Spontaneous breathing: a double-edged sword to handle with care
Introduction
Increased understanding of the negative effects of sedation and paralysis on the clinical outcome of mechanically ventilated patients (1,2) together with relevant technology advancements (3,4) recently led to larger emphasis on early switch to some form of assisted spontaneous ventilation. A recent large observational study (5) reported that around 30% of invasively ventilated patients with acute hypoxemic respiratory failure (AHRF) and acute respiratory distress syndrome (ARDS) breath spontaneously since day 1 from intubation, regardless from their severity, and this proportion tends to increase significantly within the first week of intubation. Indeed, on the one hand, spontaneous breathing is associated with multiple potential benefits on neuromuscular function, hemodynamics and lung function (6). On the other hand, seminal experimental studies from the 80’s (7) as well as more recent laboratory investigations (8) described potential harms of spontaneous breathing in the presence of: uncontrolled inspiratory effort, increased ventilation heterogeneity leading to occult pendelluft and cyclic opening and closing of small airways, and increased inspiratory resistance. In the present review, we present both the potential benefits and the risks of spontaneous breathing in mechanically ventilated patients, with a focus on physiology and clinical means to apply protective spontaneous breathing.
Physiology of spontaneous and assisted breathing
The whole goal of respiration is to allow air to enter the alveolar space, so that equilibration of alveolar gases with those in the blood flowing within peri-alveolar capillaries can take place. To enable arrival of tidal volume (Vt) from the atmosphere to the alveoli, a pressure difference is created across the respiratory system (i.e., between the mouth and the external surface of the chest wall). During unassisted spontaneous breathing, this pressure gradient is generated only by the work of respiratory muscles. During invasive or non-invasive ventilation, the mechanical ventilator represents a pressure generator arranged in series with the respiratory muscles and the work to generate the mouth-alveolar pressure gradient is shared by the muscles and the machine. Finally, during controlled ventilation, muscles are passive and the positive pressure generated by the ventilator drives the Vt to the alveolar space (9).
Whatever the clinical condition and ventilation mode, at any time, the total pressure across the respiratory system during mechanical ventilation is determined by the following equation:
Pao(t) + Pmus(t) = PEEP + [Ers × V(t)] + [Rrs × Flow(t)]
Where Pao is the pressure at the airway opening, Pmus is the pressure generated by respiratory muscles, PEEP is positive end-expiratory pressure, Ers is the respiratory system elastance, V is the Vt, Rrs is the resistance of the respiratory system and Flow is the airflow.
Thus, along inspiration, total pressure applied to the respiratory system is always proportional to the starting pressure within the airway (i.e., PEEP), to the elastic recoil of the respiratory system that opposes expansion by the Vt [i.e., Ers × V(t)] and to airway resistance against the inspiratory airflow [i.e., Rrs × Flow(t)]. During unassisted spontaneous breathing [e.g., during continuous positive airway pressure (CPAP)], along inspiration, Pao remains almost unchanged, and, hence, PEEP + Pmus is the corresponding total pressure across the respiratory system. When some form of positive pressure generated by the ventilator is added to spontaneous breathing [e.g., when pressure support ventilation (PSV) is added], Pao increases, equaling to the support set by the attending physician, yielding and increased total pressure across the respiratory system with the generation of higher flows and therefore larger Vt.
It now becomes evident that, during spontaneous and assisted breathing, the mechanisms underlying the dynamics of tidal ventilation are pressure application and volume expansion, similarly to passive mechanical ventilation. Thus, one cannot forget that, in patients switched to assisted ventilation, the main determinants of ventilator-induced lung injury (VILI), such as barotrauma and volutrauma (10), are still at play and should be carefully monitored and minimized. Moreover, while the pressure gradient across the respiratory system is always positive during assisted spontaneous ventilation, we must notice that the absolute pressure inside the alveoli (Palv), instead, follows different dynamics. Without external support from the ventilator, Palv decreases below PEEP to generate positive Pao—Palv gradient, while, during PSV, Palv is smaller than PEEP for a limited amount of the inspiratory time that increases with Pmus (11). Thus, during spontaneous breathing, the difference between intravascular capillary pressure and Palv increases in comparison to controlled ventilation, theoretically posing diseased alveoli with large surface tension at risk for transmural vascular fluid exudation and collapse.
Moreover, during assisted ventilation modes, the work of breathing is shared between the ventilator and the patient but the inspiratory time of the two might not be perfectly matched. Thus, if the ventilator’s positive pressure persists after the end of patient’s inspiration, Vt insufflation into the alveolar space switches from shared hybrid to totally passive mechanism (i.e., Pao >PEEP with Pmusc zero); instead, if the ventilator cycles to expiration before the end of positive Pmus swing, patient-ventilator asynchrony, namely double-triggering and/or early cycling, appears.
Assisted mechanical ventilation modes, i.e., ventilation modes integrating spontaneous and mechanical respiratory activity, clearly represent a way to bring a life-saving medical treatment nearer to physiology. Nevertheless, application of elevated pressures to the respiratory system, large swings in Palv and resulting asynchronies represent serious threats potentially limiting the benefits of assisted breathing.
Respiratory monitoring during spontaneous breathing
Usually, the only pressure monitored during mechanical ventilation is the airway pressure (Pao, see above), which represents the pressure difference between the patient’s airway and the atmosphere (conventionally referred to as zero pressure). However, Pao represents the total pressure across the respiratory system only in completely passive patients. Indeed, during active inspiration, Pmus is summed to Pao to generate the inspiratory airflow, and Pmus should be included in the careful clinical monitoring of active mechanically ventilated patients. Pmus represents the pressure difference between the pressure generated by the relaxed chest wall and the change in pleural pressure (Ppl) at given lung volume. In clinical practice, esophageal pressure (Pes) can be used as surrogate measure of Ppl and the elastic recoil of the chest wall [i.e., the chest wall elastance (Ew)] can be measured by switching the patient to controlled ventilation and dividing the change in Pes by Vt. Individual Ew can also be calculated as 4% of predicted vital capacity (12). Thus, if an esophageal balloon is in place, Pmus can be calculated (and monitored) at the bedside, at any time t, as:
Pmus = V(t) × Ew − ∆Pes(t)
where V is the tidal volume, Ew is the chest wall elastance and ∆Pes is the inspiratory Pes change from baseline.
Bedside quantification of Pmus allows quantification of total pressure difference across the respiratory system and it is also an estimate of patient’s inspiratory effort, representing a simple target to set assisted ventilation and avoid under- and over-assistance. The pressure generated during the first 100 milliseconds against an occluded airway (P0.1) has been suggested as surrogate to evaluate patient’s effort in the absence of Pes monitoring (12).
Recent studies also underlined the relevance of airway driving pressure (i.e., ∆Pao = plateau − total PEEP) for the outcome of mechanically ventilated ARDS patients (13). Driving pressure might represent a more specific monitoring of the strain applied to the lung by tidal ventilation. However, monitoring of ∆Pao is not feasible during assisted spontaneous breathing. In spontaneously breathing mechanically ventilated patients with Pes monitoring in place, the difference between Pao and absolute Pes at the end of inspiration (zero flow) represents the driving transpulmonary pressure (∆PL) (Figure 1). As ∆PL represents the pressure across lung parenchyma due to tidal ventilation, it might be regarded as a more specific marker of the risk of barotrauma and VILI than ∆Pao in mechanically ventilated patients. Pilot clinical data also showed that ∆PL might be a more relevant determinant of the clinical outcome of ARDS patients than ∆Pao. Thus, ∆PL might represent a clinically feasible bedside monitoring of the safety of protective assisted ventilation (12).
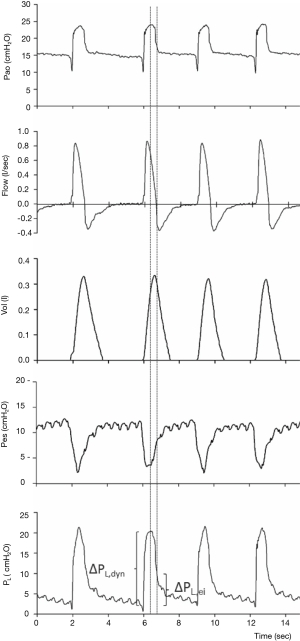
Finally, bedside Pes monitoring might be regarded as the gold standard to accurately quantify patient-ventilator asynchronies (12).
Benefits of spontaneous and assisted breathing: experimental, physiological and clinical evidences
It is often assumed that environmental oxygen concentrations increased to sufficient levels for animal respiration during the Neoproterozoic era. Our respiratory system has evolved, after millions of years of selective evolutionary pressure, to a surprisingly efficient gas exchanger characterized by thin blood-gas barrier, large interface, ventilatory regulation, and low cost of breathing (14). Of course, animals have, for these millions of years, breathed spontaneously. Indeed, spontaneous was an unnecessary adjective to the word breathing until the era of mechanical ventilation began in the mid of the 20th century, during the Copenhagen Poliomyelitis epidemic (15).
These zoological and historical premises explain why spontaneous breathing, resulting from millions of years of steady improvement, is undoubtedly better, in physiological conditions, as compared to mechanical ventilation, with its extremely short and modest evolutionary story. On the contrary, there are still many unresolved controversies about advantages and disadvantages of maintaining spontaneous breathing in critically-ill patients with respiratory failure (16-19) (i.e., patients with deranged respiratory physiology and still incompletely understood respiratory pathophysiology). Of note, in these patients, at least in the very acute phase, controlled mechanical ventilation is still the gold standard. Nevertheless, early switch to assisted ventilation/spontaneous breathing is gaining popularity in the critical care setting. When translating physiologic concepts to clinical practice, one could argue that spontaneous/assisted breathing would have several advantages as compared to controlled mechanical ventilation. First, the tone of the respiratory muscles in the spontaneously breathing subject, guarantees the expansion of chest-wall and lungs at end expiration (functional residual capacity). Furthermore, recent studies, suggest that the diaphragm might contract during expiration, thus preserving distal airway patency and avoiding/reducing expiratory atelectasis formation (20). As a result, preserving diaphragmatic activity, besides reducing the risk of ventilator-induced diaphragm dysfunction (21), could increase lung aeration as compared to muscle paralysis and mechanical ventilation. Second, the spontaneously breathing patient moves preferentially the dorsal and more compliant part of the diaphragm leading to an optimal ventilation-perfusion matching, and therefore improvement in gas exchange, and potentially reducing hyperinflation of the non-dependent lung regions (Figure 2). Third, spontaneous breathing leads to a negative swing in intrathoracic pressure, to allow movement of air from the atmosphere, through the airways and to the lungs. This intrathoracic pressure variation favors cardiac filling and output as it promotes venous return from extra-thoracic organs (22). Finally, the clinical choice of an assisted/spontaneous ventilation usually coincides with the prescription of less sedatives, thus reducing the burden of associated side-effects (23). Benefits of spontaneous breathing are summarized in Table 1.
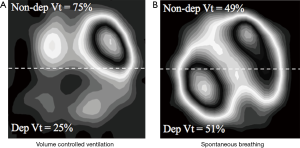
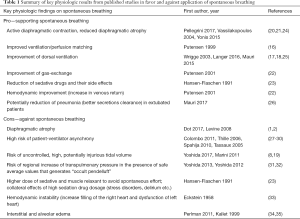
Full table
Risks of spontaneous and assisted breathing: experimental, physiological and clinical evidences
Despite playing a role in ameliorating oxygenation and diaphragmatic tone, spontaneous breathing effort during mechanical ventilation has been proven to have also detrimental effects, especially in patients with severe forms of ARDS (36). This was also suggested by recent studies on neuromuscular blocking agents in early ARDS which improved survival, also by suppressing the spontaneous damaging effort (31,37).
Latest evidence on animal models shows that the triggering effort associated with spontaneous breathing may worsen lung injury and that the mechanisms involved may be directly related to barotrauma (32,38). A study from Yoshida et al. on experimental model of lavage-injured rabbits demonstrated that, even keeping the airway plateau pressure (Pplat) within protective values, the increase in transpulmonary pressure (PL) in case of spontaneous effort, can worsen lung injury if combined with increase in respiratory rate and Vt. This is in part explained by the so-called “occult pendelluft”, which is the movement of gas from non-dependent regions to dependent regions during inspiration that dramatically increases regional distension of already injured lung regions (39).
To better understand the mechanisms generating the regional lung stress, an overview on the PL is needed. The PL is the pressure of the airway (Paw) minus the pressure of the pleura (Ppl) (40). In healthy lungs, a spontaneous breathing cycle is characterized by essentially no change in Paw and a decrease in Ppl, which is normally approximated to the Pes (17,38). This pressure difference is responsible for an inflation which is uniform in the respiratory system and corresponds to the so-called “fluid like” behavior. In the case of lung damage, the parenchyma may present inhomogeneity and non-aerated regions with consolidation and/or parenchyma disruption. In this context, in case of spontaneous effort, additional lung damage might be directly associated with the increase of Vt derived from occult pendelluft moving gas from non-dependent aerated lung to collapsed dorsal regions, likely because of stronger regional diaphragm contraction.
In a spontaneous breath, the augmentation of PL causes direct increase of Vt. This happens in case of absence of pre-existing lung conditions. In case of restrictive or obstructive diseases, the effect of driving PL on the alveoli’s inflation is decreased due to the alveoli’s poor baseline condition. However, when PL crosses the opening alveolar pressure, the alveolus expands and re-collapses during expiration if the closing pressure is crossed back (32,39,40), causing cyclic opening and closing and additional injury. This is particularly true in the dorsal lung, where opening and closing pressure are higher and the effort more vigorous.
Additionally, the negative impact on the Ppl caused by the spontaneous breathing, is responsible for deep modifications on transmural vascular pressure which is normally increased. This led to distension of pulmonary vessels, augmentation in lung perfusion and finally edema (34). In ARDS patients, the capillary endothelium and the alveolar tissue express different degrees of damage. This causes leakage of protein-rich plasma into the alveolar space decreasing the osmotic gradient of proteins which normally opposes to the edema. Excessively high negative intrathoracic pressures swing raises the transmural vascular pressure gradient thus inducing hydrostatic pulmonary edema (33,35).
Another key point is the presence of patient-ventilator asynchronies (27). In case of spontaneous effort, the arousal of consecutives inspiration triggers, as also known as “double triggering” the Vt of the first breath sums up with the Vt of the second resulting in the delivery of non-protective Vt. The same injurious effect can be elicited by the ventilator itself in case of “reverse triggering” (12).
The effect of the ventilator on the patient diaphragm is partially responsible for causing an increase in PL which consequently increases the delivered Vt, thus potentially causing additional edema and damage.
Recently Yoshida et al. demonstrated in animal study and in one patient with ARDS that volume controlled ventilation with fixed ∆PL may not be able to prevent lung injury in case of strenuous spontaneous effort (8). Even the limitation of Vt could not prevent the injury when the patient trigger the ventilator. Hence, atelectatic dependent lung is oversensitive to local stress directly caused by a “solid-like” behavior of the lung during the respiratory effort that inhomogeneously increases Ppl especially in the dependent regions. Moreover, the Pes swing can underestimate this phenomenon
Actual mechanisms increasing the risk of VILI during assisted spontaneous breathing (e.g., occult pendelluft and solid-like lung behavior) are extremely difficult to recognize at the bedside but, clinically, they should be suspected in patients with more severe lung injury (e.g., patients with extremely low compliance) and/or with strenuous inspiratory effort (41).
How to promote protective spontaneous breathing in the clinical setting
To promote protective spontaneous assisted breathing, it is essential to optimize the interaction between the patient and the ventilator. This can be obtained by improving patient-ventilator synchrony and controlling the patient’s respiratory drive. These goals can be extremely challenging to achieve, especially in “difficult” cases like patients recovering from severe ARDS, patients with chronic obstructive pulmonary disease (COPD) and dynamic hyperinflation or patients with a very high respiratory drive from any cause.
Particularly in these situations, it is extremely important to monitor the output of the neural respiratory centers and the activity of the respiratory muscles: this can be done using an esophageal balloon to measure Pes swings (17,26) or a nasogastric catheter equipped with microelectrodes to detect the diaphragm electromyography (EAdi) (42). Compared to the simple observation of respiratory pattern (Vt and respiratory rate) and ventilator waveforms, these instruments significantly improve the detection of asynchronies (27,43); in addition, they allow monitoring of the patient’s respiratory drive and effort (see below).
Several studies have demonstrated that a high incidence of asynchronies is associated with adverse clinical outcomes, such as increased duration of mechanical ventilation (28). Hence, it is very important to optimize patient-ventilator synchrony in terms of:
- Timing of assist: during pneumatically-triggered modes, in patients with intrinsic PEEP it is common to observe ineffective efforts and prolonged inspiratory triggered delay, which significantly increase the respiratory workload (29). Quite common are also cycling-off asynchronies, occurring when the switch to expiration is not synchronized with the patient’s neural inspiratory time (30): in patients with low respiratory system compliance, early cycling and double triggering are commonly observed since the cycling-off criteria are reached when the patient is still trying to inspire. On the contrary, patients with highly compliant lungs frequently experience late cycling and inappropriately high inspiratory time. During PSV, timing asynchronies can be, at least in part, controlled by carefully adjusting the inspiratory and expiratory trigger sensitivity and the inspiratory pressure time rise. In difficult patients with high incidence of asynchronies, switching from PSV to neurally adjusted ventilator assist (NAVA) may be indicated. NAVA is a new mode of assisted ventilation during which the ventilator assist is proportional (in terms of both timing and magnitude of assist) to the EAdi signal (42). Several studies have demonstrated that during NAVA the incidence of asynchronies is significantly reduced and patient-ventilator interaction improved (3,24).
- Magnitude of assist: it is important to avoid both over-assistance (i.e., inspiratory assist too high), which is associated with progressive disuse atrophy of the diaphragm, and under-assistance (i.e., inspiratory assist too low), which inevitably leads to respiratory muscles fatigue. Hence, it is crucial to carefully monitor the pressure developed by the patient’s respiratory muscles (Pmus) and to adjust accordingly the level of assist: this can be done directly by means of an esophageal balloon or indirectly by monitoring the PMI (validated during PSV) (25) or the PEI index (derived from the EAdi signal during an inspiratory effort against an occluded airway) (44).
Control of the patient’s respiratory drive is another crucial aspect. As discussed in the previous paragraph, patients with very high respiratory drive have a significant risk of developing excessive transpulmonary pressure swings during assisted spontaneous breathing. Hence, monitoring patient drive and effort is mandatory: as stated above, this can be achieved by monitoring Pes swings or EAdi signal. Another parameter very simple to obtain and closely related to the patient’s drive and work of breathing is P0.1: this is the airway pressure drop during the first 100 msec of an inspiratory effort against an occluded airway and most modern intensive care unit ventilators have a dedicated function to measure it. Several strategies can be implemented to modulate the patient’s drive and allow maintenance of protective spontaneous breathing. First, during assisted spontaneous breathing it is very difficult to accept “permissive” hypoxemia and/or hypercapnia: in other words, we should aim to more physiological targets of PaO2 and PaCO2 than those accepted during controlled ventilation. Second, it is very important to treat fever and pain, which lead to significant increase of O2 consumption (VO2) and CO2 production (VCO2) and, consequently, of the ventilation load. Similarly, it is very important to find an adequate sedation plan, to avoid anxiety, delirium and agitation that are associated to important increase in the metabolic demand. A more complex and advanced approach to respiratory drive control is represented by extracorporeal CO2 removal. There is a linear relationship between VCO2 and minute ventilation: increasing the amount of CO2 removed by the extracorporeal circuit should result in a proportional decrease of minute ventilation and inspiratory effort (45,46). In 8 patients on ECMO recovering from ARDS, Mauri et al. demonstrated that increasing extracorporeal CO2 extraction led to linear reduction of EAdi, Vt, inspiratory Pmusc and transpulmonary pressure (47). However, the underlying lung pathology may influence the relationship between CO2 removal and respiratory drive: especially in the early phases of ARDS it can be extremely difficult to control the patient’s drive and effort, even at very high levels of extracorporeal CO2 removal (46). Recently, Crotti et al. studied the response to increasing levels of extracorporeal CO2 removal in 23 patients on ECMO for different reasons (bridge to lung transplant, COPD exacerbation and ARDS) (48). They observed that extracorporeal CO2 removal could control work of breathing allowing extubation of all patients bridged to lung transplant and with COPD, while in half of ARDS patients, removal of large amounts of CO2 was not sufficient to prevent potentially harmful inspiratory efforts. An alternative but still experimental approach to facilitate lung protection during assisted spontaneous breathing has been recently proposed by Doorduin et al.: in 10 sedated patients with acute lung injury developing Vt >8 mL/kg during PSV, sub-therapeutic doses of rocuronium were administered to obtain a “partial neuromuscular blockade” (49). This led to significant reduction of Vt, EAdi and transpulmonary pressure, without major effect on arterial pH and diaphragm activity.
Conclusions
Spontaneous breathing means physiologic breathing. However, risks of developing additional alveolar edema and lung injury are not completely avoided by application of spontaneous ventilation. During spontaneous assisted ventilation modes, careful bedside monitoring of Vt, respiratory rate, pressure across respiratory structures, patient’s effort and asynchronies, also by using Pes, might be key to fully exert their beneficial effects.
Acknowledgements
This work was supported by Departmental funding and the Italian Ministry of University and Research (BC).
Footnote
Conflicts of Interest: The authors have no conflicts of interest to declare.
References
- Dot I, Pérez-Teran P, Samper MA, et al. Diaphragm Dysfunction in Mechanically Ventilated Patients. Arch Bronconeumol 2017;53:150-6. [PubMed]
- Levine S, Nguyen T, Taylor N, et al. Rapid disuse atrophy of diaphragm fibers in mechanically ventilated humans. N Engl J Med 2008;358:1327-35. [PubMed]
- Demoule A, Clavel M, Rolland-Debord C, et al. Neurally adjusted ventilatory assist as an alternative to pressure support ventilation in adults: a French multicentre randomized trial. Intensive Care Med 2016;42:1723-32. [PubMed]
- Rittayamai N, Brochard L. Recent advances in mechanical ventilation in patients with acute respiratory distress syndrome. Eur Respir Rev 2015;24:132-40. [PubMed]
- Bellani G, Laffey JG, Pham T, et al. Epidemiology, Patterns of Care, and Mortality for Patients With Acute Respiratory Distress Syndrome in Intensive Care Units in 50 Countries. JAMA 2016;315:788-800. [PubMed]
- Yoshida T, Fujino Y, Amato MB, et al. Fifty Years of Research in ARDS. Spontaneous Breathing during Mechanical Ventilation. Risks, Mechanisms, and Management. Am J Respir Crit Care Med 2017;195:985-92. [PubMed]
- Mascheroni D, Kolobow T, Fumagalli R, et al. Acute respiratory failure following pharmacologically induced hyperventilation: an experimental animal study. Intensive Care Med 1988;15:8-14. [PubMed]
- Yoshida T, Nakahashi S, Nakamura MA, et al. Volume Controlled Ventilation Does Not Prevent Injurious Inflation During Spontaneous Effort. Am J Respir Crit Care Med 2017. [Epub ahead of print]. [PubMed]
- Mauri T, Guérin C, Hubmayr R. The ten pressures of the respiratory system during assisted breathing. Intensive Care Med 2017. [Epub ahead of print]. [PubMed]
- Brochard L, Slutsky A, Pesenti A. Mechanical Ventilation to Minimize Progression of Lung Injury in Acute Respiratory Failure. Am J Respir Crit Care Med 2017;195:438-42. [PubMed]
- Bellani G, Grasselli G, Teggia-Droghi M, et al. Do spontaneous and mechanical breathing have similar effects on average transpulmonary and alveolar pressure? A clinical crossover study. Crit Care 2016;20:142. [PubMed]
- Mauri T, Yoshida T, Bellani G, et al. Esophageal and transpulmonary pressure in the clinical setting: meaning, usefulness and perspectives. Intensive Care Med 2016;42:1360-73. [PubMed]
- Laffey JG, Bellani G, Pham T, et al. Potentially modifiable factors contributing to outcome from acute respiratory distress syndrome: the LUNG SAFE study. Intensive Care Med 2016;42:1865-76. [PubMed]
- Hsia CC, Schmitz A, Lambertz M, et al. Evolution of air breathing: oxygen homeostasis and the transitions from water to land and sky. Compr Physiol 2013;3:849-915. [PubMed]
- West JB. The physiological challenges of the 1952 Copenhagen poliomyelitis epidemic and a renaissance in clinical respiratory physiology. J Appl Physiol (1985) 2005;99:424-32. [PubMed]
- Putensen C, Mutz NJ, Putensen-Himmer G, et al. Spontaneous breathing during ventilatory support improves ventilation-perfusion distributions in patients with acute respiratory distress syndrome. Am J Respir Crit Care Med 1999;159:1241-8. [PubMed]
- Wrigge H, Zinserling J, Neumann P, et al. Spontaneous breathing improves lung aeration in oleic acid-induced lung injury. Anesthesiology 2003;99:376-84. [PubMed]
- Langer T, Santini A, Bottino N, et al. “Awake” extracorporeal membrane oxygenation (ECMO): pathophysiology, technical considerations, and clinical pioneering. Crit Care 2016;20:150. [PubMed]
- Marini JJ. Spontaneously regulated vs. controlled ventilation of acute lung injury/acute respiratory distress syndrome. Curr Opin Crit Care 2011;17:24-9. [PubMed]
- Pellegrini M, Hedenstierna G, Roneus A, et al. The Diaphragm Acts as a Brake During Expiration to Prevent Lung Collapse. Am J Respir Crit Care Med 2017;195:1608-16. [PubMed]
- Vassilakopoulos T, Petrof BJ. Ventilator-induced diaphragmatic dysfunction. Am J Respir Crit Care Med 2004;169:336-41. [PubMed]
- Putensen C, Zech S, Wrigge H, et al. Long-term effects of spontaneous breathing during ventilatory support in patients with acute lung injury. Am J Respir Crit Care Med 2001;164:43-9. [PubMed]
- Hansen-Flaschen JH, Brazinsky S, Basile C, et al. Use of sedating drugs and neuromuscular blocking agents in patients requiring mechanical ventilation for respiratory failure. A national survey. JAMA 1991;266:2870-5. [PubMed]
- Yonis H, Crognier L, Conil JM, et al. Patient-ventilator synchrony in Neurally Adjusted Ventilatory Assist (NAVA) and Pressure Support Ventilation (PSV): a prospective observational study. BMC Anesthesiol 2015;15:117. [PubMed]
- Mauri T, Eronia N, Abbruzzese C, et al. Effects of Sigh on Regional Lung Strain and Ventilation Heterogeneity in Acute Respiratory Failure Patients Undergoing Assisted Mechanical Ventilation. Crit Care Med 2015;43:1823-31. [PubMed]
- Mauri T, Turrini C, Eronia N, et al. Physiologic Effects of High-Flow Nasal Cannula in Acute Hypoxemic Respiratory Failure. Am J Respir Crit Care Med 2017;195:1207-15. [PubMed]
- Colombo D, Cammarota G, Alemani M, et al. Efficacy of ventilator waveforms observation in detecting patient-ventilator asynchrony. Crit Care Med 2011;39:2452-7. [PubMed]
- Thille AW, Rodriguez P, Cabello B, et al. Patient-ventilator asynchrony during assisted mechanical ventilation. Intensive Care Med 2006;32:1515-22. [PubMed]
- Spahija J, de Marchie M, Albert M, et al. Patient-ventilator interaction during pressure support ventilation and neurally adjusted ventilatory assist. Crit Care Med 2010;38:518-26. [PubMed]
- Tassaux D, Gainnier M, Battisti A, et al. Impact of expiratory trigger setting on delayed cycling and inspiratory muscle workload. Am J Respir Crit Care Med 2005;172:1283-9. [PubMed]
- Yoshida T, Uchiyama A, Matsuura N, et al. The comparison of spontaneous breathing and muscle paralysis in two different severities of experimental lung injury. Crit Care Med 2013;41:536-45. [PubMed]
- Yoshida T, Uchiyama A, Matsuura N, et al. Spontaneous breathing during lung-protective ventilation in an experimental acute lung injury model: high transpulmonary pressure associated with strong spontaneous breathing effort may worsen lung injury. Crit Care Med 2012;40:1578-85. [PubMed]
- Eckstein JW, Hamilton WK. Changes in transmural central venous pressure in man during hyperventilation. J Clin Invest 1958;37:1537-41. [PubMed]
- Perlman CE, Lederer DJ, Bhattacharya J. Micromechanics of alveolar edema. Am J Respir Cell Mol Biol 2011;44:34-9. [PubMed]
- Kallet RH, Alonso JA, Luce JM, et al. Exacerbation of acute pulmonary edema during assisted mechanical ventilation using a low-tidal volume, lung-protective ventilator strategy. Chest 1999;116:1826-32. [PubMed]
- Takeuchi M, Tachibana K. Mechanical ventilation for ARDS patients--for a better understanding of the 2012 Surviving Sepsis Campaign Guidelines. Cardiovasc Hematol Disord Drug Targets 2015;15:41-5. [PubMed]
- Gainnier M, Roch A, Forel JM, et al. Effect of neuromuscular blocking agents on gas exchange in patients presenting with acute respiratory distress syndrome. Crit Care Med 2004;32:113-9. [PubMed]
- Yoshida T, Torsani V, Gomes S, et al. Spontaneous effort causes occult pendelluft during mechanical ventilation. Am J Respir Crit Care Med 2013;188:1420-7. [PubMed]
- Yoshida T, Uchiyama A, Fujino Y. The role of spontaneous effort during mechanical ventilation: normal lung versus injured lung. J Intensive Care 2015;3:18. [PubMed]
- Güldner A, Pelosi P, Gama de Abreu M. Spontaneous breathing in mild and moderate versus severe acute respiratory distress syndrome. Curr Opin Crit Care 2014;20:69-76. [PubMed]
- Mauri T, Bellani G, Grasselli G, et al. Patient-ventilator interaction in ARDS patients with extremely low compliance undergoing ECMO: a novel approach based on diaphragm electrical activity. Intensive Care Med 2013;39:282-91. [PubMed]
- Sinderby C, Navalesi P, Beck J, et al. Neural control of mechanical ventilation in respiratory failure. Nat Med 1999;5:1433-6. [PubMed]
- Sinderby C, Liu S, Colombo D, et al. An automated and standardized neural index to quantify patient-ventilator interaction. Crit Care 2013;17:R239. [PubMed]
- Bellani G, Mauri T, Coppadoro A, et al. Estimation of patient's inspiratory effort from the electrical activity of the diaphragm. Crit Care Med 2013;41:1483-91. [PubMed]
- Kolobow T, Gattinoni L, Tomlinson TA, et al. Control of breathing using an extracorporeal membrane lung. Anesthesiology 1977;46:138-41. [PubMed]
- Langer T, Vecchi V, Belenkiy SM, et al. Extracorporeal gas exchange and spontaneous breathing for the treatment of acute respiratory distress syndrome: an alternative to mechanical ventilation?*. Crit Care Med 2014;42:e211-20. [PubMed]
- Mauri T, Grasselli G, Suriano G, et al. Control of Respiratory Drive and Effort in Extracorporeal Membrane Oxygenation Patients Recovering from Severe Acute Respiratory Distress Syndrome. Anesthesiology 2016;125:159-67. [PubMed]
- Crotti S, Bottino N, Ruggeri GM, et al. Spontaneous Breathing during Extracorporeal Membrane Oxygenation in Acute Respiratory Failure. Anesthesiology 2017;126:678-87. [PubMed]
- Doorduin J, Nollet JL, Roesthuis LH, et al. Partial Neuromuscular Blockade during Partial Ventilatory Support in Sedated Patients with High Tidal Volumes. Am J Respir Crit Care Med 2017;195:1033-42. [PubMed]