Biomarker-guided antibiotic therapy—strengths and limitations
Introduction
The emergence and spread of antimicrobial resistance has been identified as one of the most important problems for human health, with major impact both on clinical outcome and on healthcare associated costs (1). The overuse and the misuse of antibiotics over the past decades has resulted in a widespread and strong selective pressure on the microbiota and is unanimously recognized as one of the major causative factors of the widespread of antimicrobial resistance (2-4). A proper antibiotic prescribing strategy must be part of an integrative process-of-care, which assures a prompt recognition of infection, not colonization, and a timely initiation and duration of an appropriate antimicrobial therapy. Such strategy should be based on strong and objective data; unfortunately, there is no gold standard test to diagnose infection (4), clinical information lacks accuracy particularly after the initiation of therapy (3) and microbiological results—either positive or negative—are often late in the course of therapy. As a consequence, antibiotics are commonly prescribed without a definitive diagnosis, namely in severe infections, because a delay in treatment is associated with worse outcome (4).
What is a biomarker? What characteristics should an ideal biomarker possess?
Biomarkers are biological characteristics that are objectively measured and used as an indicator of a physiological or pathological pathways or a pharmacologic response to therapeutic interventions (5). They emerged in recent years as a tool to help physicians to triage, diagnose, stratify risk, and monitor clinical course as well as response to antibiotics. Such surrogate role implies that an ideal biomarker for infection must possess diagnostic, prognostic and follow-up therapy characteristics (3). Before being used clinically, any biomarker must be evaluated regarding the laboratory methods used to measure it (analytical validation), the evidence supporting its association with one pathological process (qualification) and the feasibility of its clinical use (utilization) (6).
The most important characteristics an ideal biomarker of infection must possess are summarized in Table 1.
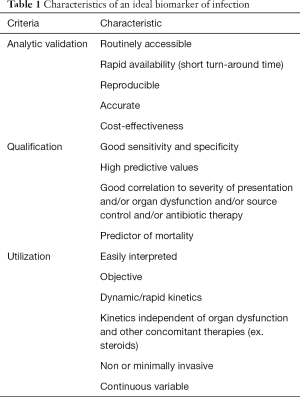
Full table
Biomarkers used in clinical practice
Although more than a hundred biomarkers have been studied, only a limited number of them became routinely available in clinical practice. C-reactive protein (CRP) and procalcitonin (PCT) are the more frequently studied and used biomarkers (7).
Serum CRP is an acute phase protein synthetized exclusively in the liver. Its secretion is initiated 4 to 6 h after an inflammatory insult (effect mediated by cytokines namely interleukin-6) and its concentration doubles every 8 h with a peak at 36–50 h (4). The sole determinant of CRP plasmatic levels is its synthesis rate, which is proportional to the intensity of the inflammatory insult (8). It starts falling after the removal of the primary inflammatory stimulus following a first-order kinetic elimination pattern with a half-life of 19 h. Its production and elimination is not influenced by renal replacement therapy or immunosuppression (both systemic steroids and neutropenia). It has a sensitivity of 68–92% and a specificity of 40–67% as a marker of bacterial infection (9). The available assays for CRP measurement are reliable, stable, reproducible, rapid, inexpensive (€4 in Europe) and present an acceptable limit of detection (0.3–5 mg/L).
PCT is a precursor protein of calcitonin that can be produced ubiquitously throughout the body (10). It is released 3–4 h after an inflammatory stimulus with a plasmatic peak within 6–24 h (8) and a half-life ranging from 22 to 35 h. Its plasmatic levels are markedly influenced by renal function, different techniques of renal replacement therapy and neutropenia. It showed a sensitivity of 77% and a specificity of 79% for early diagnosis of sepsis in critically ill patients (9). The laboratory assay used to measure plasma PCT must assure a high sensitivity in order to validate study results. Only the immunoassay based on a time-resolved amplified cryptate emission (TRACE) technology warrants an ultrasensitive analysis with a limit of detection of 0.06 ng/mL and the appropriate quickness to be useful to be applied at bedside but at a high cost (€25–30 in Europe) (4). Other available assays (semi-quantitative immunochromatographic method—PCT-Q, Brahms; luminescence immunoassay—PCT LIA, Brahms) are less sensitive and should not be used for antibiotic stewardship (4).
CRP-guided antibiotic therapy
CRP has been analysed in multiple clinical contexts but only a small number of studies have focused on its use for optimizing antibiotic therapy. Most data come from paediatric population: a recent prospective cohort study by Downes et al. (11) (OASIS Study) with 85 patients identified thresholds for biomarker combinations at patient admission (CRP plus serum amyloid A and CRP plus PCT) to identify children with systemic inflammatory response syndrome (SIRS) in whom antibiotics might be discontinued at 48 h after admission. These results corroborate data from other prospective cohort studies performed in the last two decades pointing to the fact that CRP can be used to help discontinuing antibiotic therapy in children (12,13).
In primary care, CRP improves the assessment of severity of infection and extent of inflammation (14) and performs better in predicting the diagnosis of pneumonia than any individual or combination of clinical signs and symptoms (15). Cals et al. (15) tested an algorithm in which patients with signs and symptoms of lower respiratory tract infections (n=431) were prescribed with antibiotics based on CRP value versus usual care. General practitioners in the CRP test group prescribed significantly fewer antibiotics both at first consultation (31% vs. 53%, P=0.02) and during the 28 days’ follow-up (45% vs. 58%, P<0.01) with no statistically significant difference in re-consultations, clinical recovery and patients’ satisfaction. Antibiotic overprescribing was also reduced when CRP was tested in patients with acute exacerbation of chronic obstructive pulmonary disease (16). Other studies (17,18) and a Cochrane Review (19) demonstrated reduced antibiotic prescription with CRP testing which led to its incorporation in the National Institute for Health and Care Excellence (NICE) guidelines for the diagnosis of pneumonia. Besides improving antibiotic stewardship, CRP testing proved to be cost-effective in the management of lower respiratory tract infections (20,21).
Several studies are underway (3) specifically testing the value of CRP on antibiotic discontinuation in adult patients at secondary and tertiary hospitals. So far there is evidence that CRP was as useful as PCT in reducing antibiotic use in critically ill septic patients (22,23).
PCT-guided antibiotic therapy
PCT is the most widely studied biomarker for antibiotic stewardship (3). It has been tested as an aid to the initiation and/or discontinuation of antibiotics, both in children and adults presenting with distinct sources of infection and in different scenarios, from primary care to emergency departments, hospital wards and intensive care units (ICU). Early observational studies defined PCT cut-off ranges according to which bacterial aetiology for infection was very unlikely (<0.1 ng/mL), unlikely (0.1–0.25 ng/mL), likely (0.25–0.5 ng/mL) and very likely (>0.5 ng/mL); antibiotics were discourage when PCT <0.25 ng/mL and encouraged whenever PCT >0.25 ng/mL (24). Optimal PCT cut-offs have been established for different clinical settings and/or types of infection; some showed safety and efficacy in randomized-controlled trial (RCT) while others were only tested in observational studies (25-27). PCT cut-off ranges and some overruling criteria have been included in algorithms to help decide on the initiation and duration of antibiotic therapy and have been validated and subsequently applied in different RCTs, with slight differences between studies.
A meta-analysis and a Cochrane review performed by Schuetz et al. (28,29) analysed 14 RCTs including more than 4,000 patients with respiratory tract infections and sepsis. The use of clinical algorithms based on PCT levels resulted in a significant reduction of antibiotic consumption—risk reduction of initial antibiotic therapy odds ratio (OR) =0.24 [95% confidence interval (CI), 0.2–0.29] and overall antibiotic exposure OR =0.1 (95% CI, 0.07–0.14)—across different clinical settings with lower prescribing rates in primary care and emergency departments and shorter duration of therapy in ICU patients. There were no significant differences in mortality rates or treatment failure between PCT-guided antibiotic stewardship and control patients.
Lin et al. (30) meta-analysed four trials involving 679 patients with acute exacerbations of chronic obstructive pulmonary disease. The results suggest that PCT-guided antibiotic therapy reduces the number of antibiotic prescriptions (OR =0.26; 95% CI, 0.14–0.5, P<0.0001) without compromising clinical success or increasing mortality in comparison to standard care. Furthermore, there was no statistically significant difference in the rate or readmission and exacerbation up to a 6-month follow-up period.
Andriolo et al. (31) reviewed the effectiveness of PCT evaluation in reducing mortality and duration of antibiotic therapy in adults with sepsis. Ten trials with 1,215 patients were included and no significant differences in mortality were found, both at 28 days, at longest follow-up, at ICU discharge and at hospital discharge between PCT and non-PCT groups. Combined study results (n=313) on time receiving antibiotics showed a reduction of 1.28 days (95% CI, 0.61–1.95) in the PCT group but with very low quality of evidence. No subgroup analysis (ex. patients in shock, ICU setting, specific infection foci, specific PCT cut-off) was performed because there were an insufficient number of studies with the same specific characteristics to be combined in the same subgroup.
Adult ICU patients
Studies on antibiotic stewardship performed in critical care patients analyse the role of biomarker for initiation of antibiotic therapy in non ICU-acquired infections, initiation of antibiotic therapy in ICU-acquired infections and duration of antibiotic therapy.
Layios et al. (32) applied a PCT algorithm where antibiotics were discouraged if PCT <0.25 ng/mL and encouraged if PCT >1 ng/mL in 509 patients suspected of infection on admission or during ICU stay and found no difference in the rate of initiation of therapy, percentage of days on antibiotics or overall defined daily dose of antibiotics between PCT and control groups. Bouadma et al. (33) in the PRORATA trial (n=630) used a similar algorithm and showed a reduction of antibiotic exposure in the PCT group (more days without antibiotics, 14.3±9.1 vs. 11.6±8.2 days in control group, 95% CI, P<0.0001) and a 7% absolute risk reduction for initiating antibiotics. Twenty-eight-day mortality was similar between groups.
Jensen et al. (34) (PASS trial) tested, in a large RCT, the diagnostic value of PCT for ICU-acquired infections with daily PCT measurements using a threshold of 1 ng/mL or not decreasing >10%/day to identify patients at risk and consequently starting protocoled empiric antibiotic therapy, the “alert PCT” concept (35). Results showed higher large-spectrum antibiotic consumption, more days on antibiotics, prolonged length of mechanical ventilation and ICU stay in the “alert PCT” arm.
PCT-guided algorithms used to stop antibiotics have been widely studied and validated in several RCT. All were able to decrease the duration of antibiotic therapy in ICU patients, although some differences regarding stopping rules, population enrolled and PCT thresholds in PCT-guided groups as well as duration of antibiotic therapy in control groups should be pointed out. The ProVAP and PRORATA studies used a threshold to stop therapy of PCT <0.5 ng/mL from the third day onwards after starting antibiotic or an >80% drop from maximal PCT recorded value (and PCT <1 ng/mL in the ProVAP trial). The duration of antibiotic therapy was shorter in the PCT-guided group with an absolute risk reduction of 2.7 days (33).
Jong et al. (36) prospectively analysed more than 1,500 patients in the SAPS study and found consistent data regarding shorter duration of antibiotic therapy (absolute difference 1.22 days, 95% CI, 0.65–1.78, P<0.0001) and decreased volume of prescribed antibiotics (absolute difference 2.69 daily defined doses, 95% CI, 1.26–4.12, P<0.0001) in the PCT-guided group versus standard-of-care group. At 28 days after randomisation a statistically significant reduction in mortality was showed in the PCT-guided group (absolute difference 5.4%, 95% CI, 1.2–9.5, P=0.012) that lasted until 1 year later (absolute difference 6.1%, 1.2–10.9, P=0.0158; HR 1.26, 1.07–1.49, P=0.006). This relevant impact on mortality was also statistically significant when only the group of patients who completed antibiotic therapy on ICU were analysed (n=995)—absolute reduction on mortality 6.6% (95% CI, 1.3–11.9, P=0.015) at 28 days and 7.4% (1.3–13.8, P=0.019) at 1 year, favourable to the PCT-group. The proposed association between the reduced exposure to antibiotics (both in duration and dose) and a survival benefit is thrilling but not strongly evidence-supported. Chastre et al. (37) prospectively randomised 401 patients with ventilator-associated pneumonia (VAP) and found similar clinical effectiveness with an 8-day versus a 15-day antibiotic regimen; however, no difference in mortality attributable to the higher number of antibiotic free days was documented. Pugh et al. (38) reviewed six RCT comparing short (7- or 8-day) versus prolonged (10- to 15-day) antibiotic course in 1,088 patients with hospital acquired pneumonia (including VAP). They demonstrated that the proposed decrease of antibiotic exposure was associated with similar clinical outcomes, namely mortality.
A PCT algorithm with a low cut-off value of 0.1 ng/mL for antibiotic cessation in nearly 400 ICU septic patients was tested by Shehabi et al. (39) who failed to show a significant reduction in overall antibiotic exposure. Subgroup analysis revealed that patients with positive microbiological results or septic shock had higher PCT values during the study days and that a slow PCT decline over the first 72 h was an independent predictor of hospital and 90-day all-cause mortality.
The principal characteristics of the RCTs assessing PCT-guided antibiotic stewardship in adult critical care patients are described in Table 2.
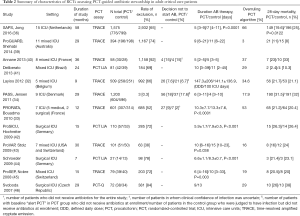
Full table
The recently published Surviving Sepsis Campaign (SSC) 2016 International Guidelines highlight the importance of biomarkers (especially PCT) to support shortening the duration of antimicrobial therapy in septic patients and the discontinuation of empiric antibiotics in patients considered to have sepsis at admission but who evolved with limited clinical evidence of infection (47).
Almost every patient enrolled in studies with PCT-guided algorithms had a clinically documented site of infection (7) and the majority of patients had a positive microbiological result. If the primary source of infection is unknown, data regarding the usefulness of PCT-guided antibiotic therapy is scarce (7). In patients with microbiologically non-documented infections the PCT-guided therapy arm had a non-statistically significant decrease in antibiotic day and similar mortality rate (33).
The PRORATA trial (33) included 98 immunocompromised patients and subgroup analysis revealed that the PCT arm had significant reduced duration of antibiotic therapy with no effect on morbi-mortality and that PCT may be used safely in immunocompromised patients. Bone marrow transplant and febrile neutropenia patients however were excluded from enrolment. Recent data showed that in febrile neutropenic patients, PCT-guided protocol did not reduce the use of antibiotics but could be a marker of bacteraemia (PCT cut off of 0.5 ng/mL with sensitivity of 52% and specificity of 76.5%).
Data regarding rates of superinfection and relapses are inconsistent between studies and the severity of infection does not appear to alter substantially the impact of PCT-guided algorithms on the reduction of antibiotic therapy duration. Both length of ICU stay and all-cause mortality rate were comparable between groups.
Data interpretation
Although it seems to exist a high strength of evidence for PCT-guided discontinuation of antibiotic therapy in adult ICU patients with sepsis and for PCT-guided initiation of antibiotic therapy for lower respiratory tract infection it is essential to critically analyse and interpret results.
Control group management according to the standard of care is a substantially subjective concept. The ProVAP and the PRORATA trials, which together enrolled more than 700 patients, defined a minimum fix duration of antibiotic therapy for control group: the ProVAP determined 15 days for dealing with VAP and the PRORATA set 7 to 10 days for community-acquired pneumonia—with extended therapy to 14 days whenever microbiological isolates of Legionella pneumophila, Mycoplasma pneumonia or Chlamydia pneumonia are present—and 8 to 15 days for VAP. Schuetz et al. (28,29) when subanalysing nearly 600 ICU patients found a median reduction of antibiotic therapy of 3.21 (2.10–4.32) days from a median 12 [8–18] days in control group to a median 8 [5–15] days in PCT-group. In patients with VAP, time on antibiotic therapy increased to a median 11 [6–17] days in PCT-guided group versus 14 [9–19.5] days in control group. Such long course of antibiotics is exceedingly higher than the known effective and safe 6 to 8 days of antibiotic therapy in VAP (37,48). This fact raises the question if the so-called standard of care corresponds to the best care and if the PCT-guided approach simply validates a shorter course of antibiotics as the best care.
Exclusion criteria were tremendously inconsistent between studies with high rates of exclusion (>35%) in the majority of them. When a throughout analysis of the study methodology is performed it becomes clear that common clinical situations were excluded, both infectious—infective endocarditis, bone and joint infection, acute mediastinitis, intracerebral or intra-abdominal abscesses or infections caused by Pseudomonas aeruginosa, Acinetobacter baumannii—that may require long-course antibiotics and non-infectious immunosuppressed patients. The compliance with PCT-guided algorithms is another indicator of the clinical applicability of each study. The overruling of PCT algorithm may occur from inclusion to the end of the trial, when the physician decides to start or prolong antibiotic therapy despite a low PCT level or when he/she decides to stop antibiotic even though PCT level is high. The PRORATA trial presented an overruling rate of 53%, which was much higher than in all other studies. No specific subgroup analysis was undertaken to access the outcome of overruled patients as well as the per-protocol treated patients. The low rate of compliance of PCT-guided algorithms and the high rate of exclusion weakens the real impact of such algorithms in the clinical decision making process (4).
Cost-effectiveness
In the SAPS study (36) the direct reduction in antibiotic cost using a PCT-guided algorithm was a mean of €34 per patient. Taking a mean of seven PCT measurements per patients implies that the direct reduction in antibiotic cost will only outweigh the cost of PCT measurements if a PCT test costs nearly €4, which is much less than the actual cost. Though, a PCT-guidance strategy is supposed to offer more important benefits and cost savings than only direct reduction of antibiotic costs.
Deliberato et al. (41) tested the clinical and economic impact of a PCT algorithm to shorten antibiotic therapy in critical care septic patients. In the per-protocol analysis they found a significant reduction of duration of antibiotic therapy (median days: 9, 5–24 in PCT group versus 13, 3–45, in the control group, P=0.008) and a net saving of USD388.25 per patient (roughly 30%) in the PCT group.
Westwood et al. (49) assessed clinical and cost-effectiveness of PCT testing to guide antibiotic therapy in patients with sepsis in ICU and emergency departments. Analysed data suggested that PCT testing was cost-saving for adults with sepsis in an ICU setting and for adults with suspected bacterial infection presenting to an emergency department—cost-savings from £368 to £3,268 with a small quality-adjusted life-year (QALY) gain. The PCT-guided approach had a probability of more than 84% of being cost-effective for all settings and populations considered (at a willingness-to-pay threshold of £20.000 and £30.000 per QALY).
CRP testing in primary care patients with respiratory tract infections proved to be cost-effective (20,21)—at a willingness-to-pay threshold of €30.000 per QALY gained, there was a 70% probability of CRP testing being cost-effective (20).
Biomarkers of potential interest in the future
The search for new biomarkers useful for guiding antibiotic therapy has produced some promising candidates. So far none of them have demonstrated to possess the properties described in Table 1 and none have been studied in a sufficient number of patients to merit further consideration. The most promising new biomarkers are briefly described in Table 3.
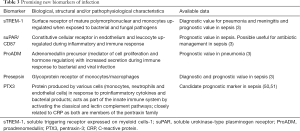
Full table
In a medium to long term it is credible to expect the availability of point-of-care rapid testing at bedside for current available biomarkers and the development of new methods of analysis (genomics, proteomics) that could allow identifying new biomarkers for better diagnosis, stratification of prognosis and tailoring antibiotic therapy in patients with infection.
Conclusions
Every antibiotic stewardship strategy recognises that antibiotics are cornerstones in the treatment of infection but acknowledges that its misuse and overuse in ICU patients is associated with increased duration of mechanical ventilation, length of stay, mortality, recurrence of infection, direct toxicity, organ failure, emergence of bacterial resistance and costs (52). Biomarkers cannot be expected to become isolated “magic bullets” with the ability of objectively distinguish patients who will benefit from starting or maintaining antibiotics. They are useful tools that help clinicians in different settings to optimize antibiotic therapy. Evidence is strong regarding the association of biomarker-guided strategies with a reduction in antibiotic exposure without adverse clinical outcomes. In order to empower such evidence and to deeply understand its potential effect on mortality it is essential in future RCTs to expand patients’ selection criteria to better reflect reality (52): clinicians have to deal with patients of increasing complexity (particularly in ICU setting) with a variety of likely infection foci—some of them not included in the available studies (25). Moreover, the true value of biomarkers in reducing antibiotic exposure must be tested against the best available evidence, which clearly evolves to shorter antibiotic courses, and not against a longer (more than 7–8 days) standard care strategy. Although PCT is certainly the most studied biomarker on antibiotic stewardship, combining information collected from several biomarkers (23) into clinical algorithms (52) may be more useful and helpful at bedside.
Neither the initiation nor the duration of antibiotic therapy can depend solely on a biomarker. Antibiotic management should reflect the complexity of infection and must be based on a composite of clinical evolution (namely organ function), microbiological data, source of infection, duration of antibiotic therapy and time course of biomarker levels.
Acknowledgements
None.
Footnote
Conflicts of Interest: The authors have no conflicts of interest to declare.
References
- Zilahi G, Artigas A, Loeches IM. What’ s new in multidrug - resistant pathogens in the ICU? Ann Intensive Care 2016;6:96. [Crossref] [PubMed]
- Michael CA, Dominey-Howes D, Labbate M. The antimicrobial resistance crisis: causes, consequences, and management. Front Public Health 2014;2:145. [Crossref] [PubMed]
- Dupuy AM, Philippart F, Péan Y, et al. Role of biomarkers in the management of antibiotic therapy: an expert panel review: I – currently available biomarkers for clinical use in acute infections. Ann Intensive Care 2013;3:22. [Crossref] [PubMed]
- Póvoa P, Salluh JIF. Biomarker-guided antibiotic therapy in adult critically ill patients: a critical review. Ann Intensive Care 2012;2:32. [Crossref] [PubMed]
- Biomarkers Definitions Working Group. Biomarkers and surrogate endpoints: Preferred definitions and conceptual framework. Clin Pharmacol Ther 2001;69:89-95. [Crossref] [PubMed]
- Póvoa P, Salluh JIF. Use of biomarkers in sepsis: Many questions, few answers. Rev Bras Ter Intensiva 2013;25:1-2. [PubMed]
- Quenot JP, Luyt CE, Roche N, et al. Role of biomarkers in the management of antibiotic therapy: an expert panel review II: clinical use of biomarkers for initiation or discontinuation of antibiotic therapy. Ann Intensive Care 2013;3:21. [Crossref] [PubMed]
- Markanday A. Acute Phase Reactants in Infections: Evidence- Based Review and a Guide for Clinicians Anurag. Open Forum Infect Dis 2015;2:ofv098. [Crossref] [PubMed]
- Cho S-Y, Choi J-HJ. Biomarkers of sepsis. Infect Chemother 2014;46:1-12. [Crossref] [PubMed]
- van der Does Y, Limper M, Schuit SCE, et al. Higher diagnostic accuracy and cost-effectiveness using procalcitonin in the treatment of emergency medicine patients with fever (The HiTEMP study): a multicenter randomized study. BMC Emerg Med 2016;16:17. [Crossref] [PubMed]
- Downes KJ, Weiss SL, Gerber JS, et al. A Pragmatic Biomarker-Driven Algorithm to Guide Antibiotic Use in the Pediatric Intensive Care Unit: The Optimizing Antibiotic Strategies in Sepsis (OASIS) Study. J Pediatric Infect Dis Soc 2016. [Epub ahead of print]. [Crossref] [PubMed]
- Ehl S, Gering B, Bartmann P, et al. C-Reactive Protein Is a Useful Marker for Guiding Duration of Antibiotic Therapy in Suspected Neonatal Bacterial Infection. Pediatrics 1997;99:216-21. [Crossref] [PubMed]
- Bomela HN, Ballot DE, Cory BJ, et al. Use of C-reactive protein to guide duration of empiric antibiotic therapy in suspected early neonatal sepsis. Pediatr Infect Dis J 2000;19:531-5. [Crossref] [PubMed]
- Huddy JR, Ni MZ, Barlow J, et al. Point-of-care C reactive protein for the diagnosis of lower respiratory tract infection in NHS primary care: a qualitative study of barriers and facilitators to adoption. BMJ Open 2016;6:e009959. [Crossref] [PubMed]
- Cals JWL, Butler CC, Hopstaken RM, et al. Effect of point of care testing for C reactive protein and. BMJ 2009;338:b1374. [Crossref] [PubMed]
- Strykowski DF, Nielsena ABS, Llorb C, et al. An intervention with access to C-reactive protein rapid test reduces antibiotic overprescribing in acute exacerbations of chronic bronchitis and COPD. Fam Pract 2015;32:395-400. [PubMed]
- Huang Y, Chen R, Wu T, et al. Association between point-of-care CRP testing and antibiotic prescribing in respiratory tract infections: A systematic review and meta-analysis of primary care studies. Br J Gen Pract 2013;63:e787-94. [Crossref] [PubMed]
- Little P, Stuart B, Francis N, et al. Effects of internet-based training on antibiotic prescribing rates for acute respiratory-tract infections: A multinational, cluster, randomised, factorial, controlled trial. Lancet 2013;382:1175-82. [Crossref] [PubMed]
- Aabenhus R, Jensen JU, Jørgensen KJ, et al. Biomarkers as point-of-care tests to guide prescription of antibiotics in patients with acute respiratory infections in primary care. Cochrane Database Syst Rev 2014.CD010130. [PubMed]
- Oppong R, Jit M, Smith RD, et al. Cost-effectiveness of point-of-care C-reactive protein testing to inform antibiotic prescribing decisions. Br J Gen Pract 2013;63:e465-71. [Crossref] [PubMed]
- Hunter R. Cost-Effectiveness of Point-of-Care C-Reactive Protein Tests for Respiratory Tract Infection in Primary Care in England. Adv Ther 2015;32:69-85. [Crossref] [PubMed]
- Kiaei BA, Ghiasi F, Moradi D. Precalcitonin and C-reactive protein as markers in response to antibiotic treatment in ventilator-associated pneumonia in intensive care unit-hospitalized patients. Adv Biomed Res 2015;4:240. [PubMed]
- Oliveira CF, Botoni FA, Oliveira CR, et al. Procalcitonin versus C-reactive protein for guiding antibiotic therapy in sepsis: a randomized trial. Crit Care Med 2013;41:2336-43. [Crossref] [PubMed]
- Christ-Crain M, Opal SM. Clinical review: the role of biomarkers in the diagnosis and management of community-acquired pneumonia. Crit Care 2010;14:203. [Crossref] [PubMed]
- Sager R, Kutz A, Mueller B, et al. Procalcitonin-guided diagnosis and antibiotic stewardship revisited. BMC Med 2017;15:15. [Crossref] [PubMed]
- Schuetz P, Albrich W, Mueller B. Procalcitonin for diagnosis of infection and guide to antibiotic decisions: past, present and future. BMC Med 2011;9:107. [Crossref] [PubMed]
- Schuetz P, Albrich W, Christ-Crain M, et al. Procalcitonin for guidance of antibiotic therapy. Expert Rev Anti Infect Ther 2010;8:575-87. [Crossref] [PubMed]
- Schuetz P, Chiappa V, Briel M, et al. Procalcitonin Algorithms for Antibiotic Therapy Decisions. Arch Intern Med 2011;171:1322-31. [Crossref] [PubMed]
- Schuetz P, Müller B, Stolz D, et al. Procalcitonin to initiate or discontinue antibiotics in acute respiratory tract infections. Cochrane Database Syst Rev 2012;9:CD007498. [PubMed]
- Lin C, Pang Q. Meta-analysis and systematic review of procalcitonin-guided treatment in acute exacerbation of chronic obstructive pulmonary disease. Clin Respir J 2016. [Epub ahead of print]. [Crossref] [PubMed]
- Andriolo BN, Andriolo R, Salomão R, et al. Effectiveness and safety of procalcitonin evaluation for reducing mortality in adult patients with sepsis, severe sepsis and septic shock. Cochrane Database Syst Rev 2017.CD010959. [PubMed]
- Layios N, Lambermont B, Canivet J-L, et al. Procalcitonin usefulness for the initiation of antibiotic treatment in intensive care unit patients. Crit Care Med 2012;40:2304-9. [Crossref] [PubMed]
- Bouadma L, Luyt C, Tubach F, et al. Use of procalcitonin to reduce patients’ exposure to antibiotics in intensive care units (PRORATA trial): a multicentre randomised controlled trial. Lancet 2010;375:463-74. [Crossref] [PubMed]
- Jensen JU, Hein L, Lundgren B, et al. Procalcitonin-guided interventions against infections to increase early appropriate antibiotics and improve survival in the intensive care unit: a randomized trial. Crit Care Med 2011;39:2048-58. [Crossref] [PubMed]
- Jensen JU, Heslet L, Jensen TH, et al. Procalcitonin increase in early identification of critically ill patients at high risk of mortality. Crit Care Med 2006;34:2596-602. [Crossref] [PubMed]
- de Jong E, van Oers JA, Beishuizen A, et al. Efficacy and safety of procalcitonin guidance in reducing the duration of antibiotic treatment in critically ill patients: a randomised, controlled, open-label trial. Lancet Infect Dis 2016;16:819-27. [Crossref] [PubMed]
- Chastre J, Wolff M, Fagon JY, et al. Comparison of 8 vs 15 Days of Antibiotic Therapy for Ventilator-Associated Pneumonia in Adults - A Randomized Trial. JAMA 2003;290:2588-98. [Crossref] [PubMed]
- Pugh R, Grant C, Rpd C, et al. Short-course versus prolonged-course antibiotic therapy for hospital-acquired pneumonia in critically ill adults Cochrane Database Syst Rev 2015.CD007577. (Review). [PubMed]
- Shehabi Y, Sterba M, Garrett PM, et al. Procalcitonin Algorithm in Critically Ill Adults with Undifferentiated Infection or Suspected Sepsis A Randomized Controlled Trial. Am J Respir Crit Care Med 2014;190:1102-10. [Crossref] [PubMed]
- Annane D, Maxime V, Faller JP, et al. Procalcitonin levels to guide antibiotic therapy in adults with non-microbiologically proven apparent severe sepsis: a randomised controlled trial. BMJ Open 2013;3:e002186. [Crossref] [PubMed]
- Deliberato RO, Marra AR, Sanches PR, et al. Clinical and economic impact of procalcitonin to shorten antimicrobial therapy in septic patients with proven bacterial infection in an intensive care setting. Diagn Microbiol Infect Dis 2013;76:266-71. [Crossref] [PubMed]
- Hochreiter M, Köhler T, Schweiger AM, et al. Procalcitonin to guide duration of antibiotic therapy in intensive care patients : a randomized prospective controlled trial. Crit Care 2009;13:R83. [Crossref] [PubMed]
- Stolz D, Smyrnios N, Eggimann P, et al. Procalcitonin for reduced antibiotic exposure in ventilator-associated pneumonia: a randomised study. Eur Respir J 2009;34:1364-75. [Crossref] [PubMed]
- Schroeder S, Hochreiter M, Koehler T, et al. Procalcitonin (PCT)-guided algorithm reduces length of antibiotic treatment in surgical intensive care patients with severe sepsis: Results of a prospective randomized study. Langenbecks Arch Surg 2009;394:221-6. [Crossref] [PubMed]
- Nobre V, Harbarth S, Graf J-D, et al. Use of Procalcitonin to Shorten Antibiotic Treatment Duration in Septic Patients. Am J Respir Crit Care Med 2008;177:498-505. [Crossref] [PubMed]
- Svoboda P, Kantorová I, Scheer P, et al. Can procalcitonin help us in timing of re-intervention in septic patients after multiple trauma or major surgery? Hepatogastroenterology 2007;54:359-63. [PubMed]
- Rhodes A, Evans LE, Alhazzani W, et al. Surviving Sepsis Campaign: International Guidelines for Management of Sepsis and Septic Shock: 2016. Intensive Care Med 2017;43:304-77. [Crossref] [PubMed]
- Kalil AC, Metersky ML, Klompas M, et al. Management of Adults With Hospital-acquired and Ventilator-associated Pneumonia: 2016 Clinical Practice Guidelines by the Infectious Diseases Society of America and the American Thoracic Society. Clin Infect Dis 2016;63:e61-111. [Crossref] [PubMed]
- Westwood M, Ramaekers B, Whiting P, et al. Procalcitonin testing to guide antibiotic therapy for the treatment of sepsis in intensive care settings and for suspected bacterial infection in emergency department settings: a systematic review and cost-effectiveness analysis. Health Technol Assess 2015;19:v-xxv, 1-236. [Crossref] [PubMed]
- Canovi M, Lucchetti J, Stravalaci M, et al. A new surface plasmon resonance-based immunoassay for rapid, reproducible and sensitive quantification of pentraxin-3 in human plasma. Sensors 2014;14:10864-75. [Crossref] [PubMed]
- Hansen MB, Rasmussen LS, Garred P, et al. Pentraxin-3 as a marker of disease severity and risk of death in patients with necrotizing soft tissue infections: a nationwide, prospective, observational study. Crit Care 2016;20:40. [Crossref] [PubMed]
- Póvoa P, Salluh JIF, Nobre V, Martín-loeches I. Biomarker guided antibiotic therapy. What’s new? ICU Manag Pract 2016;(1):24-8.