The chicken or the egg dilemma: intestinal dysbiosis in multiple sclerosis
The microbiota is the complete population of microbes that include species from the three domains that include cellular microbes, Bacteria, Archaea, Eukarya, and acellular infectious organisms such as viruses, that inhabit a biogeographical location (1). In the intestine of experimental animal models, the microbiota plays a significant role shaping the immune system, regulating the host’s metabolism, providing a biological defense mechanism against infections, as well as modulating the appropriate neuronal development. Despite the experimental evidences obtained using gnotobiotic animals, treating mice with antibiotics or by performing monocolonization studies, the direct link between the microbiota and human diseases has not been elucidated.
Recent advances in DNA sequencing and management of large amount of data implemented have allowed investigators to compare the composition of the intestinal microbiota in the context of human diseases. Experimental evidence suggests that changes in the composition of the heterogeneous intestinal ecosystem may be associated with alterations in the severity in different models of human autoimmune diseases such as inflammatory bowel disease (2), asthma (3), allergies (4), diabetes (5), rheumatoid arthritis (6), or systemic lupus erythematosus (SLE) (7). Furthermore, results obtained in the multiple sclerosis (MS) animal model, experimental autoimmune encephalomyelitis (EAE), suggest an association between the gut and the central nervous system (CNS) inflammatory demyelination (8). The gut-brain connection is currently being experimentally explored in the context of different human CNS disorders, such as autism (9,10), and Parkinson’s Disease (PD) (11).
The alteration of the intestinal microbial community that might lead to either animal or human diseases is termed intestinal or gut dysbiosis. The number of studies comparing the composition of the intestinal microbiota of MS patients with healthy individuals is gradually increasing over the recent years (Table 1). Many of the studies shown in Table 1 have been recently reviewed by Newland et al. (19). The studies conducted so far suggest the presence of a disrupted or altered microbiota in MS patients when compared to healthy controls (12-18). All studies were performed in patients diagnosed with relapsing remitting MS, and in some the effects of immunomodulatory therapies were evaluated (13,18). Overall, the analysis suggest that significant differences in specific genera or even species are found, including elevated relative abundances in Methanobrevibacter and Akkermansia, as well as reduced Butyricimonas found in the stool samples of MS patients (18). A recent study showed that the overall structure of the MS microbiota is significantly different to healthy controls and interestingly also significantly different between active and remission MS (17). Genera Adlercreutzia, Collinsella, Parabacteroides, Erysipelotrichaceae, Lachnospiraceae, Veillonellaceae, Lactobacillus, Coprobacillus, and Haemophilus relative abundances were reduced in the intestines of MS when compared to healthy controls, whereas genera Pedobacter, Flavobacterium, Blautia, Dorea, Pseudomonas and Mycoplana relative abundances were elevated. Other significant observations have been reported, such as significant reductions in specific clusters of Clostridium and species of Bacteroides (14,15). The changes in the abundances of these microbes could have direct effects on the immune system. As it will be discussed later, we suggest that the interaction between the microbiota and disease is multifactorial and bidirectional. The complex array of interactions is described in Figure 1. Both Clostridium and Bacteroides species present in the colon are known to produce short chain fatty acids (SCFA) as a product of non-digestible carbohydrates fermentation. SCFAs regulate immune cells gene expression, promotion of a regulatory phenotype, decrease of oxidative stress, and reinforce the intestinal barrier and blood-brain barrier (BBB) (20,21). Bacteroides fragilis, an inhabitant of the human gut, is known to induce strong immunomodulatory effects via the expression of the capsular polysaccharide A (PSA) (22-25). Our laboratory has previously shown that oral treatment with PSA protects mice against EAE through the induction of regulatory CD39+ T cells and Foxp3+ T regulatory cells (Tregs) (24,26,27). B. fragilis colonization also improves the integrity of the barrier (9). The complete absence of microbes in gnotobiotic mice C57BL/6 mice subjected to the induction of active EAE (28) and in gnotobiotic transgenic mice (29) that develop the disease spontaneously significantly affects the development of disease. Furthermore, treatment with oral antibiotics also reduce significantly the severity of EAE (30,31). The experimental evidence gathered using animal model studies indicate the effects of the microbiota in the regulation of EAE in animals (24,26-28,32-36). Furthermore, gut microbes, such as B. fragilis and Lactobacillus rhamnosus have been shown to interact with neurons in the enteric nervous system (ENS) directly and affect their function (37). The gut microbiota can directly influence hormonal levels, thus modulating the effects of the endocrine system in the autonomous nervous system (38). For example, the effects of the microbiota on MS maybe associated with direct interactions and functional changes on the immune, endocrine and neuronal systems (Figure 1a), as previously reviewed (39-41).
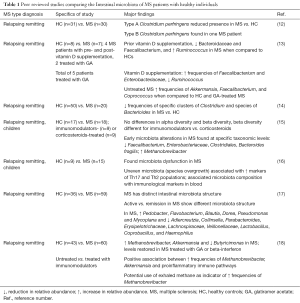
Full table
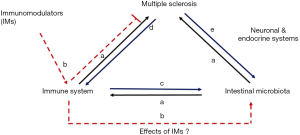
In a recent work published by Jangi and collaborators, the gut microbiota of 60 MS patients was compared with the microbiota of 43 healthy donors (18). Both male and female relapsing-remitting MS patients were selected for the study. A cohort of patients that received beta-interferon or glatiramer acetate for at least half-year were also included in the study, although individuals under treatment with immunosuppressive drugs as well as individuals suffering from other autoimmune diseases were excluded. Microbial 16S ribosomal RNA profiles were obtained from stool samples using two distinct sequencing platforms. The comparison of the intestinal microbial profile showed no significant differences in the diversity of the microbial populations of all samples analyzed. The overall structure of the microbiota determined by all sequences of both healthy and MS populations were found to be not significantly different. Of note, we have obtained preliminary data in EAE mice that indicates no significant changes in alpha diversity and time-dependent changes in the overall composition of the intestinal microbiota (42).
At the genus level the analysis showed an increased relative abundance of the archaeal genus Methanobrevibacter and bacterial genus Akkermansia and reduced relative abundance of Butyricimonas in the stool samples of MS patients, either untreated or treated with interferon-beta or glatiramer acetate and untreated when compared to healthy individuals. Reduced abundances of Prevotella and Sutterella were also reported in samples obtained from untreated MS patients when compared with healthy controls. Interestingly, the treatment with these two immunomodulatory therapies increased the relative abundances of genera, such as Prevotella and Sutterella, that were shown to be reduced in untreated MS patients versus treated. No significant differences were observed between those treated with either interferon-beta or glatiramer acetate, possibly due to the reduced size sample available for the comparison, as argued by the authors. However, despite the potential differences in the mechanism of action between these two drugs, the analysis performed suggests that immunomodulatory drugs might have the capacity to alter the composition of the microbiota, and reverse the apparent dysbiosis that was observed in those with relapsing MS (Figure 1b).
Teriflunomide, a more recently FDA approved treatment for relapsing MS may also have an impact on the dysbiosis in those with MS. We have recently shown that the oral treatment of non-EAE C57BL/6 mice with teriflunomide promotes the acquisition of a CD39 phenotype on gut-associated lymphoid tissues (GALT) T cells with enhanced protective ability when transferred into EAE mice (43). The TERI-DYNAMIC study on the immunomodulatory effects of teriflunomide on relapsing-remitting patients recently reported an increased frequency of circulating inducible Tregs in treated patients when compared with controls (44). Together, the human and murine immune studies suggest that teriflunomide may have immunomodulatory effects that could potentially affect gut dysbiosis.
In their study, Jangi and colleagues demonstrate an association between the changes in the intestinal microbiota and the peripheral immunological responses observed in relapsing remitting MS patients (Figure 1a). The gene expression in circulating T cells and in monocytes of patients and controls was used to define an immune-related gene profile. The gene expression analysis was performed after screening patients based on the intestinal relative abundances of Methanobrevibacter, increased in samples obtained from MS patients when compared to healthy individuals. Correlation studies demonstrated a positive association between increased intestinal relative abundances of Methanobrevibacter and Akkermansia and the upregulation of genes of T cells related to proinflammatory pathways of interferon and IL-2 signaling, as well as activation of peroxisome proliferator-activated receptor (PPAR) and LXR/RXR. Similar comparison was performed in monocytes isolated from blood samples of MS patients. When the expression genes of monocytes were compared, a positive correlation was observed between the presence of increased relative abundances of Methanobrevibacter and Akkermansia, and proliferative pathways including interferon signaling, dendritic cell maturation and TREM signaling. Conversely, the increased relative abundances of Butyricimonas, a genus that belong to the phylum Bacteroidetes, showed a negative correlation with the pathways described for both T cells and monocytes. The results reported are of great interest since they might define an immunological effect of the dysbiotic state of the intestinal microbiota in those with relapsing MS. Thus, these results obtained might suggest that specific changes on relative abundances could drive opposite immunological responses that could regulate disease progression and severity (Figure 1a). Valuable therapeutic information might be culled from similar studies conducted in MS patients under treatment with other immunomodulators (Figure 1b). Of great relevance would be to discern whether there are differences in the microbiota and immune pathways of MS patients that respond or not to therapy.
The search for novel MS biomarkers has been exhaustively explored with little success. When considering intestinal dysbiosis, it would be of great interest to utilize an inexpensive, noninvasive and cheap parameter to determine potentially pathogenic changes in the gut microbiota. Based on the intestinal abundances of Methanobrevibacter smithii, exhaled methane was compared in patients and healthy individuals. Archaeal microbes are classified based on metabolic and environmental adaptations that define them as extremophiles. In the gut, Methanobrevibacter use end-products of the sugars fermentation by other microbiota members for their own benefit, producing methane. Using gas chromatography, Jangi et al. detected enhanced amounts of methane exhaled by MS patients. Although preliminary, expanded studies would determine whether the detection of exhaled methane could potentially be considered a marker for MS-associated intestinal dysbiosis.
One important question that remains to be answered is whether disease can affect the intestinal ecosystem and the gut microbiota, as hypothesized in Figure 1 (Figure 1c,d). The induction of experimental CNS inflammatory demyelination increases intestinal permeability in mice as early as 7 days after active induction of the disease, with an associated increase in the frequencies of Th1, Th17, and IL-17+ gamma-delta (γδT) cells in the GALT of EAE mice (45). The intestinal permeability increases when EAE is induced. Of note, there is a similar pattern in mice that were adoptively transferred with autoreactive CD4+ T cells, a widely used protocol for the passive induction of EAE. The adoptive transfer findings are important due to the absence of adjuvants used for passive EAE. It could be postulated that the inclusion of adjuvants to induce disease can perhaps be associated with the increased intestinal permeability observed in mice developing EAE. An increase in the intestinal permeability was also observed in a murine model of autism spectrum disorders (ASD) that exhibit GI dysfunction and intestinal dysbiosis. These effects can be reversed with the oral treatment with B. fragilis (9). In germ-free mice gut epithelial integrity is also affected and is restored by colonization with a probiotic therapy consisted in Lactobacillus rhamnosus LOCK0900, L. rhamnosus LOCK0908 and L. casei LOCK0919, improving the intestinal morphology and barrier integrity (46). Experimental findings suggest a change in the intestinal permeability that occurs even before disease onset may imply that the disease itself affects the integrity of the intestinal interaction between microbes and our cells. In humans, a recent pilot study showed that the average levels of mannitol in urine were increased in 22 relapsing-remitting MS patients when compared to 18 healthy individuals (47). Authors hypothesized that the observation was the result of an increased intestinal permeability associated with MS. However, whether intestinal barrier disruption precedes or not disease is a question that remains to be answered.
The studies performed using human stool samples obtained from relapsing MS patients suggest a dysbiotic gut (Table 1). Two of the studies that compared the gut microbiota of MS patients associated the changes in the relative abundances of intestinal microbes with phenotypic modifications in the immune homeostasis and balance between pro- and anti-inflammatory or regulatory cell populations in blood (15) and genotypic changes (18). These studies advance our understanding on the interaction between the gut microbiota and the immune system. This interaction may be in one direction, by microbes regulating the type and function of the responsive immune system and immune cells controlling immunopathology of MS (Figure 1a). However, could the MS-specific immune status (Figure 1c) and disease-modifying immunomodulatory therapies also affect the composition of the intestinal microbiota (Figure 1e)? Jangi and colleagues discussed potential limitations in their study, including the effects that MS-physiological changes might have on the composition of the microbiota. The effects of EAE induction on the intestinal barrier disruption as well as on the intestinal microbiota raise the question whether on the animal models of MS, dysbiosis is cause or effect.
In summary, the experimental observations obtained from MS patients suggest that intestinal dysbiosis occurs during the course of the disease (Figure 1c-e). The association made by Jangi and collaborators between patients with elevated relative abundances of the archaeal methanogen Methanobrevibacter and increased expression of genes related to immunopathologic pathways in MS is intriguing and constitutes a step forward on the understanding of the gut/disease axis. More and larger efforts are now necessary to discern whether the differences observed in the gut microbiome are translated into a functionally biological relevance. Whether dysbiosis precedes MS (Figure 1a) or if it is the disease that affects the composition and function of the microbiota (Figure 1c-e), is a question that remains to be answered. Future directions might have a significant impact on MS patients since these will likely determine whether the effects that are now observed in the gut microbial populations and function can be used as potential target for novel therapies against the disease.
Acknowledgements
Funding: Studies referenced in the manuscript were funded by the National MS Society (NMSS) (award #PP3423) and Sanofi Genzyme Corporation (Genzyme/IST Concept Award GF-2014-11153).
Footnote
Conflicts of Interest: J Ochoa-Repáraz received funding from the National Multiple Sclerosis; K Magori reports no disclosures; LH Kasper received honoraria from a speakers bureau.
References
- Ley RE, Peterson DA, Gordon JI. Ecological and evolutionary forces shaping microbial diversity in the human intestine. Cell 2006;124:837-48. [Crossref] [PubMed]
- Prosberg M, Bendtsen F, Vind I, et al. The association between the gut microbiota and the inflammatory bowel disease activity: a systematic review and meta-analysis. Scand J Gastroenterol 2016;51:1407-15. [Crossref] [PubMed]
- Hauptmann M, Schaible UE. Linking microbiota and respiratory disease. FEBS Lett 2016;590:3721-38. [Crossref] [PubMed]
- Blázquez AB, Berin MC. Microbiome and food allergy. Transl Res 2017;179:199-203. [Crossref] [PubMed]
- Needell JC, Zipris D. The Role of the Intestinal Microbiome in Type 1 Diabetes Pathogenesis. Curr Diab Rep 2016;16:89. [Crossref] [PubMed]
- Coit P, Sawalha AH. The human microbiome in rheumatic autoimmune diseases: A comprehensive review. Clin Immunol 2016;170:70-9. [Crossref] [PubMed]
- Rosser EC, Mauri C. A clinical update on the significance of the gut microbiota in systemic autoimmunity. J Autoimmun 2016;74:85-93. [Crossref] [PubMed]
- Mielcarz DW, Kasper LH. The gut microbiome in multiple sclerosis. Curr Treat Options Neurol 2015;17:344. [Crossref] [PubMed]
- Hsiao EY, McBride SW, Hsien S, et al. Microbiota modulate behavioral and physiological abnormalities associated with neurodevelopmental disorders. Cell 2013;155:1451-63. [Crossref] [PubMed]
- Hsiao EY, McBride SW, Chow J, et al. Modeling an autism risk factor in mice leads to permanent immune dysregulation. Proc Natl Acad Sci U S A 2012;109:12776-81. [Crossref] [PubMed]
- Sampson TR, Debelius JW, Thron T, et al. Gut Microbiota Regulate Motor Deficits and Neuroinflammation in a Model of Parkinson's Disease. Cell 2016;167:1469-80.e12. [Crossref] [PubMed]
- Rumah KR, Linden J, Fischetti VA, et al. Isolation of Clostridium perfringens type B in an individual at first clinical presentation of multiple sclerosis provides clues for environmental triggers of the disease. PLoS One 2013;8:e76359. [Crossref] [PubMed]
- Cantarel BL, Waubant E, Chehoud C, et al. Gut microbiota in multiple sclerosis: possible influence of immunomodulators. J Investig Med 2015;63:729-34. [Crossref] [PubMed]
- Miyake S, Kim S, Suda W, et al. Dysbiosis in the Gut Microbiota of Patients with Multiple Sclerosis, with a Striking Depletion of Species Belonging to Clostridia XIVa and IV Clusters. PLoS One 2015;10:e0137429. [Crossref] [PubMed]
- Tremlett H, Fadrosh DW, Faruqi AA, et al. Associations between the gut microbiota and host immune markers in pediatric multiple sclerosis and controls. BMC Neurol 2016;16:182. [Crossref] [PubMed]
- Tremlett H, Fadrosh DW, Faruqi AA, et al. Gut microbiota in early pediatric multiple sclerosis: a case-control study. Eur J Neurol 2016;23:1308-21. [Crossref] [PubMed]
- Chen J, Chia N, Kalari KR, et al. Multiple sclerosis patients have a distinct gut microbiota compared to healthy controls. Sci Rep 2016;6:28484. [Crossref] [PubMed]
- Jangi S, Gandhi R, Cox LM, et al. Alterations of the human gut microbiome in multiple sclerosis. Nat Commun 2016;7:12015. [Crossref] [PubMed]
- Newland PK, Heitkemper M, Zhou Y. The Emerging Role of the Gut Microbiome in Adult Patients With Multiple Sclerosis. J Neurosci Nurs 2016;48:358-64. [Crossref] [PubMed]
- Wang H, Shi P, Zuo L, et al. Dietary Non-digestible Polysaccharides Ameliorate Intestinal Epithelial Barrier Dysfunction in IL-10 Knockout Mice. J Crohns Colitis 2016;10:1076-86. [Crossref] [PubMed]
- Braniste V, Al-Asmakh M, Kowal C, et al. The gut microbiota influences blood-brain barrier permeability in mice. Sci Transl Med 2014;6:263ra158. [Crossref] [PubMed]
- Wang Q, McLoughlin RM, Cobb BA, et al. A bacterial carbohydrate links innate and adaptive responses through Toll-like receptor 2. J Exp Med 2006;203:2853-63. [Crossref] [PubMed]
- Mazmanian SK, Liu CH, Tzianabos AO, et al. An immunomodulatory molecule of symbiotic bacteria directs maturation of the host immune system. Cell 2005;122:107-18. [Crossref] [PubMed]
- Ochoa-Repáraz J, Mielcarz DW, Wang Y, et al. A polysaccharide from the human commensal Bacteroides fragilis protects against CNS demyelinating disease. Mucosal Immunol 2010;3:487-95. [Crossref] [PubMed]
- Round JL, Mazmanian SK. Inducible Foxp3+ regulatory T-cell development by a commensal bacterium of the intestinal microbiota. Proc Natl Acad Sci U S A 2010;107:12204-9. [Crossref] [PubMed]
- Wang Y, Telesford KM, Ochoa-Repáraz J, et al. An intestinal commensal symbiosis factor controls neuroinflammation via TLR2-mediated CD39 signalling. Nat Commun 2014;5:4432. [PubMed]
- Wang Y, Begum-Haque S, Telesford KM, et al. A commensal bacterial product elicits and modulates migratory capacity of CD39(+) CD4 T regulatory subsets in the suppression of neuroinflammation. Gut Microbes 2014;5:552-61. [Crossref] [PubMed]
- Lee YK, Menezes JS, Umesaki Y, et al. Proinflammatory T-cell responses to gut microbiota promote experimental autoimmune encephalomyelitis. Proc Natl Acad Sci U S A 2011;108 Suppl 1:4615-22. [Crossref] [PubMed]
- Berer K, Mues M, Koutrolos M, et al. Commensal microbiota and myelin autoantigen cooperate to trigger autoimmune demyelination. Nature 2011;479:538-41. [Crossref] [PubMed]
- Yokote H, Miyake S, Croxford JL, et al. NKT cell-dependent amelioration of a mouse model of multiple sclerosis by altering gut flora. Am J Pathol 2008;173:1714-23. [Crossref] [PubMed]
- Ochoa-Repáraz J, Mielcarz DW, Ditrio LE, et al. Role of gut commensal microflora in the development of experimental autoimmune encephalomyelitis. J Immunol 2009;183:6041-50. [Crossref] [PubMed]
- Ochoa-Repáraz J, Mielcarz DW, Ditrio LE, et al. Central nervous system demyelinating disease protection by the human commensal Bacteroides fragilis depends on polysaccharide A expression. J Immunol 2010;185:4101-8. [Crossref] [PubMed]
- Lavasani S, Dzhambazov B, Nouri M, et al. A novel probiotic mixture exerts a therapeutic effect on experimental autoimmune encephalomyelitis mediated by IL-10 producing regulatory T cells. PLoS One 2010;5:e9009. [Crossref] [PubMed]
- Ezendam J, de Klerk A, Gremmer ER, et al. Effects of Bifidobacterium animalis administered during lactation on allergic and autoimmune responses in rodents. Clin Exp Immunol 2008;154:424-31. [Crossref] [PubMed]
- Takata K, Kinoshita M, Okuno T, et al. The lactic acid bacterium Pediococcus acidilactici suppresses autoimmune encephalomyelitis by inducing IL-10-producing regulatory T cells. PLoS One 2011;6:e27644. [Crossref] [PubMed]
- Rezende RM, Oliveira RP, Medeiros SR, et al. Hsp65-producing Lactococcus lactis prevents experimental autoimmune encephalomyelitis in mice by inducing CD4+LAP+ regulatory T cells. J Autoimmun 2013;40:45-57. [Crossref] [PubMed]
- Mao YK, Kasper DL, Wang B, et al. Bacteroides fragilis polysaccharide A is necessary and sufficient for acute activation of intestinal sensory neurons. Nat Commun 2013;4:1465. [Crossref] [PubMed]
- Yano JM, Yu K, Donaldson GP, et al. Indigenous bacteria from the gut microbiota regulate host serotonin biosynthesis. Cell 2015;161:264-76. [Crossref] [PubMed]
- Wang Y, Kasper LH. The role of microbiome in central nervous system disorders. Brain Behav Immun 2014;38:1-12. [Crossref] [PubMed]
- Mayer EA. Gut feelings: the emerging biology of gut-brain communication. Nat Rev Neurosci 2011;12:453-66. [Crossref] [PubMed]
- Mayer EA, Knight R, Mazmanian SK, et al. Gut microbes and the brain: paradigm shift in neuroscience. J Neurosci 2014;34:15490-6. [Crossref] [PubMed]
- Ochoa-Reparaz J, Colpitts S, Kasper E, et al. The Gut Microbiome Affects the Progression of Disease in a Murine Model of Secondary Progressive MS. Neurology 2016;86:P5.324
- Ochoa-Repáraz J, Colpitts SL, Kircher C, et al. Induction of gut regulatory CD39(+) T cells by teriflunomide protects against EAE. Neurol Neuroimmunol Neuroinflamm 2016;3:e291. [Crossref] [PubMed]
- Wiendl H, Gross C, Lindner M, et al. TERI-DYNAMIC: Exploring the Impact of Teriflunomide on Immune Cell Population Size, Receptor Repertoire, and Function in Patients With RRMS. Neurology 2016;86:P5.282
- Nouri M, Bredberg A, Weström B, et al. Intestinal barrier dysfunction develops at the onset of experimental autoimmune encephalomyelitis, and can be induced by adoptive transfer of auto-reactive T cells. PLoS One 2014;9:e106335. [Crossref] [PubMed]
- Kozakova H, Schwarzer M, Tuckova L, et al. Colonization of germ-free mice with a mixture of three lactobacillus strains enhances the integrity of gut mucosa and ameliorates allergic sensitization. Cell Mol Immunol 2016;13:251-62. [Crossref] [PubMed]
- Buscarinu MC, Cerasoli B, Annibali V, et al. Altered intestinal permeability in patients with relapsing-remitting multiple sclerosis: A pilot study. Mult Scler 2017;23:442-6. [Crossref] [PubMed]