Dendritic cell therapy in melanoma
Introduction
Dendritic cells (DCs) are central cells of the immune system at the interphase of innate and specific immunity, the latter also known as adaptive immunity. Therefore, they stimulate naive T cells to initiate the immune responses, but also, they interact with and influence the responses of other innate immune cells, such as macrophages or natural killer cells (NK). To initiate the immune responses, DC cells encounter and degrade antigens to small molecules called epitopes. Next, these epitopes bind to either class I major histocompatibility molecules (MHC-I) or class II MHC (MHC-II) to present them to CD8+ T cells (MHC-I) or to CD4+ T cells (MHC-II) by interaction with the T cell receptor. This whole process of antigen processing and presentation gives a first signal to T cells (signal 1). This antigen specific signal 1 in T cells is not sufficient for T cell activation. T cell activation requires that CD28 molecules of T cells, recognizes the co-stimulatory molecules CD80 (B-7.1) or CD86 (B-7.2) in DC (signal 2). Signals 1 and 2 induce the production of IL-2 by T cells and as a feedback mechanism, the expression in these cells of two molecules, the α chain of IL-2 receptor (CD25) and CD40L, the ligand of CD40. The system CD40/CD40L amplifies antigen presentation and signal 2. The binding of IL-2 to its receptor (CD25/CD122) activates mTOR, the target for rapamycin (signal 3) that results in T cell clonal proliferation and generation of effector cells such as cytotoxic T cells (1). There are two negative regulators of this overall process in T cells: (I) CTLA-4 in T cells that, if present, binds to CD80 or CD86 and stops the activation of T cells, avoiding over-activation of the immune system and (II) the cell death program antigen 1 or PD-1 also in T cells that, if present, binds to their ligands in DC, PD-L1 or PD-L2 molecules, blocking the system (Figure 1).
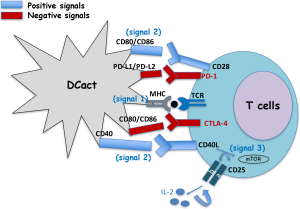
The function of DC in vivo is the surveillance of the organism to phagocyte and destroy intruders such as pathogens or tumour cells. They can act in two processes of the immune responses, in the priming phase as well as in the effector phase. In the priming phase, DC recognize molecular patterns on pathogens or in tumour cells using different Toll-like receptors (TLR) that activates signalling pathways that remodel cell surface molecules and produce different types of cytokines. Cell surface molecules suffering deep remodelling are those involved in antigen presentation, MHC-I, MHC-II molecules and co-stimulatory molecules, CD40, CD80 and CD86. In this regard, mature DC of any source, either plasmacytoid DC or monocyte derived DC (MoDC), show significant expression of MHC-I or MHC-II molecules but low or absent expression of co-stimulatory molecules. While activated MoDC present high expression of molecules involved in antigen presentation, MHC-I, MHC-II and high expression of all or some co-stimulatory molecules, CD40, CD80 or CD86. Also mature or activated MoDC produce different types of cytokines. The cytokines produced by DC depend on the signals they receive from the environment, being these cytokines classified as Th1 cytokines/chemokines with a pro-inflammatory pattern, TNF-α, MCP-1, IL-1β, IFN-α/β and IL-12p40/IL-12p70 or Th2 cytokines with predominant anti-inflammatory profiles as IL-6, IL-10 or TGF-β (Figure 2). However, the MoDC cytokine profile in each situation varies and the levels of each one of these Th1 or Th2 patterns determine immune-stimulation or immune-suppression profile, being in general Th1 profiles of MoDC considered immune stimulatory and Th2 profiles, immune suppressor. Mature MoDC in general, produce a mixture of Th1 and Th2 cytokines, while activated MoDC produced almost exclusively Th1 cytokines.
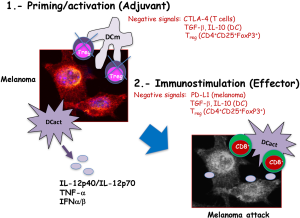
Next, MoDC can also act in the effector phase of the immune response, either CD4+ or CD8+ T cells, that are usually IFN-γ producers. The type of predominant effector cell would depend on the pathogen or tumour cell. In general, pathogens activate both CD4+ and CD8+ effector T cells that destroy the pathogens either by induction of neutralizing antibodies or activating cytotoxic T cells that lysed those cells infected with the pathogens. In the case of tumour cells, CD8+ T cells are mainly stimulated by DC as well as NK cells in order they kill directly the tumour cells (2).
Vaccines
Cancer vaccines are expected to activate the immune system as adjuvants and deliver antigens to T cells. Cancer vaccines function different than prophylactic vaccines as they are expected to expand only cytotoxic CD8+ T cells to cause tumour regression. It is suitable that cancer vaccines also decrease the number or activating responses of tumour immunosuppressor signals of T regulatory (Treg) cells or myeloid derived suppressor cells (MDSC). The different types of cancer vaccines approved by FDA are autologous, peptide, dendritic or vector-based vaccines.
Autologous vaccines are composed of tumour cells extracted from each patient. They may contain tumour-associated antigens (TAA) of each tumour but whole cells are not different than normal cells and therefore, the response they generate might not be specific enough to cause impact in the tumour size. For this reason, autologous vaccines are modified to improve their immunogenicity.
A variation of autologous vaccines is allogenic whole tumour cell vaccines expressing GM-CSF and irradiated (GVAX) (1). This approach uses a tumour cell line that expresses a plurality of shared tumour antigens such as tyrosinase, gp100, MART-1/melan-A, and MAGE-A3 and genetically modified to secrete GM-CSF, a potent adjuvant that induces DC maturation. Peptide vaccines target the above-mentioned tumour antigens but identifying those peptides unique to cancer cells. While generating specific immune responses, they do not implement survival.
Vector-based vaccines, either bacterial or viruses induce specific as well as substantial immune responses that affect clinical results. They are based in the assumption that the primary function of the immune system is to protect against foreign pathogens. However, they present several disadvantages, as the immune responses against the vector are restricted and they cause pathogenesis or be mutagenized. In this regard, attenuated mutants of Listeria monocytogenes either deficient in two virulence factors ActA and Internalin B, known as LADD mutants (Aduro Biotech) (3,4), or deficient in a partial sequence of the main virulence factor listeriolysin O (LLO) (Advaxis) (5), are constructed to express different tumour antigens, such as prostate PSV or mesothelin (CRS-207) alone or in combination with GVAX (6), supposed a great expectation as these bacteria induced not only strong innate immune responses but also cytotoxic immune responses. However, recently CRS-207 in combination with GVAX failed in trials against pancreatic cancer, getting patients worse and develop symptoms associated with the bacterial pathogen.
Dendritic vaccines are potent antigen-presenting cells with high capacity to induce T cell immune responses through eliciting pro-inflammatory cytokine responses and stimulating cytotoxic T cells. However, tumours are immunosuppressive environments and might damp the effectiveness of DC. DC are in very low frequencies in the peripheral blood and tissues and therefore, they should be expanded either in vivo or ex vivo in order to use them as therapies. The first developing approach using DC vaccines consisted on infusing immature DC that collected antigens in vivo. Next, MoDC were harvested from patients and generated at large scale to yield sufficient numbers of cells for clinical application. Next, MoDC were pulsed ex vivo with TAA, tumour lysates, whole tumour cells, tumour RNA or apoptotic tumour cells or bacteria derived reagents to induce MoDC maturation. Mature MoDC are re-infused back in patients with the purpose to induce tumour-specific immune responses.
Current ex vivo expansion of MoDC is performed after incubation with GM-CSF and IL-4 to induce full maturation of DC. The procedure implies first Ficoll isolation of leukocytes, CD45+ cells. Second, monocytes are selected as CD14+ positive cells in MACS columns (MACSTM, Myltenyi) to provide large numbers of mature MoDC. Mature MoDC are next activated with different agents such as bacteria as Mycobacterium BCG strain (BCG) or Listeria monocytogenes (Listeria) that signal through TLR-2 or TLR-4 or bacterial oligonucleotides (ODN) with CpG motifs (Figure 2). Trials with MoDC have used indistinctively either mature or activated DC, but they produce different cytokines that can generate different immune responses. Therefore, it is important DC vaccines produce Th1 cytokines with tumorigenic abilities, such as TNF-α or IL-12p40/IL-12p70, otherwise they would not induce cytotoxic immune responses and be ineffective as anti-tumour therapies. In this regard, ex vivo mature MoDC obtained from patients with malignancies produced predominantly Th2 phenotypes (7). However, if they are activated with adjuvants such as Listeria peptides in nanoparticle platforms, MoDC of these patients shifted their Th2 immune responses towards Th1 profiles (8).
DC can also be activated in vivo requiring the use of non-specific adjuvants such as GM-CSF, CpG, stimulating cytokines as IFN-α/β, STING-activating cyclic dinucleotides (9,10), bacteria as BCG or Listeria or bacterial products. In this regard, IFN-α is a common adjuvant therapy applied in melanoma patients after surgery to prevent or delay tumour relapses (11-13). Bacterial toxins can also present anti-tumour activity such as LLO, a pore-forming cytotoxin of Listeria and high capacity to expand non-specific immune responses, binds to TLR-2 costimulatory molecules as CD14 and act as potent adjuvant (14).
DC vaccines in melanoma therapy
DC vaccines have been used to activate the immune system in autologous and allogenic vaccination strategies using peptides or tumour lysates (15-18). However, the response rates using DC vaccines have been usually low ranging from 10% to 20%, although they are the only immunotherapy so far that increases patient survival rates. DC vaccines can stimulate the immune system to respond to one specific antigen or carbohydrate moiety or to elicit an immune response against multiple tumour antigens, incorporating either allogenic whole cells, autologous tumour cells, recombinant proteins or tumour lysates. Moreover, DC vaccines are safe immunotherapies compared with other adjuvants as IFN-α, or immune check point inhibitors that cause immunological adverse events and have low number of responders.
In general, the success of DC vaccines against melanoma is explained by their participation in two stages of the induction of immune responses (Figure 3). First, as adjuvants they act in the priming stage of the immune responses, decreasing the activation of Treg cells localized in the tumour environment. Moreover, as mentioned before, activated DC vaccines produce large amounts of TNF-α, IFN-α/β and of IL-12p40/IL-12p70 cytokines with anti-neoplastic abilities. These pro-inflammatory cytokines act as feedback mechanisms, activating cytotoxic CD8+ T cells. Second, as immune stimulators in the effector phase of the immune responses, activated DC interacted efficiently with cytotoxic CD8+ T cells and expanded melanoma specific CD8+ T cells. Finally, melanoma specific CD8+ T cells can attack melanoma and benefit tumour regression.
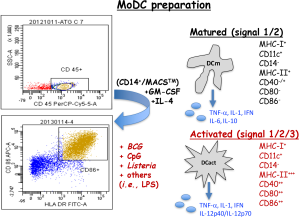
Since the approval in 2010 of the first DC vaccine for prostate cancer, sipuleucel-T (Provenge), this early design has improved currently (19) and DC vaccines have raised a huge focus in melanoma treatment. In this regard, trials for human leukocyte antigen (HLA)-A2+ advanced melanomas have been conducted (20,21), presenting significant clinical responses, albeit the number of patients benefiting of these approaches were small. DC vaccines embedded in matrices with thrombin and fibrinogen were used to increase host resistance of patients with melanoma, because they activated DC and induced high production of IFN-γ. This approach was also used in experimental models of melanoma and observed great reduction of tumour responses. In fact, mice presented 65% of tumour remission and developed effective immune responses. These DC matrices were valid approaches that interacted with immune cells, including lymphocytes (22). One of the first trials using MoDC pulsed with GM-CSF and IL-4, evaluated sixteen patients of melanoma treated with autologous MoDC pulsed with a cocktail of specific MART-1, MAGE-1, MAGE-3, gp100 or tyrosinase peptides to fit into especial class I HLA molecules of the patients. This trial also included KLH as an antigen that induced non-specific CD4+ T cells to interact with CD8+ T cells and DC vaccination was in lymph nodes non-involved in the tumour. Five out of sixteen patients presented tumour regression and two completed responses that lasted over fifteen months (15).
Later on, similar approaches were used with a similar peptide cocktail of melanoma antigens, MART-1, Melan-A, gp100 or tyrosinase to load MoDC in cancer vaccines in phase I and phase II trials (20,21). In fact, peptides, tyrosinase386-406, MART-1/Melan-A51-73 or gp10044-59 showed high induction of immune responses. Twenty-three patients out of thirty-seven showed antibody and T cell responses, two patients antibody response only and five showed T cell response only.
Electroporation of DC with mRNA encoding different activating markers such as CD40L, CD70 and a constitutive active form of TLR-4, referred as TriMix (23) constituted a potent DC-based cellular therapy.
Other approaches to activate DC have been the use of whole bacteria such as attenuated Mycobacterium BCG, Salmonella or Listeria monocytogenes as non-specific adjuvants that efficiently activate DC (3). In fact, their use is based in the first reports of immunotherapy by William Coley that presented the utility of bacteria and bacterial products in the treatment of cancer using Staphylococcus pyogenes to treat his patients. Nowadays, the use of attenuated Mycobacterium strains as BCG is a frontline treatment in several melanoma trials (24,25) as well as other tumours as bladder cancer. However, the action of BCG implies non-specific immunostimulation of the immune responses and not direct killing of cancer cells. A different action supposes the use of Listeria against melanoma. This rare human bacterial pathogen induced apoptosis of melanoma cells, even at very low doses and expands CD8+ T cell cytotoxic responses, benefiting also the expansion of melanoma specific cytotoxic responses (26). Moreover, DC loaded with LLO91–99 peptides can act as recall antigens that pre-condition the vaccination sites and induced anti-tumour immune responses (27). This effect appeared similar to the action reported by the potent recall antigen and bacterial toxin, tetanus toxoid loaded into DC vaccines, that induced effect responses against glioblastoma (28).
Another highly active area is the use of biomaterials or nanoparticles to improve DC and T cell function at various tissues for the adjuvant treatment of melanoma (29-32). The use of biomaterials and nanomaterials implies solving several issues with adjuvants as the short half-lives of antigens plus adjuvants or the loss of viability upon in vivo transplantation of activated cell products. Therefore, biomaterials are used to extend the duration of the immune responses as well as controlling antigen localization in tissues or targeting to DC cells. The use of scaffolds of macroporous poly(lactide-co-glycolide) (PLG) matrices can regulate the trafficking and activation of DC in the tissues (33). These matrices can be implanted in vivo and potentiate the immune responses. They are fabricated with GM-CSF, a CpG ODN and tumour lysate. When they were implanted in experimental mice, they recruited DC in a melanoma model and increase the cytotoxic responses, causing melanoma regression (34). Other types of materials are gold glyconanoparticles that bear carbohydrates in their structures and therefore are targeted to DC and other antigen presenting cells as macrophages (29,35).
DC loaded with peptides of bacterial toxins able to elicit anti-tumour immune responses as 91–99 LLO peptide, are able to act as efficient adjuvants and induce strong innate and cytotoxic responses, expanding melanoma specific cytotoxic responses and preventing melanoma growth, dissemination and formation of metastasis (27). LLO91–99 peptide loaded into DC is able to induce effector CD8+ T cells that localize in the tumour, inducing apoptosis of melanoma. These DC vaccines also decrease Treg in the tumours and induce NK cells, therefore, promoting anti-melanoma immune responses, but also increase the positive signals between DC and T cells and control tumour growth and dissemination.
Trials with DC vaccines for melanoma
One of the great advantages of using DC vaccines for melanoma therapy is their safety and potent ability to combine with other immunotherapies as immunological check point inhibitors, either in patients or in experimental models (36-40).
The current trials with DC vaccines for melanoma have been approved by FDA in USA as well as by the European agency of the medicaments and they are compiled in Table 1. Some of these trials involved DC vaccines and other strategies as tumour infiltrating lymphocytes (trial 2006-005238-19 in Spain) or uses electroporated DC with mRNA (trial 2011-001410-33 in Belgium) or combination with other adjuvants as pegylated IFN-α (trial 2005-002636-97 in Great Britain) or the use of the TriMix DC formula combined with ipilimumab (trial 2010-023058-35 in Belgium). Some the trials are for advanced melanoma, others for early stages melanoma IIA, IIB, IIIB, IIIC and others for uveal melanoma. In all cases, DC vaccines are prepared ex vivo using mature MoDC. A large amount of trials haw requirements of HLA-A2 expression as well as other melanoma specific antigens such as gp100 or tyrosinase (Table 1).
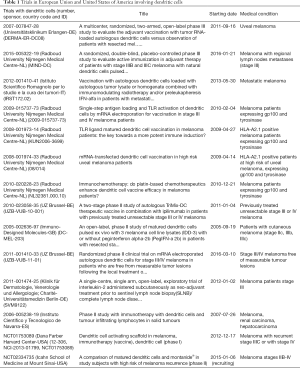
Full table
Conclusions
DC cell vaccines because of their safety and dual action into the two arms of the immune responses, the priming and effector stages are versatile immunotherapies that can be used to stimulate the immune system to fight against melanoma, both early stages and metastatic melanoma and even uveal melanoma. They also accept combination with all types of immunotherapies currently been used for melanoma, immunological check point inhibitors as ipilimumab or adjuvant therapies as pegylated IFN-a. Their high induction of TH1 cytokines as TNF-a and IL-12p40/IL-12p70 and activation of anti-melanoma cytotoxic CD8+ T cells are their best features to be used as adjuvants, with specific antigens or with polyvalent vaccines. In this regard, bacterial epitopes loaded into DC as LLO91–99 peptides are also emerging as potent anti-melanoma treatments.
Acknowledgements
Authors acknowledge the technical assistance of lab technician M. Sierra (IDIVAL) and Innovation coordinator P. Zorilla (IDIVAL).
Funding: This study is funded by MINECO (grant: SAF2012-34203) and IDIVAL to C Alvarez-Dominguez (grant: INNVAL15/01).
Footnote
Conflicts of Interest: The authors have no conflicts of interest to declare.
References
- Lipson EJ, Sharfmann WH, Chen S, et al. Safety and immunologic correlates of melanoma GVAX, a GM-CSF allogenic melanoma secreting vaccine administered in the adjuvant setting. J Transl Med 2015;13:214. [Crossref] [PubMed]
- Berraondo P, Minute L, Ajona D, et al. Innate immune mediators in cancer: between defense and resistance. Immunol Rev 2016;274:290-306. [Crossref] [PubMed]
- Shahabi V, Maciag PC, Rivera S, et al. Live, attenuated strains of Listeria and Salmonella as vaccine vectors in cancer treatment. Bioengineered Bugs 2010;1:235-43. [Crossref] [PubMed]
- Wood LM, Pan ZK, Shahabi V, et al. Listeria derived ActA is an effective adjuvant for primary and metastatic tumour immunotherapy. Cancer Immunol Immunother 2010;59:1049-58. [Crossref] [PubMed]
- Pan ZK, Weiskirch LM, Paterson Y. Regression of established B16F10 melanoma with a recombinant Listeria monocytogenes vaccine. Cancer Res 1999;59:5264-9. [PubMed]
- Le DT, Wang-Guillan A, Picozzi V, et al. Safety and survival with GVAX pancreas prime and Listeria monocytogenes–expressing mesothelin (CRS-207) boost vaccines for metastatic pancreatic cancer. J Clin Oncol 2015;33:1325-33. [Crossref] [PubMed]
- Calderon-Gonzalez R, Teran-Navarro H, Marimon JM, et al. Biomarker tools to design clinical vaccines determined from a study of annual listeriosis incidence in northern Spain. Front Immunol 2016;7:541. [Crossref] [PubMed]
- Calderon-Gonzalez R, Teran-Navarro H, Salcines-Cuevas D, et al. Gold glyconanoparticles coupled to lysteriolysin O 91-99 peptide serve as adjuvant therapy against melanoma. Nanoscale 2017. In Press. [Crossref] [PubMed]
- Dubensky TW, Kanne DB, Leong ML. Rationale, progress and development of vaccines utilizing STING-activating cyclic dinucleotide adjuvants. Ther Adv Vaccines 2013;1:131-43. [Crossref] [PubMed]
- Fu J, Kanne DB, Leong M, et al. STING agonist formulated cancer vaccines can cure established tumors resistant to PD-1 blockage. Sci Transl Med 2015;7:283ra52. [Crossref] [PubMed]
- Moreno Nogueira JA, Valero-Arbizu M, Perez-Temprano R. Adjuvant treatment of melanoma. ISRN Dermatology 2013;2013:545631. [Crossref] [PubMed]
- Parker BS, Rautela J, Hertzog PJ. Antitumor actions of interferons: implications for cancer therapy. Nat Rev Cancer 2016;16:131-44. [Crossref] [PubMed]
- Espinosa E, Soriano V, Malvehy J, et al. Treatment patterns of adjuvant interferon-α2b for high-risk melanoma: a retrospective study of the Grupo Español Multidisciplinar de Melanoma - Prima study. Melanoma Res 2016;26:278-83. [Crossref] [PubMed]
- Sun R, Liu Y. Listeriolysin O as a strong immunogenic molecule for the development of new anti-tumor vaccines. Hum Vaccin Immunother 2013;9:1058-68. [Crossref] [PubMed]
- Nestle FO, Alijagic S, Gilliet M, et al. Vaccination of melanoma patients with peptide-or tumor lysate-pulsed dendritic cells. Nat Med 1998;4:328-32. [Crossref] [PubMed]
- Butterfield LH. Dendritic cells in cancer immunotherapy clinical trials: are we making progress? Front Immunol 2013;4:454. [Crossref] [PubMed]
- Berrocal A, Cabañas L, Espinosa E, et al. Melanoma: diagnosis, staging, and treatment. Consensus group recommendations. Adv Ther 2014;31:945-60. [Crossref] [PubMed]
- de Rosa F, Ridolfi L, Ridolfi R, et al. Vaccination with autologous dendritic cells loaded with autologous tumor lysate or homogenate combined with immunomodulating radiotherapy and/or preleukapheresis IFN-α in patients with metastatic melanoma: a randomised "proof-of-principle" phase II study. J Transl Med 2014;12:209. [Crossref] [PubMed]
- Wei XX, Fong L, Small EJ. Prostate cancer immunotherapy with Sipuleucel-T: surrent standards and future directions. Expert Rev Vaccines 2015;14:1529-41. [Crossref] [PubMed]
- Akiyama Y, Tanosaki R, Inoue I, et al. Clinical response in japanese metastatic melanoma patients treated with peptide cocktail-pulse dendritic cells. J Transl Med 2005;3:4. [Crossref] [PubMed]
- Oshita C, Takikawa M, Kume A, et al. Dendritic cell-based vaccination in metastatic melanoma patients: Phase II clinical trial. Oncol Rep 2012;28:1131-8. [Crossref] [PubMed]
- Verma V, Kim Y, Lee MC, et al. Activated dendritic cells delivered in tissue compatible biomatrices induce in-situ anti-tumor CTL responses leading to tumor regression. Oncotarget 2016;7:39894-906. [Crossref] [PubMed]
- Van Lint S, Wilgenhof S, Heirman C, et al. Optimized dendritic cell-based immunotherapy for melanoma: the TriMix formula. Cancer Immunol Immunother 2014;63:959-67. [Crossref] [PubMed]
- Aris M, Barrio MM. Combining immunotherapy with oncogene-targeted therapy: a new road for melanoma treatment. Front Immunol 2015;6:46. [Crossref] [PubMed]
- Aris M, Bravo AA, Barrio MM, et al. Inoculation site from a cutaneous melanoma patient treated with an allogenic therapeutic vaccine: a case report. Front Immunol 2015;6:144. [Crossref] [PubMed]
- Bronchalo-Vicente L, Calderon-Gonzalez R, Freire J, et al. A novel therapy for melanoma developed in mice: transformation of melanoma into dendritic cells with Listeria monocytogenes. PLoS One 2015;10:e0117923. [Crossref] [PubMed]
- Calderon-Gonzalez R, Bronchalo-Vicente L, Freire J, et al. Exceptional anti-neoplastic activity of a dendritic-cell-targetted vaccine loaded with a Listeria peptide proposed against metastatic melanoma. Oncotarget 2016;7:16855-65. [Crossref] [PubMed]
- Mitchell DA, Batich KA, Gunn MD, et al. Tetanous toxoid and CCL3 improve dendritic cell vaccines in mice and gliobastoma patients. Nature 2015;519:366-9. [Crossref] [PubMed]
- Ojeda R, de Paz JL, Barrientos AG, et al. Preparation of multifunctional glyconanoparticles as a platform for potential carbohydrate-based anticancer vaccines. Carbohydr Res 2007;342:448-59. [Crossref] [PubMed]
- Brinãs RP, Sundgren A, Sahoo P, et al. Design and synthesis of multifunctional gold nanoparticles bearing tumor-associated glycopeptide antigens as potential cancer vaccines. Bioconjug Chem 2012;23:1513-23. [Crossref] [PubMed]
- Silva JM, Videira M, Gaspar R, et al. Immune system targeting by biodegradable nanoparticles for cancer vaccines. J Control Release 2013;168:179-99. [Crossref] [PubMed]
- Irvine DJ, Hanson MC, Rakhra K, et al. Synthetic Nanoparticles for Vaccines and Immunotherapy. Chem Rev 2015;115:11109-46. [Crossref] [PubMed]
- Bencherif SA, Sands RA, Ali OA, et al. Injectable cryogel-based whole cell cancer vaccines. Nat Commun 2015;6:7556. [Crossref] [PubMed]
- Ali OA, Doherty E, Mooney D, et al. Relationship of vaccine efficiency to the kinetics of DC and T cell responses induced by PLG-based cancer vaccines. Biomatter 2011;1:66-75. [Crossref] [PubMed]
- Rojo J, Diaz V, De la Fuente JM, et al. Gold glyconanoparticles as new tolos as anti-adhesive therapy. Chembiochem 2004;5:291-7. [Crossref] [PubMed]
- Grosso JF, Jure-Kunkel MN. CTLA-4 blockade in tumor models: an overview of preclinical and translational research. Cancer Immun 2013;13:5. [PubMed]
- Selby MJ, Engelhardt JJ, Johnston RJ, et al. Preclinical Development of Ipilimumab and Nivolumab Combination Immunotherapy: Mouse Tumor Models, In Vitro Functional Studies, and Cynomolgus Macaque Toxicology. PLoS One 2016;11:e0161779. [Crossref] [PubMed]
- Ali OA, Verbeke C, Johnson C, et al. Identification of immune factors regulating anti-tumour immunity using polymeric vaccines with multiple adjuvants. Cancer Res 2014;74:1670-81. [Crossref] [PubMed]
- Sanmamed MF, Carranza-Rua O, Alfaro C, et al. Serum interleukin-8 reflects tumor burden and treatment response across malignancies of multiple tissue origins. Clin Cancer Res 2014;20:5697-707. [Crossref] [PubMed]
- Márquez-Rodas I, Marin Gonzalez M, Nagore E, et al. Frequency and characteristics of familial melanoma in Spain: the FAM-GEM-1 Study. PLoS One 2015;10:e0124239. [Crossref] [PubMed]