Cardiotoxicity of cancer chemotherapy: identification, prevention and treatment
Introduction
Cancer is the second largest cause of mortality in the USA (1). Advances in the early diagnosis, precise staging and therapy have significantly reduced the mortality and increased longevity in cancer patients. An estimated 14.5 million people are currently living with a history of cancer in the USA. This number is projected to rise to 19 million over the next 10 years (1). Approximately 12,500 new cases of cancer are diagnosed annually in the children and adolescents in the USA with over 300,000 survivors of childhood cancers. A significant proportion of cancer survivors are living with long-term adverse effects of cancer therapy, involving multiple organ systems. Cardiovascular toxicity of cancer therapy is a major concern in this regard. Several anticancer agents, such as anthracyclines, trastuzumab, cyclophosphamide, 5-fluorouracil, angiogenesis inhibitors and tyrosine kinase inhibitors (TKIs) are associated with an increase in the risk of cardiovascular morbidity and mortality. Childhood cancer survivors face a high lifetime risk of late cardiovascular disease (2,3). The spectrum of cardiovascular complications of cancer therapy is wide and includes left ventricular (LV) dysfunction, congestive heart failure (CHF), coronary vasospasm, angina, myocardial infarction, arrhythmias, systemic hypertension, pericardial effusion, pulmonary fibrosis and pulmonary hypertension. The risk of cardiotoxicity is higher in patients with pre-existing cardiovascular diseases. Cardio-oncology is a newly emerging subspecialty to address the complex interaction between cancer and cardiovascular system; monitoring, early detection, prevention and treatment of cardiotoxicity of cancer therapies; development of newer therapies with lower or no cardiotoxicity; and careful planning of cancer therapy in patients with pre-existing cardiovascular disease to avoid overt cardiotoxicity and heart failure (4-6).
Cancers can affect cardiovascular system in multiple ways. Primary tumors of the heart are relatively rare, but often associated with dramatic and catastrophic clinical presentations. More commonly, tumors from lungs, breast, esophagus and other mediastinal structures or those metastasizing to lungs or mediastinum can directly invade or compress the heart, great vessels or the pericardial space. Malignancies of the plasma cells can result in deposition of immunoglobulins or their fragments in multiple organs including the heart, resulting in myocardial dysfunction, heart failure and arrhythmias. Cancers can affect the coagulation cascade resulting in hypercoagulability and thromboembolic disorders. All of these aspects are highly relevant to the field of cardio-oncology. The current article is primarily focused on the direct cardiovascular toxicity of cancer therapy. Several recent articles have addressed these ancillary aspects of cardio-oncology in detail (5,6).
Table 1 lists the adverse cardiovascular effects of commonly used cancer therapies. Following is a brief description of most commonly used cancer therapy agents and their cardiovascular toxicity
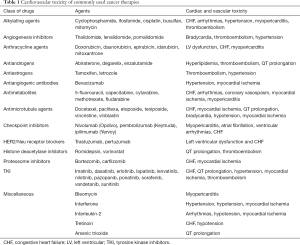
Full table
Anthracyclines
Anthracycline agents are antibiotics isolated from soil microbe Streptomyces peucetius, and are highly effective, broad-spectrum anti-cancer agents. Cardiotoxicity is a major limitation of their administration (5-10). These agents contain an anthraquinone ring, which results in the formation of hydrogen peroxide and reactive oxygen species through redox cycling (10). Anthracyclines inhibit DNA and RNA synthesis by intercalating between the base pairs and by inhibiting the activity of topoisomerase II, which prevents DNA repair (11). Oxidative injury from free radicals, peroxidation of membrane lipids, altered calcium handling by sarcoplasmic reticulum, and impaired protein synthesis also contribute to anthracycline cardiotoxicity (7,11). Anthracyclines result in progressive and cumulative myocellular injury, myocyte loss due to apoptosis and necrosis, LV dilation, adverse remodeling, impaired contractility and CHF. Anthracycline cardiotoxicity is categorized as acute and chronic. Acute cardiotoxicity occurs during or soon after initiation of therapy. This is usually transient and self-limiting with a myopericarditis like picture, non-specific repolarization changes on ECG, dysrhythmias, troponin elevation, and transient LV dysfunction (12). These abnormalities usually resolve spontaneously with only supportive therapy. In contrast, chronic cardiotoxicity is the most common and relevant form of anthracycline cardiotoxicity. This comprises of LV systolic dysfunction, which is insidious in onset and asymptomatic in the early stages, but can progress to dilated cardiomyopathy and overt CHF, which is generally irreversible. Chronic cardiotoxicity is arbitrarily classified as type 1 or early onset (typically detected within one year of completion of chemotherapy) and type 2 or late-onset (usually detected after the first year with an unlimited time frame of up to decades after completion of chemotherapy) (6,13,14). Majority of the patients develop chronic cardiotoxicity within the first year of completing therapy (9). The total lifetime cumulative dose of anthracycline is the most important determinant of anthracycline cardiotoxicity (15). High intensity chemotherapy increases the risk of cardiotoxicity. Age above 65 years or below 5 years, hypertension, female gender, preexisting cardiac disease, concomitant mediastinal irradiation or therapy with other cardiotoxic agents, high dose cyclophosphamide therapy, a higher cumulative dose (above 450 mg/m2) and larger individual anthracycline doses increase the risk of developing anthracycline cardiotoxicity. Subclinical iron overload due to multiple blood transfusions, therapeutic iron supplementation, and genetic mutations of iron handling are also suspected to confer increased susceptibility to anthracycline cardiotoxicity (16,17).
Asymptomatic and subclinical LV dysfunction generally precedes overt CHF (18-21). A prompt discontinuation of anthracycline therapy with the onset of asymptomatic LV dysfunction can prevent its progression to CHF and is pivotal to the current strategies for the prevention of anthracycline related CHF. Chronic anthracycline cardiotoxicity can also present as restrictive cardiomyopathy with diastolic dysfunction and heart failure with concomitant mediastinal irradiation. Prior to the widespread use of serial LV function monitoring, the incidence of CHF with doxorubicin was 4–7% in patients receiving 400 to 550 mg/m2, 18% in those receiving 551 to 700 mg/m2, and 30% at doses above 701 mg/m2 (20-22).
A large inter-individual variation in susceptibility to anthracycline cardiotoxicity is likely related, to polymorphisms in genes related to the metabolism of anthracyclines (23), detoxification of free radicals or variations in body iron levels (16,17). However, the exact molecular, biochemical, cellular, and genetic mechanisms of variation in susceptibility to anthracycline cardiotoxicity are not fully understood. Trastuzumab (Herceptin), TKIs and taxanes potentiate the cardiotoxicity of anthracyclines (24). With a marked inter-individual variation in the susceptibility to anthracycline cardiotoxicity it is not practical to choose an arbitrary dose ceiling to prevent CHF, since this would deny the benefit of therapy to those who can tolerate higher doses without developing cardiotoxicity. Therefore, an approach of serial LV function monitoring during anthracycline therapy and discontinuing further therapy at the appearance of subclinical LV dysfunction remains the most effective strategy for preventing overt CHF. There are some important considerations regarding LV function monitoring for the purpose preventing anthracycline cardiotoxicity. Serial LV ejection fraction (EF) is the most widely studied and well-established parameter for preventing anthracycline induced CHF. Since, this approach relies on the detection of a small sub-clinical, but definite fall in LVEF on serial monitoring, for discontinuing anthracycline therapy, the reliability, precision, reproducibility, variability and cost are important considerations while choosing a technique for LVEF monitoring. Several techniques are readily available for LVEF monitoring. They are often used interchangeably in clinical practice. However, for the prevention of anthracycline cardiotoxicity, techniques with higher variability of the measurement are less optimal, whereas techniques with higher reproducibility and less inter-observer variability are far better suited. Equilibrium radionuclide angiography (ERNA) more commonly known as multigated acquisition (MUGA) or radionuclide ventriculography (RNV), and cardiac MR imaging, meet these requirements. Of these, MUGA has been the most widely studied and used technique over the last 35 years. Zaret, Alexander, Schwartz, and colleagues pioneered the concept of predicting doxorubicin-induced CHF by monitoring LVEF using MUGA (18-21). Recommendations for monitoring LVEF during doxorubicin therapy to prevent CHF were developed based on the analysis of these studies (Figure 1). A baseline MUGA is recommended prior to initiating doxorubicin. Subsequent measurements are performed prior to the administration of the next planned dose at predefined intervals. With a normal baseline LVEF, the next measurements are performed at cumulative doses of 250–300 and 400–450 mg/m2 and then prior to each subsequent dose. Discontinuation of doxorubicin is recommended if LVEF decreases ≥10% units from the baseline and reaches an LVEF ≤50%. For patients with abnormal baseline LVEF <50%, serial studies are recommended prior to each subsequent dose of doxorubicin. Discontinuation of doxorubicin is recommended if LVEF decreases ≥10% units from the baseline or reaches LVEF ≤30% (19,20). Anthracycline therapy should be avoided with a baseline LVEF ≤30%. Management concordant with these guidelines resulted in a seven-fold reduction in the incidence of CHF (21). The variability of measured LVEF with 2-dimensional echocardiography is up to 10% (25), making it difficult to detect small changes in LVEF required for the prediction of CHF (21,26). The role of echocardiographic assessment of LVEF in monitoring the risk of CHF in cancer patients is based upon extrapolation from the role of ERNA in this population. Echocardiography is perhaps more suited for pediatric patient population (13), where smaller patient size permits use of higher frequency transducers, better image quality and less acoustic artifact than in adults. Contrast echocardiography represents an important opportunity for further investigation, although its use did not improve accuracy or reproducibility of LVEF measurements (25). 3D echocardiography has been observed to improve the reproducibility of the LVEF on serial studies. LV diastolic dysfunction (prolonged isovolumic relaxation period, reduction in peak flow velocity, the ratio of early peak flow velocity/atrial peak flow velocity, and reduction in the deceleration rate of the early peak flow velocity) precede systolic dysfunction (27). Stoddard et al reported prolongation of isovolumic relaxation time (IVRT) by Doppler echocardiography to predict doxorubicin-induced systolic dysfunction in a study of 26 patients (28). A greater than 37% increase in IVRT had 78% sensitivity and 88% specificity for predicting the development of doxorubicin-induced systolic dysfunction (28). Echocardiographic diastolic parameters are more complex to interpret, have poor reproducibility and not well established for preventing doxorubicin induced CHF (29,30). Several other parameters of LV systolic and diastolic function, such as LV strain (longitudinal strain, short axis strain, tissue Doppler imaging) have also been proposed and studied. Of these, only longitudinal strain has shown promise for routine use. Myocardial strain imaging by echocardiography for the detection of cardiotoxicity of cancer chemotherapy was studied in a meta-analysis of 1,504 patients. Peak systolic longitudinal strain using tissue Doppler-based strain imaging, or by speckle tracking echocardiography detected early myocardial changes during therapy (31). A 10–15% reduction in global longitudinal strain during therapy appears to be a useful parameter for predicting cardiotoxicity and CHF (32,33). However, the specificity of this measure is less well established. Late survivors of cancers, frequently show abnormalities in global, radial, circumferential and longitudinal strain despite normal LVEF. Their value in predicting late LV dysfunction or CHF has not been explored. Larger prospective multicenter studies are required to establish the role of strain imaging to predict anthracycline cardiotoxicity and to prevent CHF. The role of longitudinal strain imaging may be limited in patients with obesity, valvular heart disease, LV hypertrophy, coronary artery disease and old age.
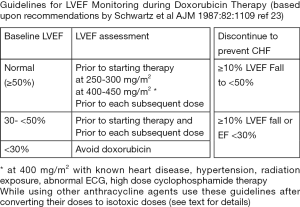
MUGA derived LVEF remains the most widely studied and most reliable parameter for monitoring cardiac function in patients undergoing anthracycline therapy to detect an early fall in LVEF, which is predictive of impending CHF with continuation of anthracycline therapy. Echocardiographically derived longitudinal strain imaging of the LV appears to be promising for detecting early LV dysfunction. However, further studies are warranted.
Recent emphasis on the development of biomarkers indicative of specific tissue and organ damage have resulted in the identification of highly sensitive biomarkers for myocardial injury and damage, such as troponin-T, troponin-I, myoglobin, BNP and proBNP. These biomarkers have been extensively studied and are used routinely in the context of coronary artery disease, acute myocardial infarction and unstable angina. These biomarkers are also being used for the evaluation of conditions associated with myocardial injury and damage not related to coronary artery disease (33). High sensitivity troponin elevation following anthracycline therapy indicates myocardial injury and is predictive of larger and more sustained decline in LVEF compared to those without troponin elevation (34). However, a consistent correlation between troponin elevation and anthracycline or trastuzumab cardiotoxicity has not been found (35-37). Recent availability of high-sensitivity troponin assays may add incremental value to the risk of cardiotoxicity and CHF with cancer therapy. An increase in pro-BNP (brain-type natriuretic peptide) has been reported early after anthracycline administration. However, elevated pro-BNP is not predictive of LV dysfunction. The role of biomarkers is under evaluation in a multicenter NIH funded PREDICT study (ClinicalTrials.gov identifier NCT01032278).
Prevention of anthracycline cardiotoxicity
Approaches to reduce anthracycline cardiotoxicity have met with limited success. Continuous slow infusion of doxorubicin over 24–96 h instead of a single bolus injection was found to lower cardiotoxicity in early clinical studies (38). But reduced cardiotoxicity was not consistently demonstrated in subsequent studies. Moreover, due to the logistical limitations, this approach has not found acceptance in clinical practice. Novel drug delivery system using liposomal incorporation of doxorubicin was developed to improve tumor delivery of doxorubicin and lower its cardiotoxicity. However, the clinical studies have been inconclusive regarding cardiotoxicity of liposomal doxorubicin (39). Several analogues of doxorubicin were evaluated for reduced cardiotoxicity. However, all anthracycline agents have cardiotoxicity. Isotoxic dose conversion is recommended while comparing cardiotoxicity of various anthracycline agents: with a factor of 1.0 for doxorubicin and daunorubicin, 0.67 for epirubicin, 4.0 for mitoxantrone, and 5.0 for idarubicin (40). Perhaps, future genomic and molecular studies may help to identify and develop biomarkers to predict individual predisposition to anthracycline cardiotoxicity prior to starting anthracycline therapy. Such an approach would allow a more effective analysis of risk-benefit ratio, and pre-emptive institution of preventive therapies in high risk-individuals even prior to initiation of anthracycline therapy, and perhaps consider alternative therapies in very high-risk patients.
Treatment of anthracycline cardiotoxicity
Treatment strategies rely on CHF therapy with ACE inhibitor/ARB and beta-blockers. Use of these agents can stabilize, or even improve, LV systolic function (41). Whereas, any ACE inhibitor/ARB can be used for this indication, only carvedilol or nebivolol amongst the beta-blockers have been found to be effective in anthracycline cardiotoxicity. An early detection and early treatment with these agents is associated with a better response compared to those with advanced and long-standing LV dysfunction. Given the high cost associated with the treatment and follow up of CHF (42), serial noninvasive LVEF monitoring is highly cost-effective in preventing CHF (20). Dexrazoxane is an iron chelator, which reduces cardiotoxicity of anthracyclines. However, adverse effects including myelotoxicity and concerns about leukemia have limited its use in clinical practice. This is approved for use only in patients receiving >300 mg/m2 of doxorubicin (43).
Trastuzumab and other HER2 blockers
Trastuzumab (Herceptin) is a humanized monoclonal antibody targeted against a growth receptor protein (HER2/neu) expressed on the cell membranes (44-51). This is used in patients with HER2/neu overexpressing breast cancers and is given as weekly intravenous injection for up to one year. HER2 is a member of HER receptor family. There are 4 types of HER receptors HER1, HER2, HER3 and HER4 (47). These receptors have extracellular, trans-membrane and intracellular domain. Binding of ligand to these receptors results in receptor dimerization and activation, and signaling of PI3K/AKT/mTOR/Ras/MAPK pathways downstream. These pathways signal growth, proliferation and survival of the cells. Blockage of these pathways promotes apoptosis and cell death (44). Approximately 20–25% of breast cancers overexpress HER2/neu. Trastuzumab is effective in combination with other chemotherapy in HER2 over-expressing breast cancers. Trastuzumab can also result in cardiotoxicity. However, the spectrum, mechanism and clinical course of its cardiotoxicity and cardiac dysfunction are quite different and distinct from anthracyclines. Trastuzumab results in LV dysfunction and CHF, which is generally reversible upon discontinuation of therapy (45,46). Unlike anthracyclines, the cardiac dysfunction caused by trastuzumab is not related to cumulative dose and is not associated with cardiomyocyte ultrastructural changes observed with anthracyclines (44). In addition, the risk factors for developing trastuzumab-associated LV dysfunction are not adequately defined except age >65 years and concomitant or prior anthracycline therapy. HER2 receptors are also found on myocytes membrane, where they serve a cardioprotective and proangiogenic role. This explains, in part, the cardiotoxic effects of trastuzumab (46,47), which blocks the HER2 receptor activation.
Trastuzumab was approved in 1998 for first line therapy in HER2 overexpressing breast cancers. Trastuzumab binds to extracellular domain 4 of HER2 receptor, which blocks HER2 signaling. This also activates antibody dependent cytotoxicity and cytolysis. This is approved for early stage as well as metastatic breast cancers overexpressing HER2. Pertuzumab is another humanized monoclonal antibody that binds to extracellular domain 2 of HER2 and was approved for use in HER2 overexpressing breast cancers in 2012 in combination with other chemotherapy (49). Trastuzumab and pertuzumab show synergistic activity and their combined use increase time to tumor progression and overall survival (49). Lapatinib is an intracellular TKI of HER1 and HER2 receptors (50). Dual blockade of the HER2 signaling by both HER2 monoclonal antibody and lapatinib may be more effective than the either agent alone and is under study. All of these agents show cardiotoxicity. Whether combined therapy with these agents increase cardiotoxicity warrants further study.
Trastuzumab induced decline of LVEF generally improves spontaneously upon discontinuation of trastuzumab. Interestingly, re-introduction of trastuzumab after the recovery of LVEF does not necessarily result in recurrence of LV dysfunction (48). The effect of trastuzumab on patients with prior cardiac disease remains unclear. A closer monitoring of LVEF is recommended in such patients (48). Algorithms for LVEF monitoring during adjuvant trastuzumab therapy have been published by Panjrath et al. (48) (Figure 2), and by the Canadian Trastuzumab Working Group (44) and the European Society of Medical Oncologists.
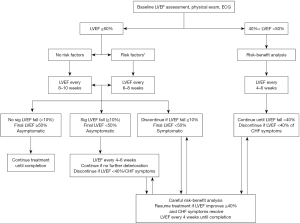
Indium-111 labeled trastuzumab (111In-trastuzumab) imaging can be used to image HER2 receptors expression in the heart. The extent of myocardial HER2 expression as determined by the intensity of myocardial radiotracer uptake on 111In-trastuzumab imaging can predict the susceptibility to trastuzumab cardiotoxicity. Twenty patients with breast cancer underwent 111In-trastuzumab imaging prior to initiating trastuzumab therapy (51). Seven patients showed cardiac radiotracer uptake. Six of these individuals subsequently developed trastuzumab-associated heart failure (51). However, subsequent studies were unable to demonstrate any relationship between cardiac uptake of radiolabeled trastuzumab and development of LV dysfunction. Further studies are needed to determine if molecular imaging techniques such as 111In-trastuzumab imaging can provide any further insight into the mechanism of trastuzumab cardiotoxicity.
Radiation therapy
When heart is included even partially in the radiation field, this can result in cardiovascular injury, which includes CHF, restrictive cardiomyopathy, coronary artery disease, valvular heart disease, constrictive pericarditis and pericardial effusion (52-56). Heart can get radiation in patients undergoing radiation therapy for breast, lung, esophageal and mediastinal malignancies. The risk of cardiac disease is related to the total radiation dose and volume of the heart receiving radiation. A significant increase in the incidence for cardiovascular disease has been observed in long-term cancer survivors exposed to radiation therapy, including increased need for CABG and coronary interventions (52-54). Radiation therapy reduces local recurrence and improved survival in early stage breast cancers. Breast radiation including regional lymph nodes is often used following lumpectomy. Radiation injury to the heart is far more common with left breast irradiation (55). Anthracycline use, older age, and prior CAD are all risk factors for radiation-induced cardiovascular toxicity. Newer techniques for more focused radiation delivery such as three-dimensional planning, which minimizes radiation to the non-target organs, are less cardiotoxic. Myocardial perfusion defects have been reported on myocardial perfusion imaging after radiation treatment of left-sided breast cancer (54,55). Most of these perfusion defects are otherwise asymptomatic, and the long-term consequence of these asymptomatic perfusion abnormalities is not clear. The clinical presentation of CAD following radiotherapy is typically 10 years after completion of treatment (56). Patients undergoing breast, chest or mediastina radiation should undergo long term follow up for development of cardiac disease. Stress myocardial perfusion imaging and echocardiography can detect the presence of coronary artery disease, valvular and pericardial disease in this population.
5-flourouracil and other antimetabolites
Cardiotoxicity has been reported in up to 2–4% of patients receiving the antimetabolite 5-fluorouracil or its analogues (57-60). 5-flurouracil can cause coronary vasospasm resulting in chest pain, myocardial ischemia, myocardial infarction, and death. These adverse effects are more likely to occur in those with pre-existing or previously undocumented CAD, prior radiation therapy, or concomitant use of cisplatin, which by itself can predispose to ischemia (59). These adverse effects resolve with discontinuation of the 5-fluoroucil. Capecitabine, which is a prodrug that is metabolized to 5-FU in the body, has similar cardiotoxic effects (60). 5-fluorouracil and its analogues should be avoided in patients with known CAD and ischemia, who have not undergone revascularization. Elderly patients or those with multiple CAD risk factors should undergo myocardial perfusion imaging prior to undergoing therapy with 5-fluorouracil.
TKIs
Well over 25 TKIs are in clinical use or are undergoing advanced clinical studies (61-64). Tyrosine kinases are enzymes that activate proteins by transferring a phosphate group to the tyrosine residue of the proteins (phosphorylation) in signal transduction cascade. TKIs inhibit the activation of these proteins by tyrosine kinases and interrupt the signal transduction cascade. TKIs are highly selective for inhibiting tyrosine phosphorylation and do not inhibit other protein kinases that phosphorylate serine or threonine residues. These agents inhibit epidermal growth factor receptors (EGRF), and vascular endothelial growth factor (VEGF) signaling pathway thereby inhibiting angiogenesis and growth (62,63). The most common cardiovascular adverse effect associated with these drugs is hypertension, due to decreased nitric oxide signaling, increased endothelin-1 production and capillary rarefaction in the endothelium (63). Coronary microvascular dysfunction caused by loss of vascular pericytes has been reported in in vivo studies of sunitinib (64).
In addition to the anti-VEGF-mediated hypertension, TKIs may also cause LV dysfunction. Eleven percent of patients receiving sunitinib developed CHF or a significant decrease in LVEF (63). The potential for cardiotoxicity exists for many other agents of this class because the pathways targeted by these compounds are important not only in malignant cells but also in heart and endothelial cells (61).
BCR-Abl kinase is a tyrosine kinase target in chronic myelogenous leukemia. Several TKIs including imatinib, dasatinib, erlotinib and nilotinib have targeted this kinase. These agents have been reported to cause pulmonary hypertension and thromboembolism. Unlike other chemotherapeutic agents, this group of TKIs is given for an indefinite period to suppress chronic myelogenous leukemia rather than being given for a finite period of time.
Patients on TKI therapy should be monitored and treated for hypertension and thromboembolism. Cardiac function should be monitored in patients on sunitinib and medication should be discontinued at the appearance of significant LV dysfunction.
Taxanes
Taxanes such as paclitaxel and docetaxel are used to treat breast, lung, and ovarian cancer. These agents can produce ischemia, arrhythmias and CHF (65,66). Up to 5% of patients receiving docetaxel have been reported to experience myocardial ischemia. Taxanes can impair normal microtubular transport systems in cardiomyocytes, which can impair the storage and mobilization of free fatty acids from the cytosolic pool to the mitochondria (65). Taxanes potentiate the cardiotoxicity of anthracyclines and trastuzumab. Cardiac function should be monitored closely in patients receiving combinations of taxanes, anthracyclines and or trastuzumab. Molecular imaging with radiolabeled free fatty acids may provide further insight into the mechanism of impaired cardiac fatty acid metabolism and cardiotoxicity.
Interleukin-2 therapy
High-dose interleukin-2 therapy is used for renal cell carcinoma and malignant melanomas. This is associated with the capillary leak syndrome, hypotension, tachycardia and myocardial ischemia. Early studies of this agent demonstrated an incidence of myocardial infarction of 2–4% (67,68), although subsequent studies showed much lower rates of myocardial infarction. Cardiac examination should be performed prior to and during the course of high dose interleukin therapy.
Checkpoint inhibitor immune therapy
The use of recently developed checkpoint inhibitors has significantly improved the clinical outcome in several tumors such as metastatic melanoma, lung, renal, and bladder cancers (69-75). Several other cancers are also under study. Checkpoint inhibitors block the mechanism used by the tumors to evade activated T lymphocytes from binding and killing the tumor cells (69,70). This system comprises of PD-1/PD-L1, PD-L2 system. PD-1 is expressed on activated T cells, natural killer cells, macrophages and B cells. PD-L1 and PD-L2 are its natural substrates present in a subset of macrophages and a variety of peripheral tissue. PD-1/PD-L1, PD-L2 binding negatively regulates the immune responses and controls and regulates the inflammatory responses in the tissues. Tumors use this mechanism to evade body’s immune response by overexpressing PD-L1 and PD-L2 ligands. Blockade of PD1/PD-L1, PD-L2 interaction by antibodies directed against any of these targets, results in unleashing of body’s immune response to the tumors. Pembrolizumab (Keytruda) and nivolumab (Opdivo) are antibodies directed against PD-1, which are used to treat metastatic melanoma and several other cancers. Several other antibodies targeting PD-1/PD-L1, PD-L2 are under development and likely to be available in near future. Cytotoxic T lymphocyte-associated antigen 4 (CTLA-4) is another mechanism protecting the tumors from T lymphocytes. T lymphocytes can recognize and destroy cancer cells. However, an inhibitory mechanism interrupts this destruction. Cancer cells produce antigens, which are used by the immune system to identify them. These antigens are recognized by the dendritic cells. Dendritic cells present these antigens to cytotoxic T lymphocytes in the lymph nodes for them to identify and destroy the cancer cells. However, along with the antigens, the dendritic cells also present an inhibitory signal to the cytotoxic lymphocytes that binds to a receptor, called CTLA-4, on the tumor cells that turns off the cytotoxic reaction. This allows the cancer cells to evade cytotoxic T cells. Antibodies directed against the CTLA-4 receptor block this inhibitory signal and promoted cytotoxic T cell mediated tumor cell lysis (71). Ipilimumab (Yervoy) is an antibody against CTLA-4.
PD-1 antibody and CTLA-4 antibodies are synergistic and often used in combination, which enhances the therapeutic efficacy of the either agent. Unfortunately, these immune modulatory agents, particularly when used in combination also cause a variety of immune mediated diseases, including thyroiditis and myositis. Thyroiditis can result in thyroid storm, atrial fibrillation, rapid ventricular rates and heart failure (72,73). Recently two cases of fatal myocarditis with the combined use of ipilimumab and nivolumab for metastatic melanoma have been described (74). Both patient developed myositis, rhabdomyolysis and myocarditis and fatal arrhythmias and were found to have intense myocardial infiltration by T and B lymphocytes. So far, no specific guidelines are available for the monitoring and treating cardiotoxicity of these agents. With an anticipated increase in the use of these agents and a wider availability of more agents of this class, more cases of cardiotoxicity due to thyroid storm or due to direct myocardial leukocytic infiltration are expected and algorithms for monitoring for cardiotoxicity are required.
Angiogenesis inhibitors
Monoclonal antibody bevacizumab and several TKIs, such as sunitinib, inhibit angiogenesis in tumors. This has resulted in significant improvements in survival for certain forms of cancer. As discussed earlier, these agents also have adverse cardiovascular effects based on their inhibition of the VEGF receptor and the platelet derived growth factor receptor. Such side effects could alter the coronary flow reserve, or the resting blood flow to the myocardium (75). Therefore, patients receiving antiangiogenic therapies may benefit from study of myocardial perfusion and coronary flow reserve prior to initiating therapy with these agents (76).
Conclusions
The development of newer and more potent chemotherapeutic agents has improved the outcome of patients with cancers. However, many of these agents are associated with significant short term as well as long-term toxicities. Cardiotoxicity is a serious concern with several of the established as well as newer anticancer agents. Cardio-oncology has emerged as a sub-specialty to meet the challenges posed by a complex interaction between cancer and the cardiovascular system and the cardiotoxicity of established and newly developed cancer therapeutic agents. The traditional emphasis in this field has been a careful serial monitoring of the cardiovascular system for an early detection of the cardiotoxicity and therapy interruption to prevent more serious and life-threatening cardiotoxicity with continued therapy. However, with better understanding of the precise cellular, biochemical and genomic mechanism(s) of cardiotoxicity, it may be possible to identify and predict individual susceptibility to cardiotoxicity prior to starting therapy and tailor therapeutic regimens based upon tumor sensitivity and patients’ susceptibility to toxicity.
Acknowledgements
None.
Footnote
Conflicts of Interest: The authors have no conflicts of interest to declare.
References
- Siegel RL, Miller KD, Jemal A. Cancer statistics 2016. CA Cancer J Clin 2016;66:7-30. [Crossref] [PubMed]
- Reulen RC, Winter DL, Frobisher C, et al. Long-term cause-specific mortality among survivors of childhood cancer. JAMA 2010;304:172-9. [Crossref] [PubMed]
- Lipshultz SE, Adams MJ, Colan SD, et al. Long-term cardiovascular toxicity in children, adolescents, and young adults who receive cancer therapy: pathophysiology, course, monitoring, management, prevention, and research directions: a scientific statement from the American Heart Association. Circulation 2013;128:1927-95. [Crossref] [PubMed]
- Albini A, Pennesi G, Donatelli F, et al. Cardiotoxicity of anticancer drugs: the need for cardio-oncology and cardio-oncological prevention. J Natl Cancer Inst 2010;102:14-25. [Crossref] [PubMed]
- Schwartz RG, Jain D, Storozynsky E. Traditional and novel methods to assess and prevent chemotherapy-related cardiac dysfunction noninvasively. J Nucl Cardiol 2013;20:443-64. [Crossref] [PubMed]
- Russell RR, Alexander J, Jain D, et al. The role and clinical effectiveness of multimodality imaging in the management of cardiac complications of cancer and cancer therapy. J Nucl Cardiol 2016;23:856-84. [Crossref] [PubMed]
- Volkova M, Russell R 3rd. Anthracycline cardiotoxicity: prevalence, pathogenesis and treatment. Curr Cardiol Rev 2011;7:214-20. [Crossref] [PubMed]
- Bristow MR, Thompson PD, Martin RP, et al. Early anthracycline cardiotoxicity. Am J Med 1978;65:823-32. [Crossref] [PubMed]
- Cardinale D, Colombo A, Bacchiani G, et al. Early Detection of Anthracycline Cardiotoxicity and Improvement With Heart Failure Therapy. Circulation 2015;131:1981-8. [Crossref] [PubMed]
- Xu X, Persson HL, Richardson DR. Molecular pharmacology of the interaction of anthracyclines with iron. Mol Pharmacol 2005;68:261-71. [PubMed]
- Zhang S, Liu X, Bawa-Khalfe T, et al. Identification of the molecular basis of doxorubicin-induced cardiotoxicity. Nat Med 2012;18:1639-42. [Crossref] [PubMed]
- Dazzi H, Kaufmann K, Follath F. Anthracycline-induced acute cardiotoxicity in adults treated for leukaemia. Analysis of the clinico-pathological aspects of documented acute anthracycline-induced cardiotoxicity in patients treated for acute leukaemia at the University Hospital of Zurich, Switzerland, between 1990 and 1996. Ann Oncol 2001;12:963-6. [Crossref] [PubMed]
- Lipshultz SE, Colan SD, Gelber RD, et al. Late cardiac effects of doxorubicin therapy for acute lymphoblastic leukemia in childhood. N Engl J Med 1991;324:808-15. [Crossref] [PubMed]
- Lipshultz SE, Lipsitz SR, Sallan SE, et al. Chronic progressive cardiac dysfunction years after doxorubicin therapy for childhood acute lymphoblastic leukemia. J Clin Oncol 2005;23:2629-36. [Crossref] [PubMed]
- Von Hoff DD, Layard MW, Basa P, et al. Risk factors for doxorubicin-induced congestive heart failure. Ann Intern Med 1979;91:710-7. [Crossref] [PubMed]
- Panjrath GS, Patel V, Valdiviezo CI, et al. Potentiation of Doxorubicin cardiotoxicity by iron loading in a rodent model. J Am Coll Cardiol 2007;49:2457-64. [Crossref] [PubMed]
- Miranda CJ, Makui H, Soares RJ, et al. Hfe deficiency increases susceptibility to cardiotoxicity and exacerbates changes in iron metabolism induced by doxorubicin. Blood 2003;102:2574-80. [Crossref] [PubMed]
- Alexander J, Dainiak N, Berger HJ, et al. Serial assessment of doxorubicin cardiotoxicity with quantitative radionuclide angiocardiography. N Engl J Med 1979;300:278-83. [Crossref] [PubMed]
- Choi BW, Berger HJ, Schwartz PE, et al. Serial radionuclide assessment of doxorubicin cardiotoxicity in cancer patients with abnormal baseline resting left ventricular performance. Am Heart J 1983;106:638-43. [Crossref] [PubMed]
- Mitani I, Jain D, Joska TM, et al. Doxorubicin cardiotoxicity: prevention of congestive heart failure with serial cardiac function monitoring with equilibrium radionuclide angiocardiography in the current era. J Nucl Cardiol 2003;10:132-9. [Crossref] [PubMed]
- Schwartz RG, McKenzie WB, Alexander J, et al. Congestive heart failure and left ventricular dysfunction complicating doxorubicin therapy: Seven-year experience using serial radionuclide angiocardiography. Am J Med 1987;82:1109-18. [Crossref] [PubMed]
- Carver JR, Shapiro CL, Ng A, et al. American Society of Clinical Oncology clinical evidence review on the ongoing care of adult cancer survivors: cardiac and pulmonary late effects. J Clin Oncol 2007;25:3991-4008. [Crossref] [PubMed]
- Wojnowski L, Kulle B, Schirmer M, et al. NAD(P)H oxidase and multidrug resistance protein genetic polymorphisms are associated with doxorubicin-induced cardiotoxicity. Circulation 2005;112:3754-62. [Crossref] [PubMed]
- Mackey JR, Clemons M, Cote MA, et al. Cardiac management during adjuvant trastuzumab therapy: recommendations of the Canadian Trastuzumab Working Group. Curr Oncol 2008;15:24-35. [Crossref] [PubMed]
- Thavendiranathan P, Grant AD, Negishi T, et al. Reproducibility of echocardiographic techniques for sequential assessment of left ventricular ejection fraction and volumes: application to patients undergoing cancer chemotherapy. J Am Coll Cardiol 2013;61:77-84. [Crossref] [PubMed]
- Walker J, Bhullar N, Fallah-Rad N, et al. Role of three-dimensional echocardiography in breast cancer: comparison with two-dimensional echocardiography, multiple-gated acquisition scans, and cardiac magnetic resonance imaging. J Clin Oncol 2010;28:3429-36. [Crossref] [PubMed]
- Marchandise B, Schroeder E, Bosly A, et al. Early detection of doxorubicin cardiotoxicity: interest of Doppler echocardiographic analysis of left ventricular filling dynamics. Am Heart J 1989;118:92-8. [Crossref] [PubMed]
- Stoddard MF, Seeger J, Liddell NE, et al. Prolongation of isovolumetric relaxation time as assessed by Doppler echocardiography predicts doxorubicin-induced systolic dysfunction in humans. J Am Coll Cardiol 1992;20:62-9. [Crossref] [PubMed]
- Tassan-Mangina S, Codorean D, Metivier M, et al. Tissue Doppler imaging and conventional echocardiography after anthracycline treatment in adults: early and late alterations of left ventricular function during a prospective study. Eur J Echocardiogr 2006;7:141-6. [Crossref] [PubMed]
- Mor-Avi V, Lang RM. Is echocardiography reliable for monitoring the adverse cardiac effects of chemotherapy? J Am Coll Cardiol 2013;61:85-7. [Crossref] [PubMed]
- Thavendiranathan P, Poulin F, Lim KD, et al. Use of myocardial strain imaging by echocardiography for the early detection of cardiotoxicity in patients during and after cancer chemotherapy: a systematic review. J Am Coll Cardiol 2014;63:2751-68. [Crossref] [PubMed]
- Sawaya H, Sebag IA, Plana JC, et al. Early detection and prediction of cardiotoxicity in chemotherapy-treated patients. Am J Cardiol 2011;107:1375-80. [Crossref] [PubMed]
- Sawaya H, Sebag IA, Plana JC, et al. Assessment of echocardiography and biomarkers for the extended prediction of cardiotoxicity in patients treated with anthracyclines, taxanes, and trastuzumab. Circ Cardiovasc Imaging 2012;5:596-603. [Crossref] [PubMed]
- Cardinale D, Sandri MT. Role of biomarkers in chemotherapy-induced cardiotoxicity. Prog Cardiovasc Dis 2010;53:121-9. [Crossref] [PubMed]
- Dolci A, Dominici R, Cardinale D, et al. Biochemical markers for prediction of chemotherapy-induced cardiotoxicity: systematic review of the literature and recommendations for use. Am J Clin Pathol 2008;130:688-95. [Crossref] [PubMed]
- Fallah-Rad N, Walker JR, Wassef A, et al. The Utility of Cardiac Biomarkers, Tissue Velocity and Strain Imaging, and Cardiac Magnetic Resonance Imaging in Predicting Early Left Ventricular Dysfunction in Patients With Human Epidermal Growth Factor Receptor II-Positive Breast Cancer Treated With Adjuvant Trastuzumab Therapy. J Am Coll Cardiol 2011;57:2263-70. [Crossref] [PubMed]
- Jurcut R, Wildiers H, Ganame J, et al. Strain rate imaging detects early cardiac effects of pegylated liposomal Doxorubicin as adjuvant therapy in elderly patients with breast cancer. J Am Soc Echocardiogr 2008;21:1283-9. [Crossref] [PubMed]
- Lipshultz SE, Miller TL, Lipsitz SR, et al. Continuous Versus Bolus Infusion of Doxorubicin in Children With ALL: Long-term Cardiac Outcomes. Pediatrics 2012;130:1003-11. [Crossref] [PubMed]
- Palmieri C, Misra V, Januszewski A, et al. Multicenter experience of nonpegylated liposomal doxorubicin use in the management of metastatic breast cancer. Clin Breast Cancer 2014;14:85-93. [Crossref] [PubMed]
- Children’s Oncology Group Long-term Follow up Guidelines for Survivors of Childhood, Adolescent and Young Adult Cancer. Available online: www.survivorshipguidelines.org/pdf/COG_LTFU_Guidelines_Appendix1_v4.pdf
- Cardinale D, Colombo A, Lamantia G, et al. Anthracycline-induced cardiomyopathy: clinical relevance and response to pharmacologic therapy. J Am Coll Cardiol 2010;55:213-20. [Crossref] [PubMed]
- Voigt J, Sasha John M, Taylor A, et al. A reevaluation of the costs of heart failure and its implications for allocation of health resources in the United States. Clin Cardiol 2014;37:312-21. [Crossref] [PubMed]
- Tebbi CK, London WB, Friedman D, et al. Dexrazoxane-associated risk for acute myeloid leukemia/myelodysplastic syndrome and other secondary malignancies in pediatric Hodgkin's disease. J Clin Oncol 2007;25:493-500. [Crossref] [PubMed]
- Perik PJ, de Korte MA, van Veldhuisen DJ, et al. Cardiotoxicity associated with the use of trastuzumab in breast cancer patients. Expert Rev Anticancer Ther 2007;7:1763-71. [Crossref] [PubMed]
- Ewer MS, Vooletich MT, Durand JB, et al. Reversibility of trastuzumab-related cardiotoxicity: new insights based on clinical course and response to medical treatment. J Clin Oncol 2005;23:7820-6. [Crossref] [PubMed]
- Ewer SM, Ewer MS. Cardiotoxicity profile of trastuzumab. Drug Saf 2008;31:459-67. [Crossref] [PubMed]
- Hedhli N, Dobrucki LW, Kalinowski A, et al. Endothelial-derived neuregulin is an important mediator of ischaemia-induced angiogenesis and arteriogenesis. Cardiovasc Res 2012;93:516-24. [Crossref] [PubMed]
- Panjrath GS, Jain D. Trastuzumab-induced cardiac dysfunction. Nucl Med Commun 2007;28:69-73. [Crossref] [PubMed]
- Gianni L, Pienkowski T, Im YH, et al. Efficacy and safety of neoadjuvant pertuzumab and trastuzumab in women with locally advanced, inflammatory, or early HER2-positive breast cancer (NeoSphere): a randomised multicentre, open-label, phase 2 trial. Lancet Oncol 2012;13:25-32. [Crossref] [PubMed]
- Blackwell KL, Burstein HJ, Storniolo AM, et al. Randomized study of Lapatinib alone or in combination with trastuzumab in women with ErbB2-positive, trastuzumab-refractory metastatic breast cancer. J Clin Oncol 2010;28:1124-30. [Crossref] [PubMed]
- Behr TM, Behe M, Wormann B. Trastuzumab and breast cancer. N Engl J Med 2001;345:995-6. [Crossref] [PubMed]
- McGale P, Darby SC, Hall P, et al. Incidence of heart disease in 35,000 women treated with radiotherapy for breast cancer in Denmark and Sweden. Radiother Oncol 2011;100:167-75. [Crossref] [PubMed]
- Darby SC, Ewertz M, McGale P, et al. Risk of ischemic heart disease in women after radiotherapy for breast cancer. N Engl J Med 2013;368:987-98. [Crossref] [PubMed]
- Marks LB, Yu X, Prosnitz RG, et al. The incidence and functional consequences of RT-associated cardiac perfusion defects. Int J Radiat Oncol Biol Phys 2005;63:214-23. [Crossref] [PubMed]
- Correa CR, Litt HI, Hwang WT, et al. Coronary artery findings after left-sided compared with right-sided radiation treatment for early-stage breast cancer. J Clin Oncol 2007;25:3031-7. [Crossref] [PubMed]
- Nilsson G, Holmberg L, Garmo H, et al. Distribution of coronary artery stenosis after radiation for breast cancer. J Clin Oncol 2012;30:380-6. [Crossref] [PubMed]
- de Forni M, Malet-Martino MC, Jaillais P, et al. Cardiotoxicity of high-dose continuous infusion fluorouracil: a prospective clinical study. J Clin Oncol 1992;10:1795-801. [Crossref] [PubMed]
- Saif MW, Shah MM, Shah AR. Fluoropyrimidine-associated cardiotoxicity: revisited. Expert Opin Drug Saf 2009;8:191-202. [Crossref] [PubMed]
- Berliner S, Rahima M, Sidi Y, et al. Acute coronary events following cisplatin-based chemotherapy. Cancer Invest 1990;8:583-6. [Crossref] [PubMed]
- Frickhofen N, Beck FJ, Jung B, et al. Capecitabine can induce acute coronary syndrome similar to 5-fluorouracil. Ann Oncol 2002;13:797-801. [Crossref] [PubMed]
- Force T, Kolaja KL. Cardiotoxicity of kinase inhibitors: the prediction and translation of preclinical models to clinical outcomes. Nat Rev Drug Discov 2011;10:111-26. [Crossref] [PubMed]
- Schmidinger M, Zielinski CC, Vogl UM, et al. Cardiac toxicity of sunitinib and sorafenib in patients with metastatic renal cell carcinoma. J Clin Oncol 2008;26:5204-12. [Crossref] [PubMed]
- Chu TF, Rupnick MA, Kerkela R, et al. Cardiotoxicity associated with tyrosine kinase inhibitor sunitinib. Lancet 2007;370:2011-9. [Crossref] [PubMed]
- Chintalgattu V, Rees ML, Culver JC, et al. Coronary microvascular pericytes are the cellular target of sunitinib malate-induced cardiotoxicity. Sci Transl Med 2013;5:187ra69. [Crossref] [PubMed]
- Saito K, Takeda K, Imanaka-Yoshida K, et al. Assessment of fatty acid metabolism in taxan-induced myocardial damage with iodine-123 BMIPP SPECT: comparative study with myocardial perfusion, left ventricular function, and histopathological findings. Ann Nucl Med 2003;17:481-8. [Crossref] [PubMed]
- Rowinsky EK, McGuire WP, Guarnieri T, et al. Cardiac disturbances during the administration of taxol. J Clin Oncol 1991;9:1704-12. [Crossref] [PubMed]
- Schwartz RN, Stover L, Dutcher J. Managing toxicities of high-dose interleukin-2. Oncology (Williston Park) 2002;16:11-20. [PubMed]
- Dutcher J, Atkins MB, Margolin K, et al. Kidney cancer: the Cytokine Working Group experience (1986-2001): part II. Management of IL-2 toxicity and studies with other cytokines. Med Oncol 2001;18:209-19. [Crossref] [PubMed]
- Wolchok JD. PD-1 blockers. Cell 2015;162:937. [Crossref] [PubMed]
- Sunshine J, Taube JM. PD-1/PD-L1 inhibitors. Curr Opin Pharmacol 2015;23:32-8. [Crossref] [PubMed]
- Lee JY, Lee HT, Shin W, et al. Structural basis of checkpoint blockade by monoclonal antibodies in cancer immunotherapy. Nat Commun 2016;7:13354. [Crossref] [PubMed]
- Ryder M, Callahan M, Postow MA, et al. Endocrine-related adverse events following ipilimumab in patients with advanced melanoma: a comprehensive retrospective review from a single institution. Endocr Relat Cancer 2014;21:371-81. [Crossref] [PubMed]
- Yu C, Chopra IJ, Ha E. A novel melanoma therapy stirs up a storm: ipilimumab-induced thyrotoxicosis. Endocrinol Diabetes Metab Case Rep 2015;2015:140092. [PubMed]
- Johnson DB, Balko JM, Compton ML, et al. Fulminant myocarditis with combined checkpoint inhibitors. N Engl J Med 2016;375:1749-55. [Crossref] [PubMed]
- Moslehi JJ. Cardiovascular toxic effects of targeted cancer therapies. N Engl J Med 2016;375:1457-67. [Crossref] [PubMed]
- Schindler TH, Schelbert HR, Quercioli A, et al. Cardiac PET imaging for the detection and monitoring of coronary artery disease and microvascular health. JACC Cardiovasc Imaging 2010;3:623-40. [Crossref] [PubMed]