Extracellular vesicle-mediated communication in host-parasite interactions: insight from Fasciola hepatica
Extracellular vesicles in the host-parasite interaction
In recent years, extracellular vesicles (EVs) have been accepted as a new intercellular communication system that mediate the transfer of proteins, lipids, mRNA, microRNA and other non-coding RNA species. Special attention has been paid to the role of EVs in the establishment and progression of human diseases. Indeed, perturbing EV production to modulate their pathological effects is an attractive therapeutic option that has been successful in a number of diseases, including cancer (1). To the same extent, several studies have described the contribution of parasite-derived EVs to the modulation of the host immune system (2-4) or the pathological effects on host cells (5). Tools such as transcriptomics and proteomics, have been particularly useful for identification of the immunomodulatory molecules that parasites package into EVs (6). A better understanding of how parasite EVs are produced and interact with host cells may open new avenues for parasite control, since the selective inhibition of these would prevent the delivery of potent immunomodulators that induce a host immune phenotype that favors parasite survival.
It is in this context that we established a definitive characterization of the total secretome of the zoonotic parasite Fasciola hepatica (6), one of the causative agents of the trematode foodborne disease known as fascioliasis. Whilst primarily regarded as a disease of livestock, it is estimated that F. hepatica infects between 2 and 17 million people worldwide, with a further 180 million living at risk of infection (7). Resistance to triclabendazole, the frontline chemical treatment against Fasciola, is rapidly spreading and highlights the need for novel control strategies against the parasite (8).
Characterization of the EVs released by Fasciola hepatica
EVs released by the parasite during in vitro culture were isolated using ultracentrifugation and ultrafiltration and subsequently analyzed by transmission electron microscopy (TEM) and mass spectrometry. One of the pivotal findings was that Fasciola secretes at least two sub-populations of EVs of varying size that bear different cargo molecules and may be released from distinct sites within the parasite. TEM revealed that the larger EVs are released from the specialized cells that line the parasite gastrodermis and are specifically enriched in the zymogen of the 37 kDa cathepsin L peptidase, which mainly performs a digestive function (9). Proteomics and transcriptomics data provided insight into molecular origin of the smaller exosome-like EV population. Whilst a previous exosome characterization study described only the total vesicular content (10), we wanted to obtain a more detailed picture of the vesicle architecture. Thus, we performed a differential extraction of membrane associated proteins—more likely to participate in interactions with host cells—and those packaged as cargo—envisaged to be delivered into host cells. Mass spectrometry analysis revealed a significant number of proteins belonging to the ESCRT pathway of EV biogenesis and vesicular transport. Together with the abundance of shared tegumental proteins (11), these results suggested that at least some EVs from Fasciola originate from multivesicular bodies within the tegumental syncytium before being shed from the apical plasma membrane. Furthermore, transcriptomics analysis indicated that whilst the molecular “machinery” required for EV biogenesis is constitutively expressed (albeit at low levels) across the intra-mammalian developmental stages of the parasite, the cargo molecules packaged within the EVs are developmentally regulated. This suggests that there is a constant release of EVs containing effector molecules finely tuned to the defensive needs of the developing parasite as it migrates through various host tissues.
Future research directions
Although this study provided insight into the mechanisms that helminth parasites use to produce EVs, it raised a considerable number of questions that need to be addressed before designing a rational therapeutic approach for this or other helminth parasites (12). Our proteomics data largely supports an ESCRT-dependent origin for the exosome-like EVs released by F. hepatica (Table 1). However, further research is needed to determine the specific roles of individual pathway members during exosome biogenesis in liver fluke—e.g., by RNAi mediated gene silencing, which is functional in this parasite (13). Additionally, before members from these pathways can be selected as possible targets for anti-parasite drugs, it remains to be elucidated whether mammalian exosome biogenesis pathways are conserved in F. hepatica or if novel routes are used by the parasite. The presence of orthologues from ESCRT-independent pathways, such as sphingomyelinase and various members of the tetraspanin family in its secretome (6) (Table 1), could indicate that F. hepatica uses hybrid routes for EV release as have been described in some mammalian cell types (14). However, this may also be due to the heterogeneity of vesicle populations in the isolated EVs. The lack of specific markers to distinguish EV sup-populations is a common issue in the field (15) and therefore, to establish a broader set of markers would help to discriminate EV populations and track down their site(s) of production and release from the parasite. We found that the zymogen of cathepsin L, specifically enriched in the larger EVs released by the parasite, constitutes a potential marker for this type of vesicle. Our differential extraction approach, which separated membrane-associated proteins from those packaged into the lumen of exosome-like vesicles, helped to identify exosomal markers common to many species as well as potential parasite-specific molecules, such as tetraspanins.
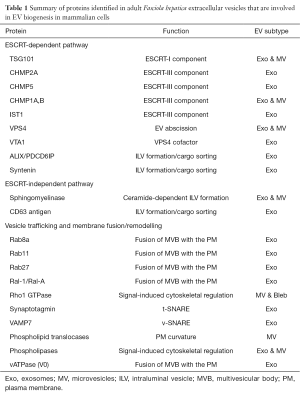
Full table
Transcriptome analysis indicated that members of EV biogenesis pathways are constitutively expressed during the intra-mammalian developmental stages of the parasite. This is in agreement with reports of constitutive release of exosomes via the endosomal pathway in mammalian cells (16). On the other hand, shedding of microvesicles from the plasma membrane usually occurs in response to a stimulus. It is well documented that F. hepatica, as well as other platyhelminth and nematode parasites, shed vesicles (usually referred to as blebs) from their cuticle/tegument in response to drug treatment or humoral immune challenge (17,18). Although it has been suggested that blebbing is an attempt by helminths to replenish tegument that has been lost/damaged due to drug action (19), this mechanism might constitute a defensive response of the parasite to reduce drug effective concentrations by packing them into vesicles that are immediately disposed of. To determine whether the molecular pathways involved in bleb production are the same as microvesicle production could provide a better understanding of drug resistance in helminth parasites, and a means to counter it.
Whilst EVs secreted by helminths can be internalized by host cells and regulate host immune and inflammatory responses (2-5,10), it is unclear to what extent Fasciola EVs contribute to maintaining a Th2/regulatory environment that is permissive to fluke survival and reproduction. Once we gain a better understanding of these issues, the selective disruption of key pathways involved in EV biogenesis, or blocking the EV-driven delivery of parasite immunomodulators to host cells, might prove to be an efficient way to achieve parasite control in the future.
Acknowledgements
Funding: MW Robinson is supported by a grant (BB/L019612/1) from the Biotechnology and Biological Sciences Research Council (BBSRC).
Footnote
Conflicts of Interest: The authors have no conflicts of interest to declare.
References
- El Andaloussi S, Mäger I, Breakefield XO, et al. Extracellular vesicles: biology and emerging therapeutic opportunities. Nat Rev Drug Discov 2013;12:347-357. [Crossref] [PubMed]
- Buck AH, Coakley G, Simbari F, et al. Exosomes secreted by nematode parasites transfer small RNAs to mammalian cells and modulate innate immunity. Nat Commun 2014;5:5488. [Crossref] [PubMed]
- Wang L, Li Z, Shen J, et al. Exosome-like vesicles derived by Schistosoma japonicum adult worms mediates M1 type immune-activity of macrophage. Parasitol Res 2015;114:1865-1873. [Crossref] [PubMed]
- Zamanian M, Fraser LM, Agbedanu PN, et al. Release of small RNA-containing exosome-like vesicles from the human filarial parasite Brugia malayi. PLoS Negl Trop Dis 2015;9:e0004069. [Crossref] [PubMed]
- Chaiyadet S, Sotillo J, Smout M, et al. Carcinogenic liver fluke secretes extracellular vesicles that promote cholangiocytes to adopt a tumorigenic phenotype. J Infect Dis 2015;212:1636-45. [Crossref] [PubMed]
- Cwiklinski K, de la Torre-Escudero E, Trelis M, et al. The extracellular vesicles of the helminth pathogen, Fasciola hepatica: biogenesis pathways and cargo molecules involved in parasite pathogenesis. Mol Cell Proteomics 2015;14:3258-73. [Crossref] [PubMed]
- Mas-Coma S, Bargues MD, Valero MA. Fascioliasis and other plant-borne trematode zoonoses. Int J Parasitol 2005;35:1255-1278. [Crossref] [PubMed]
- Molina-Hernández V, Mulcahy G, Pérez J, et al. Fasciola hepatica vaccine: we may not be there yet but we're on the right road. Vet Parasitol 2015;208:101-111. [Crossref] [PubMed]
- McVeigh P, Maule AG, Dalton JP, et al. Fasciola hepatica virulence-associated cysteine peptidases: a systems biology perspective. Microbes Infect 2012;14:301-310. [Crossref] [PubMed]
- Marcilla A, Trelis M, Cortés A, et al. Extracellular vesicles from parasitic helminths contain specific excretory/secretory proteins and are internalized in intestinal host cells. PLoS ONE 2012;7:e45974. [Crossref] [PubMed]
- Wilson RA, Wright JM, de Castro-Borges W, et al. Exploring the Fasciola hepatica tegument proteome. Int J Parasitol 2011;41:1347-1359. [Crossref] [PubMed]
- de la Torre-Escudero E, Bennett AP, Clarke A, et al. Extracellular vesicle biogenesis in helminths: more than one route to the surface? Trends Parasitol 2016;32:921-929. [Crossref] [PubMed]
- McVeigh P, McCammick EM, McCusker P, et al. RNAi dynamics in juvenile Fasciola spp. liver flukes reveals the persistence of gene silencing in vitro. PLoS Negl Trop Dis 2014;8:e3185. [Crossref] [PubMed]
- Vilette D, Laulagnier K, Huor A, et al. Efficient inhibition of infectious prions multiplication and release by targeting the exosomal pathway. Cell Mol Life Sci 2015;72:4409-27. [Crossref] [PubMed]
- Kowal J, Arras G, Colombo M, et al. Proteomic comparison defines novel markers to characterize heterogeneous populations of extracellular vesicle subtypes. Proc Natl Acad Sci U S A 2016;113:E968-77. [Crossref] [PubMed]
- Colombo M, Raposo G, Théry C. Biogenesis, secretion, and intercellular interactions of exosomes and other extracellular vesicles. Annu Rev Cell Dev Biol 2014;30:255-89. [Crossref] [PubMed]
- Robinson MW, Trudgett A, Hoey EM, et al. The effect of the microtubule inhibitor tubulozole-C on the tegument of triclabendazole-susceptible and triclabendazole-resistant Fasciola hepatica. Parasitol Res 2003;91:117-29. [Crossref] [PubMed]
- Abdeen SH, Reda ES, El-Shabasy EA, et al. Ultrastructural changes of adult Schistosoma mansoni worms recovered from C57BL/6 mice passively immunized with normal and vaccinated rabbit sera in vivo. Parasitol Res 2012;110:37-47. [Crossref] [PubMed]
- Bennett CE, Hughes DL, Harness E. Fasciola hepatica: changes in tegument during killing of adult flukes surgically transferred to sensitized rats. Parasite Immunol 1980;2:39-55. [Crossref]