Positive end-expiratory pressure: how to set it at the individual level
Introduction
Barach and colleagues in 1938 fully described the effects of positive end-expiratory pressure (PEEP) as an adjunct to mechanical ventilation for cardiogenic pulmonary edema, sepsis or asphyxia, in experimental animals and in seven patients with cardiac failure (1). Cournand and colleagues in 1946 measured the dismal effect on hemodynamics caused by the intermittent intrathoracic positive pressure (2). As logical consequence, PEEP was not implemented in clinical practice until the late 1960s, in order to avoid further impact on the hemodynamic.
In that period, Gregory and colleagues in San Francisco applied PEEP in spontaneous breathing neonates with respiratory distress due to surfactant deficit, without reporting major hemodynamic problems (3). In the same period was described the adult respiratory distress syndrome which, in analogy with the infants’ respiratory distress syndrome, was thought to be primarily due to surfactant deficit (4). The adult respiratory distress syndrome, later called acute respiratory distress syndrome (ARDS), became the prototype pathology accompanying the development of intensive care medicine (5) and PEEP was one of the most widely used therapeutic approaches. The first studies on mechanisms of PEEP during ARDS were performed by Falke and colleagues at the Massachusetts General Hospital (6), while the most popular clinical approach for PEEP setting was proposed by Suter as a function of oxygen transport and respiratory mechanics (7). In the meantime, Kirby and colleagues, from Miami, proposed the use of a “super PEEP”, that is PEEP up to 25 cmH2O, somehow forerunner of the “open lung approach” (8). Tenaillon, from the French side, proposed to increase PEEP up to whatever level was sufficient to decrease the venous admixture below 10% (9). Dantzker, revisiting the Cournand worries, claimed that the primary mechanism of PEEP in improving oxygenation was through a decrease of cardiac output (10). Indeed, venous admixture and cardiac output are linearly related, as firstly described by Lemaire et al. in that same period (11,12). It is interesting to note that, within the variety of approaches proposed, tested and discussed, two points were unquestioned: first, the use of PEEP was uniquely linked to the oxygenation; second, the hemodynamic effects were always taken into account.
A major breakthrough in the history of PEEP was the discovery that the inflammatory reaction, as measured by cytokines analysis, was prevented/dampened by the use of PEEP (13). Mead’s theoretical model provided the foundations for the atelectrauma theory (14), which was then illustrated by Lachmann (15) and clinically corroborated by Ranieri (16). Since the 90s, following a series of experimental observations that found PEEP effective in preventing huge lung edema, beginning with the Webb and Tierney findings (17), PEEP was not considered anymore a mere means to improve oxygenation, but a tool to protect the lung. The emphasis on lung protection led to a progressive oblivion of the PEEP-related hemodynamic complications, and only a few centers continued through the years to report and underline the crucial role of hemodynamics in the framework of PEEP (18-20).
In this paper, we would like to discuss the use of PEEP from these two different perspectives (oxygenation and lung protection) and to speculate on its ‘personalized’ application.
Mechanisms of PEEP
To properly apply PEEP, it is first mandatory to understand how it works, its benefits and its drawbacks. PEEP is usually related to lung recruitment and it is common to read statements as “PEEP-related recruitment”. This is misleading for at least four reasons:
- PEEP is an intensive property of the system, while the recruitment is a capacitive property;
- Recruitment is an inspiratory phenomenon, while PEEP relates to the expiratory phase;
- PEEP, through transpulmonary pressure acts not only on recruitable pulmonary units, but on every pulmonary units open to the ventilation;
- PEEP acts not as such, but through the transpulmonary pressure.
Intensive and capacitive properties
By definition, an intensive property of a system is a physical property whose magnitude is independent of the size of the system, while a capacitive (or extensive) property strictly depends on the size system. In other words, a pressure (such as a PEEP) of 10 cmH2O is the same, either applied to the lung of a mouse or to the lung of an elephant. On the contrary, the same percentage of recruited tissue means an enormous difference in absolute value, as recruitment is a capacitive property. This distinction is not just academic, as we will discuss later.
Inspiratory and expiratory recruitment
Inspiratory recruitment
During inspiration, if the applied pressure is sufficient, previously collapsed pulmonary units will open and inflate. We have then to understand: first, why and how the units are collapsed; second, where are they located; finally, how the opening pressures actually work.
- A collapsed unit may be defined as a pulmonary unit where the gas content is near zero or nil. The first kind of collapse [loose atelectasis (21)] is due to the small airways collapse, primarily because of the increased lung weight; this “squeezes” the gas out of the unit and closes the small airways. Some gas is left behind the collapsed airway (gas content near zero). The second kind of collapse [sticky atelectasis (21)] is due to the complete reabsorption of the gases from the pulmonary unit. This occurs whenever and wherever a tributary airway stays closed throughout the entire respiratory cycle. During ARDS, other pulmonary units may present as a gasless, but are “consolidated” instead of collapsed. These units are usually filled with liquid/solid material originating from the disease process leading to ARDS. In practice, collapsed and consolidated units may be differentiated only after a given “maximal” opening pressure is applied;
- The number of units in which the collapse is primarily due to the gravitational forces increases when the superimposed pressure (i.e., the lung mass times the vertical height) increases (22-24). It must be noted, however, that the units at a given iso-gravitational plane aren’t necessarily all open or all closed, as local phenomena of interaction between contiguous units may prevent or favor their closure. Note that the unit collapsing at end-expiration remains “loose” if they reopen and receive gas during the next inspiration, otherwise they become “sticky” atelectasis with time. While the most frequent loose atelectasis follows a quite definite spatial orientation (from non-dependent to dependent lung) (25,26), the reabsorption atelectasis arises both in the most dependent lung regions (where the inspiratory pressure isn’t sufficient to open the gravitational dependent collapsed units) and wherever an airway obstruction occurs for non-gravitational reasons;
- To open a given unit the applied pressure must overcome at least four distinct forces (ignoring the gas movement):
- The surface tension forces (27). These are likely lower in the “loose” atelectasis, where some gas is still present, than in the “sticky” atelectasis, where all the water molecules are in contact with each other;
- The pressure superimposed to that given unit (22,23);
- The pressure likely due to the interaction between neighboring units collapsed in an iso-gravitational plane (28);
- The pressure needed to lift up the chest wall at the same volume to which the lung has been inflated (24).
Taking into account all these phenomena may contribute in understanding the behavior of the opening pressures. In Figure 1 we present an inspiratory recruitment-airway pressure curve measured in 34 ARDS patients. As shown, the shape is sigmoidal (29,30), which, expressed as opening pressure distribution, results in a Gaussian curve. Accordingly, most of the recruitment occurs at 20 cmH2O, while few units require either very low pressures or very high pressures (26,31). Actually, considering a standard sternum-vertebral height of 15 cm, a normal chest wall elastance and the pressure required to overcome the tension forces, ignoring the interaction between neighboring units, the order of magnitude for opening a unit compressed by 15 cmH2O (a theoretical limit) would be:
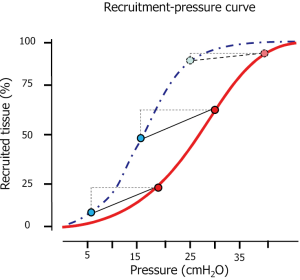
Opening pressure = compressive forces (10–15 cmH2O) + surface tension (15–20 cmH2O) + chest wall (5–10 cmH2O) = 30–45 cmH2O
As shown, at 45 cmH2O, most of the possible recruitment should be accomplished in the majority of the patients. A minor fraction (2–3%) of the recruitment may occur at higher pressure (45–60 cmH2O) (31), possibly due to the interaction between collapsed units. Therefore, to open completely the lung, pressures as high as 45 cmH2O (if the thoracic cage is normal) are usually required. Of note, at the recommended plateau pressure of 30 cmH2O (32), a consistent fraction of the lung (up to 30% in severe ARDS) remains closed (28).
Expiratory recruitment
It is well known that the pressures needed to keep open a given pulmonary unit are far lower than the ones required for opening it. The main reason is that the fraction of pressure which was required to overcome the surface forces during inspiration is no longer necessary during expiration. Actually, the surfactant function was identified by analyzing the differences between a lung volume-pressure curve performed inflating the lung with gas (surface forces present) or with saline (surface forces absent) (33,34). As a result, the pressure needed to keep the lung open is far lower than the one needed for opening it (see Figure 1). As shown, most of the lung is kept open at airway pressure around 10 cmH2O. Pressures as high as 20–25 cmH2O may be necessary to keep open some pulmonary units.
Recruitment versus inflation
Anytime the end-expiratory pressure is applied, it will keep open a certain fraction of the previously recruited lung, while keeping the already opened lung units at a higher level of inflation. If we define, as we believe correct, the recruitment as the mass of pulmonary units regaining and maintaining inflation, it is convenient to estimate how much of the gas volume due to the presence of PEEP is distributed in the newly recruited units and how much is distributed in already open units. As shown in Figure 2, most of the PEEP volume enters the units already inflated (35). Therefore, the effect of PEEP is dual: on one side, it maintains a minimal amount of gas in newly opened units; on the other side, it increases the aeration of the units already open, even causing an overstretch. In Figure 3 we present a model of distribution for a PEEP increase from 0 to 25 cmH2O in a hypothetical representative ARDS lung. It must be noted that in most of the cases, a pressure of 20 cmH2O generates an end-expiratory lung volume (EELV) almost equal to the total lung capacity (TLC). This is the volume on which the tidal volume (VT) is superimposed. The recruitment of new tissue and expansion of previously inflated lung accounts for the large difference reported in literature where recruitment is assessed (35). The CT scan allows to exactly quantify the amount of lung tissue regaining by inflation. The magnitude of this fraction, related to the total lung weight, ranges between 0 and 40% with a median value around 10–12%, depending on the severity of the studied ARDS population. In contrast, all the recruitment assessment based on gas measurements, such as the dual volume-pressure curve method or the gas method we implemented in 1998 (37) and Dellamonica reintroduced in 2011 (38), do not measure only the gas entering in the previously degassed regions, but also the amount of gas entering the already open units, which, at higher volume, increase their compliance (35).
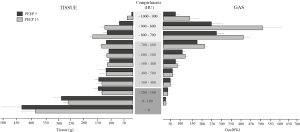
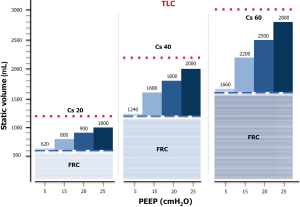
Transpulmonary pressure
It must be noted that all the above considerations should refer not to the airway pressure, but instead to the transpulmonary pressure. In a given patient, changes in transpulmonary pressure are related to the changes of airway pressure by the following relationship (39):
Transpulmonary pressure (PL) = Paw × EL/Etot
Where Paw stands for airway pressure, EL elastance of the lung and Etot elastance of the respiratory system (i.e., elastance of the lung plus elastance of the chest wall).
It must be noted that the so-called transmission is related to the ratio of the lung to the total elastance. This, in normal conditions, is ~0.5, while in ARDS it can range between 0.2 and 0.8. This underlines the need for measuring the transpulmonary pressure for a safer application of mechanical ventilation.
Clinical application of PEEP
PEEP targets
When selecting the PEEP level, we should consider the oxygenation advantage and the putative benefits on “lung protection”.
PEEP and oxygenation
For decades, the sole purpose of introducing PEEP in the ARDS management was to improve oxygenation. Less attention was paid to the PCO2, and its possible protective effects were not really considered, although proposed in experimental animals, until the development of the “atelectrauma theory” in the late 1980s. PEEP improves oxygenation through two possible mechanisms: (I) keeping open previously collapsed and perfused regions, causes an intra-pulmonary right-to-left shunt (venous admixture) decrease; (II) decreasing the cardiac output, generates a reduction of intra-pulmonary right-to-left shunt.
- Although low ventilation-perfusion (VA/Q) regions are represented in ARDS, the main mechanism of hypoxemia is the relevant presence of right-to-left shunt (40). Indeed Riley’s model (41), referring to the lung as a three-compartment reality (dead space, “normal VA/Q” and shunt) well applies to the ARDS lung. The shunt fraction is usually lower than the fraction of non-aerated tissue due to hypoxic vasoconstriction, which is dampened, but still present (42). If PEEP is able to maintain open units previously collapsed and perfused, the shunt fraction decreases.
- ARDS is characterized by elevated vascular resistance due to diffuse vasoconstriction. The vessels serving open pulmonary units, however, are more dilated than the ones serving collapsed units. An increase of cardiac output, associated with an increase of pulmonary artery pressure is preferentially distributed in these latter units, increasing the right-to-left shunt (11,12). While these mechanisms are likely operating when PEEP is present, they have been challenged during unsupported spontaneous breathing (43).
Due to these dual mechanism, PEEP and more in general the increase of pressures, is associated in nearly the totality of cases, with an increase in PaO2. A decrease of PaO2 when PEEP is increased must be carefully investigated, as it may reveal unusual mechanisms operating in the system (44-46).
If PEEP is used to provide viable oxygenation but with a minimum risk of overinflation and of hemodynamic instability, a reasonable PaO2 target should be established. In our opinion, this approach lacks sound physiological background. Referring only to a PaO2 value is insufficient, as what matters is the tissue oxygenation (served by hemodynamics), which decreases while the PaO2 increases when PEEP is applied. A right balance should be pursued by the physician, taking into account the trajectory of the disease, the patient’s physiological reserve and the relevance of possible comorbidities.
PEEP and lung protection
The protective effect of PEEP is commonly referred to a seminal paper by Webb and Tierney, who showed, in 2-hour experiments on rats, that lung edema was worse when ventilating the lungs between 45 cmH2O plateau and 0 cmH2O PEEP than 45 cmH2O plateau and 10 cmH2O PEEP (17). This figure is still today reported in meetings and papers as an unquestionable proof of the protective effect of PEEP. In the same paper, Webb and Tierney also report data (mostly neglected) showing that ventilating at 30 cmH2O plateau and 0 cmH2O PEEP did not cause dramatic damages. Despite the large consensus on the importance of atelectrauma, and its prevention by PEEP, bedrock of the protective lung strategy, in our opinion the role of PEEP should be reappraised. Actually, the basic question is whether the protection of PEEP is just a side effect of the decrease in tidal volume, commonly associated with the increase of PEEP since the Webb and Tierney experiments, or, in contrast, if the PEEP acts directly on preventing barotrauma and VILI, as proposed by the lung protective strategy. We think that considering the PEEP protective is more a belief (47) than an evidence or even a physiology-supported reality. Actually, we doubt the direct protective effect of PEEP (particularly of higher PEEP) for three main reasons:
- PEEP is a pressure and as such it is a component of the mechanical power, which is delivered to the lung parenchyma. To distend a lung, energy must be supplied, higher than the one used to keep it distended, and the pre-stressed fibers may reach their unphysiological limits (48);
- A large series of experiments led to the conclusion that what really matters is the mechanical power overcoming the physiological upper limit of the lung. If this is not reached, the presence of PEEP or its absence is irrelevant. If the PEEP is so high that the associated tidal volume overcomes the physiological limits of lung expansion, PEEP is harmful (36);
- Three large studies comparing, despite different methodological approach, lower PEEP (in the range of 8 cmH2O) to higher PEEP (in the range of 15 cmH2O) did not find any significant difference. That means that the putative atelectrauma associated with lower PEEP is not more dangerous than the putative volutrauma associated with higher PEEP (49).
This does not indicate that PEEP is useless, but simply that the open lung theory is not necessarily a correct conceptual approach.
PEEP in individual patients
Patient characterization
The most important preliminary step in the PEEP selection process, either if the target is exclusively to improve oxygenation either to obtain a fully open lung, is to assess the lung recruitability, an extensive property of the system which solely depends on the nature and the extent of the disease leading to the ARDS (50). Several methods have been suggested to directly, or indirectly, assess lung recruitability, based either on imaging (direct assessment) or on gas exchange/respiratory system mechanic variables during challenge tests (indirect methods).
- Imaging:
- CT scan. The best tool to measure the recruitability is by quantitatively analyzing two CT scans taken at different pressure levels. The difference between the amount of gasless tissue at lower pressure and the gasless tissue at higher pressure, normalized for lung weight, gives the percent recruitability (51);
- Electric impedence tomography (EIT). The advantage of using this technique is its availability at the bedside. However, it reflects more a better overall aeration of the lung between two levels of PEEP than the opening of previously gasless tissue. Accordingly, the CT scan-EIT correlation in assessing the recruitability is quite poor (52), while it is better if compared with gas-assessed recruitability (53). It is worth re-emphasizing that the gas method preferentially measures the better inflation at higher PEEP of previously inflated units at lower PEEP than the opening of previously collapsed units (35);
- Ultrasound. Also this technique is available at the bedside (54). Beside the enthusiasm of their early proponents (55), it suffers, in our opinion, from several limitations, as the lack of spatial resolution and the limited penetration of ultrasound. Finally, as the EIT and the gas-based methods, it is related with the overall better inflation instead of the opening of previously non aerated tissue;
- Challenge tests. A complete review of the different tests has been recently published (56) and here we will only summarize them:
- PEEP test during inspiration. Typically, 5, 10 and 15 (sometimes up to 20) cmH2O are applied, 15 to 30 minutes apart (equilibration time may be reduced to 10 minutes) (57);
- PEEP test during expiration. After a full inflation up to 45 cmH2O (or sometimes 60 cmH2O), airway pressure with PEEP around 25 cmH2O, the PEEP is progressively decreased until either oxygenation or respiratory system compliance deteriorates. This value is considered as the beginning of lung collapse (58,59);
- Another possibility is to use the Berlin classification when PaO2/FiO2 ratio is assessed at 5 cmH2O PEEP. In this case, we may expect, with some degree of probability (70–80%), that recruitability reflects the ARDS severity (60).
Each of these systems presents its own problems. The CT scan requires an intense work, both for the test and the analysis. The EIT is promising, but at the moment it is semi-quantitative. The ultrasound can only explore the “shell” of the lung. All the tests referring to the gas exchange variables do not take into account the role of the hemodynamics, nor the behavior of PaCO2. An increase of PaCO2 at constant minute ventilation usually indicates an increase of overinflation (i.e., pulmonary units more inflated and less ventilated). As a surrogate for hemodynamics, it can be useful to consider the central venous hemoglobin oxygen saturation (ScvO2). If both arterial oxygenation and ScvO2 increase, it is likely that hemodynamics are well preserved. Attention should be paid when the oxygenation increases and the saturation does not, since possible danger for the hemodynamics may be displayed by an increase of PaO2 associated with a decrease of central venous saturation. Therefore, the assessment of the arterial-venous oxygen content difference may prevent us to wrongly attribute to recruitment an increase of PaO2 simply due to a cardiac output decrease (61).
Choice of PEEP
Once the recruitment has been assessed, we have to balance the possible benefits and risks of different levels of PEEP. When different PEEP selection methods were compared, the one based on the revised P/F ratio-PEEP table, which is based on experts opinion (62), was the only one which provided lower PEEP in patients with lower recruitability, compared to methods based on lung mechanics (63,64), transpulmonary pressure (65), or CT scan (24). Most of the lung mechanics-based methods, and even the CT-based method, did provide equally higher PEEP in patients with high, intermediate or low recruitability as inferred from a modified Berlin classification (49). By the way, we recently showed that a lung cannot be fully opened at ventilation set between 15 cmH2O PEEP and 30 cmH2O plateau pressure (28). The full opening likely requires PEEP greater than 25 cmH2O, whatever the recruitability is. Indeed, to keep open 1 or 1,000 grams of non-aerated tissue (extensive property) requires the same PEEP if the opening pressures are the same. In addition, in routine practice, the PEEP used worldwide in severe ARDS lies around 8.5 cmH2O, suggesting that in the normal clinical practice the intensivist targets more a viable oxygenation than an open lung (66). Therefore, it is quite clear that nobody has the recipe for some ideal PEEP setting that likely doesn’t exist. We believe that in early full-blown ARDS the severity and the recruitability suggest that values around 15 cmH2O in severe patients, although non preventing the opening-closing, are sufficient to keep open at least 70% of the lung and to provide viable gas exchange. In moderate ARDS, values around 10 cmH2O are indicated and in mild ARDS even lower PEEP is more than adequate, since the recruitability is extremely low (67).
Acknowledgements
None.
Footnote
Conflicts of Interest: The authors have no conflicts of interest to declare.
References
- Barach AL, Martin J, Eckman M. Positive pressure respiration and its application to the treatment of acute pulmonary edema. Ann Intern Med 1938;12:754-95. [Crossref]
- Cournand A, Motley HL, Werko L. Mechanism underlying cardiac output change during intermittent positive pressure breathing (IPP). Fed Proc 1947;6:92. [PubMed]
- Gregory GA, Kitterman JA, Phibbs RH, et al. Treatment of the idiopathic respiratory-distress syndrome with continuous positive airway pressure. N Engl J Med 1971;284:1333-40. [Crossref] [PubMed]
- Ashbaugh DG, Bigelow DB, Petty TL, et al. Acute respiratory distress in adults. Lancet 1967;2:319-23. [Crossref] [PubMed]
- Gattinoni L, Quintel M. Fifty Years of Research in ARDS Why Is Acute Respiratory Distress Syndrome So Important for Critical Care? Am J Respir Crit Care Med 2016;194:1051-2. [Crossref] [PubMed]
- Falke KJ, Pontoppidan H, Kumar A, et al. Ventilation with end-expiratory pressure in acute lung disease. J Clin Invest 1972;51:2315-23. [Crossref] [PubMed]
- Suter PM, Fairley B, Isenberg MD. Optimum end-expiratory airway pressure in patients with acute pulmonary failure. N Engl J Med 1975;292:284-9. [Crossref] [PubMed]
- Kirby RR, Downs JB, Civetta JM, et al. High level positive end expiratory pressure (PEEP) in acute respiratory insufficiency. Chest 1975;67:156-63. [Crossref] [PubMed]
- Tenaillon A, Labrousse J, Gateau O, et al. Optimal positive end-expiratory pressure and static lung compliance. N Engl J Med 1978;299:774-5. [Crossref] [PubMed]
- Dantzker DR, Lynch JP, Weg JG. Depression of cardiac output is a mechanism of shunt reduction in the therapy of acute respiratory failure. Chest 1980;77:636-42. [Crossref] [PubMed]
- Rapin M, Lemaire F, Regnier B, et al. Increase of intrapulmonary shunting induced by dopamine. Proc R Soc Med 1977;70 Suppl 2:71-5. [PubMed]
- Lemaire F, Harf A, Simonneau G, et al. Gas exchange, static pressure-volume curve and positive-pressure ventilation at the end of expiration. Study of 16 cases of acute respiratory insufficiency in adults. Ann Anesthesiol Fr 1981;22:435-41. [PubMed]
- Tremblay L, Valenza F, Ribeiro SP, et al. Injurious ventilatory strategies increase cytokines and c-fos m-RNA expression in an isolated rat lung model. J Clin Invest 1997;99:944-52. [Crossref] [PubMed]
- Mead J, Takishima T, Leith D. Stress distribution in lungs: a model of pulmonary elasticity. J Appl Physiol 1970;28:596-608. [PubMed]
- Lachmann B. Open up the lung and keep the lung open. Intensive Care Med 1992;18:319-21. [Crossref] [PubMed]
- Ranieri VM, Suter PM, Tortorella C, et al. Effect of mechanical ventilation on inflammatory mediators in patients with acute respiratory distress syndrome: a randomized controlled trial. JAMA 1999;282:54-61. [Crossref] [PubMed]
- Webb HH, Tierney DF. Experimental pulmonary edema due to intermittent positive pressure ventilation with high inflation pressures. Protection by positive end-expiratory pressure. Am Rev Respir Dis 1974;110:556-65. [PubMed]
- Jardin F, Farcot JC, Boisante L, et al. Two dimensional echocardiography during controlled ventilation with PEEP. Intensive Care Med 1980;6:204. [Crossref] [PubMed]
- Bouferrache K, Vieillard-Baron A. Acute respiratory distress syndrome, mechanical ventilation, and right ventricular function. Curr Opin Crit Care 2011;17:30-5. [Crossref] [PubMed]
- Vieillard-Baron A, Matthay M, Teboul JL, et al. Experts’ opinion on management of hemodynamics in ARDS patients: focus on the effects of mechanical ventilation. Intensive Care Med 2016;42:739-49. [Crossref] [PubMed]
- Pelosi P, Cadringher P, Bottino N, et al. Sigh in acute respiratory distress syndrome. Am J Respir Crit Care Med 1999;159:872-80. [Crossref] [PubMed]
- Gattinoni L, D'Andrea L, Pelosi P, et al. Regional effects and mechanism of positive end-expiratory pressure in early adult respiratory distress syndrome. JAMA 1993;269:2122-7. [Crossref] [PubMed]
- Pelosi P, D'Andrea L, Vitale G, et al. Vertical gradient of regional lung inflation in adult respiratory distress syndrome. Am J Respir Crit Care Med 1994;149:8-13. [Crossref] [PubMed]
- Cressoni M, Chiumello D, Carlesso E, et al. Compressive forces and computed tomography-derived positive end-expiratory pressure in acute respiratory distress syndrome. Anesthesiology 2014;121:572-81. [Crossref] [PubMed]
- Pelosi P, Goldner M, McKibben A, et al. Recruitment and derecruitment during acute respiratory failure: an experimental study. Am J Respir Crit Care Med 2001;164:122-30. [Crossref] [PubMed]
- Crotti S, Mascheroni D, Caironi P, et al. Recruitment and derecruitment during acute respiratory failure: a clinical study. Am J Respir Crit Care Med 2001;164:131-40. [Crossref] [PubMed]
- Ghadiali SN, Gaver DP. Biomechanics of liquid-epithelium interactions in pulmonary airways. Respir Physiol Neurobiol 2008;163:232-43. [Crossref] [PubMed]
- Cressoni M, Chiumello D, Algieri I, et al. Opening pressures and atelectrauma in acute respiratory distress syndrome. Intensive Care Med 2017;43:603-11. [Crossref] [PubMed]
- Hickling KG. The pressure-volume curve is greatly modified by recruitment. A mathematical model of ARDS lungs. Am J Respir Crit Care Med 1998;158:194-202. [Crossref] [PubMed]
- Harris RS, Hess DR, Venegas JG. An objective analysis of the pressure-volume curve in the acute respiratory distress syndrome. Am J Respir Crit Care Med 2000;161:432-9. [Crossref] [PubMed]
- Borges JB, Okamoto VN, Matos GF, et al. Reversibility of lung collapse and hypoxemia in early acute respiratory distress syndrome. Am J Respir Crit Care Med 2006;174:268-78. [Crossref] [PubMed]
- Tobin MJ. Culmination of an era in research on the acute respiratory distress syndrome. N Engl J Med 2000;342:1360-1. [Crossref] [PubMed]
- von Neergaard K. Neue Auffassungenüber einen Grandbegriff der Atemmechanik. Die Retraktionskraft der Lunge, abhängig non der Oberflachenspannung in den Alveolen. 1929:373.
- Radford E. Static mechanical properties of mammalian lungs. Handbook of physiology 1964;1:429-49.
- Chiumello D, Marino A, Brioni M, et al. Lung Recruitment Assessed by Respiratory Mechanics and Computed Tomography in Patients with Acute Respiratory Distress Syndrome. What Is the Relationship? Am J Respir Crit Care Med 2016;193:1254-63. [Crossref] [PubMed]
- Protti A, Andreis DT, Milesi M, et al. Lung anatomy, energy load, and ventilator-induced lung injury. Intensive Care Med Exp 2015;3:34. [Crossref] [PubMed]
- Gattinoni L, Pelosi P, Suter PM, et al. Acute respiratory distress syndrome caused by pulmonary and extrapulmonary disease. Different syndromes? Am J Respir Crit Care Med 1998;158:3-11. [Crossref] [PubMed]
- Dellamonica J, Lerolle N, Sargentini C, et al. PEEP-induced changes in lung volume in acute respiratory distress syndrome. Two methods to estimate alveolar recruitment. Intensive Care Med 2011;37:1595-604. [Crossref] [PubMed]
- Gattinoni L, Carlesso E, Cadringher P, et al. Physical and biological triggers of ventilator-induced lung injury and its prevention. Eur Respir J Suppl 2003;47:15s-25s. [Crossref] [PubMed]
- Pesenti A, Latini R, Riboni A, et al. Simple estimate of the true right to left shunt (Qs/Qt) at maintenance F1O2 by sulphur hexafluoride retention. Intensive Care Med 1982;8:283-6. [Crossref] [PubMed]
- Riley RL, Cournand A. Ideal alveolar air and the analysis of ventilation-perfusion relationships in the lungs. J Appl Physiol 1949;1:825-47. [PubMed]
- Cressoni M, Caironi P, Polli F, et al. Anatomical and functional intrapulmonary shunt in acute respiratory distress syndrome. Crit Care Med 2008;36:669-75. [Crossref] [PubMed]
- Vimlati L, Larsson A, Hedenstierna G, et al. Pulmonary shunt is independent of decrease in cardiac output during unsupported spontaneous breathing in the pig. Anesthesiology 2013;118:914-23. [Crossref] [PubMed]
- Lemaire F, Richalet JP, Carlet J, et al. Postoperative hypoxemia due to opening of a patent foramen ovale confirmed by a right atrium-left atrium pressure gradient during mechanical ventilation. Anesthesiology 1982;57:233-6. [Crossref] [PubMed]
- Granati GT, Teressa G. Worsening Hypoxemia in the Face of Increasing PEEP: A Case of Large Pulmonary Embolism in the Setting of Intracardiac Shunt. Am J Case Rep 2016;17:454-8. [Crossref] [PubMed]
- Tavazzi G, Pozzi M, Via G, et al. Weaning Failure for Disproportionate Hypoxemia Caused by Paradoxical Response to Positive End-Expiratory Pressure in a Patient with Patent Foramen Ovale. Am J Respir Crit Care Med 2016;193:e1-2. [Crossref] [PubMed]
- Gattinoni L, Marini JJ, Quintel M. Evidence or belief-based medicine? Ten doubts. Intensive Care Med 2017. [Epub ahead of print]. [Crossref] [PubMed]
- Gattinoni L, Tonetti T, Cressoni M, et al. Ventilator-related causes of lung injury: the mechanical power. Intensive Care Med 2016;42:1567-75. [Crossref] [PubMed]
- Chiumello D, Cressoni M, Carlesso E, et al. Bedside selection of positive end-expiratory pressure in mild, moderate, and severe acute respiratory distress syndrome. Crit Care Med 2014;42:252-64. [Crossref] [PubMed]
- Gattinoni L, Caironi P, Cressoni M, et al. Lung recruitment in patients with the acute respiratory distress syndrome. N Engl J Med 2006;354:1775-86. [Crossref] [PubMed]
- Gattinoni L, Caironi P, Pelosi P, et al. What has computed tomography taught us about the acute respiratory distress syndrome? Am J Respir Crit Care Med 2001;164:1701-11. [Crossref] [PubMed]
- Costa EL, Borges JB, Melo A, et al. Bedside estimation of recruitable alveolar collapse and hyperdistension by electrical impedance tomography. Intensive Care Med 2009;35:1132-7. [Crossref] [PubMed]
- Mauri T, Eronia N, Turrini C, et al. Bedside assessment of the effects of positive end-expiratory pressure on lung inflation and recruitment by the helium dilution technique and electrical impedance tomography. Intensive Care Med 2016;42:1576-87. [Crossref] [PubMed]
- Bouhemad B, Brisson H, Le-Guen M, et al. Bedside ultrasound assessment of positive end-expiratory pressure-induced lung recruitment. Am J Respir Crit Care Med 2011;183:341-7. [Crossref] [PubMed]
- Rouby JJ, Arbelot C, Brisson H, et al. Measurement of Alveolar Recruitment at the Bedside: The Beginning of a New Era in Respiratory Monitoring? Respir Care 2013;58:539-42. [Crossref] [PubMed]
- Gattinoni L, Carlesso E, Cressoni M. Selecting the “right” positive end-expiratory pressure level. Curr Opin Crit Care 2015;21:50-7. [Crossref] [PubMed]
- Chiumello D, Coppola S, Froio S, et al. Time to reach a new steady state after changes of positive end expiratory pressure. Intensive Care Med 2013;39:1377-85. [Crossref] [PubMed]
- Hickling KG. Best compliance during a decremental, but not incremental, positive end-expiratory pressure trial is related to open-lung positive end-expiratory pressure: a mathematical model of acute respiratory distress syndrome lungs. Am J Respir Crit Care Med 2001;163:69-78. [Crossref] [PubMed]
- Albaiceta GM, Taboada F, Parra D, et al. Tomographic study of the inflection points of the pressure-volume curve in acute lung injury. Am J Respir Crit Care Med 2004;170:1066-72. [Crossref] [PubMed]
- Caironi P, Carlesso E, Cressoni M, et al. Lung recruitability is better estimated according to the Berlin definition of acute respiratory distress syndrome at standard 5 cmH2O rather than higher positive end-expiratory pressure: a retrospective cohort study. Crit Care Med 2015;43:781-90. [Crossref] [PubMed]
- Gattinoni L, Pesenti A, Matthay M. Understanding blood gas analysis. Intensive Care Med 2017. [Epub ahead of print]. [Crossref] [PubMed]
- Meade MO, Cook DJ, Guyatt GH, et al. Ventilation strategy using low tidal volumes, recruitment maneuvers, and high positive end-expiratory pressure for acute lung injury and acute respiratory distress syndrome: a randomized controlled trial. JAMA 2008;299:637-45. [Crossref] [PubMed]
- Grasso S, Terragni P, Mascia L, et al. Airway pressure-time curve profile (stress index) detects tidal recruitment/hyperinflation in experimental acute lung injury. Crit Care Med 2004;32:1018-27. [Crossref] [PubMed]
- Mercat A, Richard JC, Vielle B, et al. Positive end-expiratory pressure setting in adults with acute lung injury and acute respiratory distress syndrome: a randomized controlled trial. JAMA 2008;299:646-55. [Crossref] [PubMed]
- Talmor D, Sarge T, Malhotra A, et al. Mechanical ventilation guided by esophageal pressure in acute lung injury. N Engl J Med 2008;359:2095-104. [Crossref] [PubMed]
- Bellani G, Laffey JG, Pham T, et al. Epidemiology, Patterns of Care, and Mortality for Patients With Acute Respiratory Distress Syndrome in Intensive Care Units in 50 Countries. JAMA 2016;315:788-800. [Crossref] [PubMed]
- Gattinoni L, Carlesso E, Brazzi L, et al. Friday night ventilation: a safety starting tool kit for mechanically ventilated patients. Minerva Anestesiol 2014;80:1046-57. [PubMed]