare.
References
- Choy DF, Hart KM, Borthwick LA, et al. TH2 and TH17 inflammatory pathways are reciprocally regulated in asthma. Sci Transl Med 2015;7:301ra129. [Crossref] [PubMed]
- Arron JR, Choy DF, Laviolette M, et al. Disconnect between sputum neutrophils and other measures of airway inflammation in asthma. Eur Respir J 2014;43:627-9. [Crossref] [PubMed]
- Shikotra A, Choy DF, Ohri CM, et al. Increased expression of immunoreactive thymic stromal lymphopoietin in patients with severe asthma. J Allergy Clin Immunol 2012;129:104-11.e1-9.
- Vrugt B, Wilson S, Underwood J, et al. Mucosal inflammation in severe glucocorticoid-dependent asthma. Eur Respir J 1999;13:1245-52. [Crossref] [PubMed]
- Synek M, Beasley R, Frew AJ, et al. Cellular infiltration of the airways in asthma of varying severity. Am J Respir Crit Care Med 1996;154:224-30. [Crossref] [PubMed]
- Siddiqui S, Mistry V, Doe C, et al. Airway hyperresponsiveness is dissociated from airway wall structural remodeling. J Allergy Clin Immunol 2008;122:335-41, 341.e1-3.
- Bradley BL, Azzawi M, Jacobson M, et al. Eosinophils, T-lymphocytes, mast cells, neutrophils, and macrophages in bronchial biopsy specimens from atopic subjects with asthma: comparison with biopsy specimens from atopic subjects without asthma and normal control subjects and relationship to bronchial hyperresponsiveness. J Allergy Clin Immunol 1991;88:661-74. [Crossref] [PubMed]
- Chen F, Wu W, Millman A, et al. Neutrophils prime a long-lived effector macrophage phenotype that mediates accelerated helminth expulsion. Nat Immunol 2014;15:938-46. [Crossref] [PubMed]
- Mesnil C, Raulier S, Paulissen G, et al. Lung-resident eosinophils represent a distinct regulatory eosinophil subset. J Clin Invest 2016;126:3279-95. [Crossref] [PubMed]
- Staton TL, Choy DF, Arron JR. Biomarkers in the clinical development of asthma therapies. Biomark Med 2016;10:165-76. [Crossref] [PubMed]
- Roche HL. A Study Evaluating the Effects of Lebrikizumab on Airway Eosinophilic Inflammation in Participants With Uncontrolled Asthma. Available online: https://clinicaltrials.gov/ct2/show/NCT02099656
- Sanofi. Evaluation of Dupilumab's Effects on Airway Inflammation in Patients With Asthma (EXPEDITION). Available online: https://clinicaltrials.gov/ct2/show/NCT02573233
- McGrath KW, Icitovic N, Boushey HA, et al. A large subgroup of mild-to-moderate asthma is persistently noneosinophilic. Am J Respir Crit Care Med 2012;185:612-9. [Crossref] [PubMed]
- Chandriani S, DePianto DJ, N'Diaye EN, et al. Endogenously expressed IL-13Rα2 attenuates IL-13-mediated responses but does not activate signaling in human lung fibroblasts. J Immunol 2014;193:111-9. [Crossref] [PubMed]
- Badalyan V, Thompson R, Addo K, et al. TNF-α/IL-17 synergy inhibits IL-13 bioactivity via IL-13Rα2 induction. J Allergy Clin Immunol 2014;134:975-8.e5. [Crossref] [PubMed]
- Irvin C, Zafar I, Good J, et al. Increased frequency of dual-positive TH2/TH17 cells in bronchoalveolar lavage fluid characterizes a population of patients with severe asthma. J Allergy Clin Immunol 2014;134:1175-1186.e7. [Crossref] [PubMed]
- Liu W, Liu S, Verma M, et al. Mechanism of TH2/TH17-predominant and neutrophilic TH2/TH17-low subtypes of asthma. J Allergy Clin Immunol 2016. [Epub ahead of print]. [PubMed]
- Arron JR, Choy DF, Scheerens H, et al. Noninvasive biomarkers that predict treatment benefit from biologic therapies in asthma. Ann Am Thorac Soc 2013;10 Suppl:S206-13. [Crossref] [PubMed]
- Smith DA, Minthorn EA, Beerahee M. Pharmacokinetics and pharmacodynamics of mepolizumab, an anti-interleukin-5 monoclonal antibody. Clin Pharmacokinet 2011;50:215-27. [Crossref] [PubMed]
- Fingleton J, Braithwaite I, Travers J, et al. Serum periostin in obstructive airways disease. Eur Respir J 2016;47:1383-91. [Crossref] [PubMed]
- Haldar P, Brightling CE, Hargadon B, et al. Mepolizumab and exacerbations of refractory eosinophilic asthma. N Engl J Med 2009;360:973-84. [Crossref] [PubMed]
- Radin MS. Pitfalls in hemoglobin A1c measurement: when results may be misleading. J Gen Intern Med 2014;29:388-94. [Crossref] [PubMed]
- Vannella KM, Ramalingam TR, Borthwick LA, et al. Combinatorial targeting of TSLP, IL-25, and IL-33 in type 2 cytokine-driven inflammation and fibrosis. Sci Transl Med 2016;8:337ra65. [Crossref] [PubMed]
- Raundhal M, Morse C, Khare A, et al. High IFN-γ and low SLPI mark severe asthma in mice and humans. J Clin Invest 2015;125:3037-50. [Crossref] [PubMed]
- Christianson CA, Goplen NP, Zafar I, et al. Persistence of asthma requires multiple feedback circuits involving type 2 innate lymphoid cells and IL-33. J Allergy Clin Immunol 2015;136:59-68.e14. [Crossref] [PubMed]
- Hanania NA, Korenblat P, Chapman KR, et al. Efficacy and safety of lebrikizumab in patients with uncontrolled asthma (LAVOLTA I and LAVOLTA II): replicate, phase 3, randomised, double-blind, placebo-controlled trials. Lancet Respir Med 2016;4:781-96. [Crossref] [PubMed]
- Busse WW, Holgate S, Kerwin E, et al. Randomized, double-blind, placebo-controlled study of brodalumab, a human anti-IL-17 receptor monoclonal antibody, in moderate to severe asthma. Am J Respir Crit Care Med 2013;188:1294-302. [Crossref] [PubMed]