Therapeutic targeting of dysregulated cellular communication
Intercellular communication represents a fundamental phenomenon in multicellular eukaryote organisms, such as in the human beings. Multicellularity has allowed developing cells involved in different works. Within the same organisms, some cells function as nutrient providers, while other cells are involved in defense responses or reproduction. Multiple cell-types join to form tissues, such as muscle, blood, vascular and brain tissue. In this scenario, signaling systems are crucial to ensure the harmonious development of multicellular structures and functions. Cells have to sense information from the outside environment, including the availability of nutrients, changes in temperature, or light levels and then the information has to be transmitted intracellularly. Considering that tissues are constituted by different cell-types which have to collaborate to carry on a particular function, the intercellular communication is a major factor (Figure 1), and dysfunctional cellular crosstalk promotes the development of various types of diseases, including atherothrombosis and cancer (1).
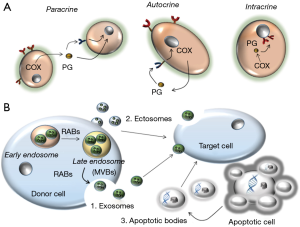
Defective intercellular communication in cancer
Within the same cell-type, such as fibroblasts, it has been shown that cell collective responses to the sensory stimulus ATP (adenosine triphosphate), is dictated not only by the stimulus concentration but also by the degree of communication within the cellular population (2). In fact, when cells interact, they form a small channel called gap junction between adjacent cells. These pores allow small molecules and ions to move from one cell to another, thus promoting the transmission of the signal to adjacent cells and increasing the sensitivity to the stimulus (3). Interesting, Potter et al. (2) found that the cancer cells are defective in the multicellular network due to reduced gap junction communication. In fact, the coculture of breast cancer cells with fibroblasts is associated with the reduction of the intercellular communicative process (4).
Direct cell-cell interaction and autacoid release in health and disease
The communication between cells also occurs through specific proteins which mediate the adhesion between cells, one important class of adhesive molecules is that of the integrins. They transmit signals to and from cells, thus sensing the environment and controlling cell shape and motility of cells (5). Specific integrins mediate the interaction of platelets with other platelets or other cell types, including endothelial cells and cancer cells. Integrins mediate platelet adhesion and aggregation two phenomena involved in the maintenance of the integrity of the vasculature (5).
Cells also communicate through the release of several soluble molecules of different chemical nature, including lipids and proteins. They are called autacoids and have the characteristic to act near the site of synthesis because they usually have a very brief lifetime in vivo due to a rapid and intense catabolism. Autacoids work through the induction of paracrine and/or autocrine signaling pathways in adjacent cells or in the same cell which has produced them, respectively. Finally, they can also induce an intracrine signaling, by acting on the producing cell before being secreted (6) (Figure 1A). Autacoids are involved in physiologic processes and act via the interaction with specific receptors thus inducing a complex network of signaling pathways which participate in a vast number of cellular responses. Interestingly, some cells, such as platelets, uptake various autacoids and store them into specific granules that will be released upon appropriate cellular stimulation. This a mechanism which can prolong the lifespan of the autacoid in the circulation. An important family of autacoids is that produced by the metabolism of arachidonic acid (AA) (7).
It is oxygenated and further transformed into a variety of lipids which mediate or modulate important physiological and pathological functions, including inflammation, cancer, and atherothrombosis. AA (20:4, ω6) is a major component of cellular membranes esterified in the sn-2 position of glycerophospholipids. Upon cell activation, AA is released by the activity of different phospholipases (PLs), including cytosolic PLA2. Then, various enzymatic pathways transform AA into the large family of eicosanoids. Among them there are: (I) the prostanoids, i.e., prostacyclin [prostaglandin(PG)I2], thromboxane A2 (TXA2), PGE2, PGD2 and PGF2α, produced by the activity of cyclooxygenases (COX-1 and COX-2) and downstream synthases (7); (II) the leukotrienes (LTs) LTC4 and LTB4 formed by the activity of 5-lipoxygenase (5-LOX) coupled to glutathione S-transferase (GST) and LTA4 hydrolase, respectively (8); (III) epoxyeicosatrienoic acid (EET) and dihydroxy acids by cytochrome p450 epoxygenase (9) (Figure 2). The important role of prostanoids in health and disease has been clearly demonstrated by the use of drugs which target specifically COX-1 and/or COX-2, i.e., the nonsteroidal anti-inflammatory drugs (NSAIDs) nonselective for COX-isozymes (named traditional NSAIDs) or selective for COX-2 (called coxibs). The use of these pharmacological tools together with that of gene-modified mice have enlightened a central role of endothelial COX-2-dependent PGI2 to protect the vascular system from an excessive adhesion of platelets which leads to the recruitment of further platelets and leukocytes (10). Altogether, these events promote the development of a chronic inflammatory response within the vasculature which is a hallmark of atherosclerosis and atherothrombosis. Moreover, the use of the NSAID aspirin, which at low-doses causes a selective inhibition of platelet function via the acetylation of COX-1, has allowed clarifying the crucial role of platelets in the thrombotic occlusion of arteries involved in blood supply to the heart and brain. Finally, the key role of COX-2-dependent PGE2 in cancer development and progression has been convincingly demonstrated by clinical and experimental evidence (11).
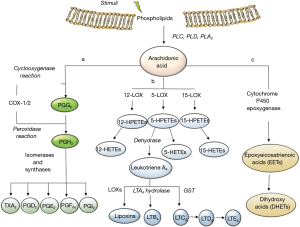
Platelets induce phenotypic changes of cells
Functions of platelets, beyond thrombosis and hemostasis, include the capacity to influence the gene expression program of other cells. This may occur via the biosynthesis and release of prostanoids, including PGE2 and the secretion of proteins, such as PDGF and TGF-beta which are stored in α-granules. These molecules present in the platelet releasate influence the phenotype of cancer cells, leading to enhanced invasiveness and malignancy (12,13). These effects are mediated by the overexpression of COX-2, which increases survival of cancer cells, and by the induction of epithelial-mesenchymal transition (EMT), which promote migration and invasiveness of cancer cells. To realize these effects, the direct interaction of platelets with cancer cells synergizes with the release of soluble factors by platelets (12,13).
A vast number of results have shown that an anti-metastatic effect is associated with the use of therapeutics which interrupt the crosstalk between cancer cells and platelets. This is strongly supported by the clinical efficacy of the antiplatelet agent low-dose aspirin to reduce the incidence and mortality for cancer (14).
Extracellular vesicle trafficking and signaling in cellular communication
Another way that cells may use to communicate is via the biogenesis of extracellular vesicles. They are classified as exosomes, shedding vesicles (ectosomes, microparticles) or apoptotic bodies (15).
Exosomes have a diameter of 30–100 nm and are intraluminal vesicles (ILVs) formed inside the multivesicular bodies (MVBs). They are released by the exocytosis of MVBs. RAB proteins have been shown to have a role in exosome secretion (16).
Ectosomes are shedding vesicles larger than exosomes (with a diameter of 100 nm to 1 µm) and are produced by direct plasma membrane blebbing in regions of lipid rafts (i.e., specialized lipid microdomains constituted by cholesterol associated with sphingomyelin and glycerophospholipids). Tumor cells constitutively shed scores of ectosomes. In contrast, in normal cells this phenomenon is small, but the rate of release can be increased by cell activation. Finally, cells can release apoptotic bodies which are larger than ectosomes or exosomes (>1 µm in diameter) and are released as blebs of apoptotic cells (17) (Figure 1B).
Extracellular vesicles contain proteins, mRNAs, and microRNAs (miRNAs) that can be transferred to nearby cells or a distant cell via the blood circulation. However, the mechanisms involved in the uptake and the integration of these molecules into the recipient cell are poor understood (17).
Several lines of evidence suggest that the mRNAs of secreted vesicles derived from different types of cells (including embryonic stem cells or tumor cells) can be delivered to target cells where they are translated into functional proteins. Also, miRNAs can be delivered to target cells (18). Moreover, it has been reported that the phagocytosis of apoptotic bodies leads to the transfer of DNA into fibroblasts and endothelial cells (19).
Colorectal cancer cell-derived exosomes have important roles in tumor progression and the formation of distal metastasis by delivering miRNAs, mRNAs, and proteins.
Recently, it has been shown that hypoxic colorectal cancer cell-derived exosomes promote angiogenesis through the delivery of Wnt/β-catenin signaling in endothelial cells. Interestingly, RAB27a knockdown suppressed exosome secretion by colorectal cancer cells associated with the inhibition of proliferation and migration of endothelial cells (20).
Platelet-dependent cellular communication via the delivery of vesicle cargo
Upon activation, platelets release exosomes and microparticles (MPs). Recently, Dervin et al. (21) have characterized the proteome of human platelet exosomes thus showing that a population of these vesicles carries active Wnt glycoproteins on their surface that can modulate Wnt signaling activity in both endothelial and monocytic cells (21).
We have recently shown that incubation of the HT29 colon cancer cell line with platelets is associated with a time-dependent induction of β-catenin translocation into the nucleus of HT29 cells causing the rapid increase in COX-2 mRNA levels (22). This effect was associated with the detection of Wnt3a in the supernatant of platelet-HT29 cell cocultures but not in that of HT29 cells cultured alone. Altogether, these results suggest that Wnt released by activated platelets leads to β-catenin translocation into the nucleus, thus causing the activation of target genes, such as COX-2, of the transcription factor T-cell factor (Tcf)/lymphocyte enhancer (Lef) (22).
miRNAs are short (20–25 bp) RNAs that can regulate gene expression through various mechanisms. Profiling miRNAs (miRNome) of exosomes derived from human platelets has been recently characterized. Platelets contain miRNAs which may regulate platelet function. Moreover, platelet exosomes enriched with miRNAs can be secreted upon activation to influence the behavior of targeted cells (23).
Platelets can uptake different molecules, including genetic material, thus, these cells can change their cargo depending on a pathological environment. It has been shown that tumor-associated blood platelets provide accurate information on the location and molecular composition of the primary tumor. This information can be used for early detection of cancer or its progression (prognostic information), real-time monitoring of treatment (24). However, the different platelet phenotype of cancer patients may also play a significant role in the progression of the disease through the possible delivery of pathological signals to stromal cells and cancer cells.
Conclusions and perspectives
The rapid development of new omic technologies, such as genomics, transcriptomics, proteomics and metabolomics together with that of microscopic imaging platform technology combined with the development of novel optical biosensors and sophisticated image analysis solutions have allowed making a leap forward in understanding how cells communicate with each other. Importantly, it has been clarified that cells can exchange genetic material which can be incorporated into the recipient cells but also different proteins. In this context, it has been enlightened the role of extracellular vesicles in the crosstalk between cells. The release of the various types of vesicles may be altered in pathological conditions thus contributing to the development of different diseases (25). In fact, extracellular vesicles can contain a different cargo depending on the clinical conditions. Vesicles released from nucleated cells contain different molecules derived from the cell itself.
In contrast, the anucleate platelet uptakes various factors present in the environment which are then released as soluble forms or within vesicles. The delivery of the cargo of exosomes to the recipient cell may induce important changes in cellular functions, thus contributing to the development of diseases, including cancer (25). This knowledge opens the avenue to the development of novel therapeutic strategies to prevent cancer and other pathological conditions, including atherothrombosis and tissue fibrosis, by affecting the release of vesicles and their delivery to target cells. To realize this aim, we have to improve our knowledge on the biogenesis of extracellular vesicles and their trafficking. The time is ripe to achieve this goal because we own the appropriate technology and experimental models. The new information should be rapidly translated to humans to confirm the efficacy of these novel therapeutics approaches to prevent and cure different diseases.
Acknowledgements
Funding: This work was supported by the Ministero dell’Istruzione, dell’Università e della Ricerca (MIUR) (Grant PRIN 2010-2011, protocol number 2010FHH32M), and Associazione Italiana per la Ricerca sul Cancro (Grant IG-12111) (to P Patrignani).
Footnote
Conflicts of Interest: The authors have no conflicts of interest to declare.
References
- Ruch RJ. Intercellular communication, homeostasis, and toxicology. Toxicol Sci 2002;68:265-6. [Crossref] [PubMed]
- Potter GD, Byrd TA, Mugler A, et al. Communication shapes sensory response in multicellular networks. Proc Natl Acad Sci U S A 2016;113:10334-9. [Crossref] [PubMed]
- Kumar NM, Gilula NB. The gap junction communication channel. Cell 1996;84:381-8. [Crossref] [PubMed]
- Alberts B, Johnson A, Lewis J, et al. Molecular biology of the cell. 4th edn. New York: Garland Science, 2002.
- Sorrentino S, Studt JD, Medalia O, et al. Roll, adhere, spread and contract: structural mechanics of platelet function. Eur J Cell Biol 2015;94:129-38. [Crossref] [PubMed]
- Prescott SM. Is cyclooxygenase-2 the alpha and the omega in cancer? J Clin Invest 2000;105:1511-3. [Crossref] [PubMed]
- Ricciotti E, FitzGerald GA. Prostaglandins and inflammation. Arterioscler Thromb Vasc Biol 2011;31:986-1000. [Crossref] [PubMed]
- Lewis RA, Austen KF, Soberman RJ. Leukotrienes and other products of the 5-lipoxygenase pathway. Biochemistry and relation to pathobiology in human diseases. N Engl J Med 1990;323:645-55. [Crossref] [PubMed]
- Spector AA. Arachidonic acid cytochrome P450 epoxygenase pathway. J Lipid Res 2009;50 Suppl:S52-6. [Crossref] [PubMed]
- Grosser T, Fries S, FitzGerald GA. Biological basis for the cardiovascular consequences of COX-2 inhibition: therapeutic challenges and opportunities. J Clin Invest 2006;116:4-15. [Crossref] [PubMed]
- Dixon DA, Blanco FF, Bruno A, et al. Mechanistic aspects of COX-2 expression in colorectal neoplasia. Recent Results Cancer Res 2013;191:7-37. [Crossref] [PubMed]
- Dovizio M, Maier TJ, Alberti S, et al. Pharmacological inhibition of platelet-tumor cell cross-talk prevents platelet-induced overexpression of cyclooxygenase-2 in HT29 human colon carcinoma cells. Mol Pharmacol 2013;84:25-40. [Crossref] [PubMed]
- Labelle M, Begum S, Hynes RO. Direct signaling between platelets and cancer cells induces an epithelial-mesenchymal-like transition and promotes metastasis. Cancer Cell 2011;20:576-90. [Crossref] [PubMed]
- Patrignani P, Patrono C. Aspirin and Cancer. J Am Coll Cardiol 2016;68:967-76. [Crossref] [PubMed]
- Raposo G, Stoorvogel W. Extracellular vesicles: exosomes, microvesicles, and friends. J Cell Biol 2013;200:373-83. [Crossref] [PubMed]
- Hsu C, Morohashi Y, Yoshimura S, et al. Regulation of exosome secretion by Rab35 and its GTPase-activating proteins TBC1D10A-C. J Cell Biol 2010;189:223-32. [Crossref] [PubMed]
- Mittelbrunn M, Sánchez-Madrid F. Intercellular communication: diverse structures for exchange of genetic information. Nat Rev Mol Cell Biol 2012;13:328-35. [PubMed]
- Quesenberry PJ, Aliotta J, Deregibus MC, et al. Role of extracellular RNA-carrying vesicles in cell differentiation and reprogramming. Stem Cell Res Ther 2015;6:153. [Crossref] [PubMed]
- Ehnfors J, Kost-Alimova M, Persson NL, et al. Horizontal transfer of tumor DNA to endothelial cells in vivo. Cell Death Differ 2009;16:749-57. [Crossref] [PubMed]
- Huang Z, Feng Y. Exosomes derived from hypoxic colorectal cancer cells promotes angiogenesis through Wnt4 induced β-catenin signaling in endothelial cells. Oncol Res 2016. [Epub ahead of print]. [PubMed]
- Dervin F, Wynne K, Maguire PB. Human platelet exosome proteomics leads to the identification of WNT positive exosomes which impact canonical WNT signalling in target cells. Blood 2014;124:2758. [PubMed]
- Dovizio M, Alberti S, Sacco A, et al. Novel insights into the regulation of cyclooxygenase-2 expression by platelet-cancer cell cross-talk. Biochem Soc Trans 2015;43:707-14. [Crossref] [PubMed]
- Dempsey E, Dervin F, Maguire PB. Platelet derived exosomes are enriched for specific microRNAs which regulate WNT signalling in endothelial cells. Blood 2014;124:2760. [PubMed]
- Best MG, Sol N, Kooi I, et al. RNA-Seq of tumor-educated platelets enables blood-based pan-cancer, multiclass, and molecular pathway cancer diagnostics. Cancer Cell 2015;28:666-76. [Crossref] [PubMed]
- Yuana Y, Sturk A, Nieuwland R. Extracellular vesicles in physiological and pathological conditions. Blood Rev 2013;27:31-9. [Crossref] [PubMed]