Personalized therapy based on image for esophageal or gastroesophageal junction adenocarcinoma
Introduction
Esophageal cancer (EC) is the 8th most common of all cancers in the world (456,000 cases) and the 6th most common cause of cancer death (400,000 deaths) (1). Esophageal adenocarcinoma (EAC), one of the two common EC histologic types, has become quite prevalent in the western world (2). Despite the development of multimodality therapies, the prognosis of EAC patients remains dismal (3,4).
To date, preoperative chemotherapy or chemoradiation followed by esophagectomy is considered a standard option in cases where surgery is possible (5). After preoperative chemoradiation, ~25% patients achieve pathological complete response (pCR) (6). Patients who have pCR often experience a longer overall survival compared to those that achieve < pCR (7,8). Importantly, patients who are destined to have pCR may be able to avoid the esophagectomy (9). Therefore, predicting the response to preoperative therapy can be useful in the clinic and may allow novel algorithms.
Computed tomography (CT), positron emission tomography (PET)-CT and upper endoscopy with endoscopic ultrasound (EUS) have been the standard for staging of localized EC. In addition, these have been used for restaging after preoperative therapy (10).
Predicting preoperative chemotherapy response by PET-CT
Several studies have assessed the value of PET-CT to predict response and prognosticate after preoperative chemotherapy (Table 1). Weber et al. reported that standardized uptake value (SUV) reduction of clinical responders was significantly higher than that of non-responders (11). Subsequent studies confirmed these observations and suggested that that SUV reduction could be correlated with the degree of pathological response (12,13,15,16). MUNICON1 phase II trial prospectively evaluated whether PET is useful for predicting histopathological response and survival (14). When 35% SUVmax reduction was defined as PET-responder, major histological responses (less than 10% residual tumor) were detected in 29 of 50 PET-responders, but none in PET-non-responders. But, SUV changes could not predict pCR. The median overall survival was significantly longer for PET-responders than for PET-non-responders [hazard ratio (HR): 2.13; 95% confidence interval (CI): 1.14–3.99; P=0.015] (14). A large retrospective single institution study of 301 patients observed a relationship between SUVmax reduction and pathological response (17). As pathologic response was defined by the Mandard tumor regression grades (TRGs) 1–3 (19), SUV changes could identify pathologic response [odds ratio (OR) for each percentage reduction: 1.03; 95% CI: 1.01–1.06; P=0.003] (17). Thus, metabolic response could be correlated with pathological response. However, there is not standard cut-off value for metabolic response. PERCIST recommends 30% reduction as cut-off value, but this was applied other tumor types and not EAC (20). In EAC setting, 35% SUVmax reduction seem to be most commonly defined as metabolic responder as MUNICON trial (14).
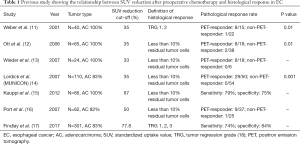
Full table
Predicting preoperative chemoradiation response by PET-CT
One drawback of this area of research is that most studies have small cohorts, they are retrospective, and conducted at single institution. Therefore, varying results have been reported (Table 2). Unlike preoperative chemotherapy, pCR can be highly anticipated after preoperative chemoradiation (9). A prospective cohort study with 138 EC patients (EAC 75%) showed that when complete metabolic response (cMR) was defined as maximal value of SUVmax of <4, only 27% patients who had cMR achieved pCR after chemoradiation (27). Elliot et al. also reported similar result. In 100 EAC patients, when cMR defined as SUVmax of <4 after preoperative chemoradiation, 46 patients (46%) achieved cMR, but 37 patients (80%) had residual EAC in the resected specimen (24). These studies confirm the limitation of SUV after chemoradiation to predict pCR.
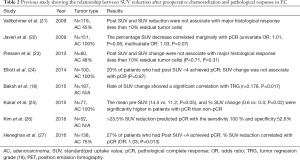
Full table
Our group reported on consecutive 151 EAC patients and noted that SUVmax changes after chemoradiation was marginally associated with pCR (univariate OR: 1.01, P=0.06; multivariate OR: 1.03, P=0.07) (22). Kukar et al. reported in 77 patients and noted that less than 45% SUV decrease was a risk factor for residual disease (25). Another study with 53 EAC patients reported that a decrease of >23.5% SUV resulted in the sensitivity and specificity for pCR prediction were 100% and 53%, respectively (26). Baksh et al. retrospectively reported SUV changes in 187 EC patients who had preoperative chemoradiation and noted a significant correlation with TRG (18). However, Piessen et al., Vallbohmer et al., and Myslivecek et al. reported the no benefit of PET-CT parameter after chemoradiation to identify histological responders (21,23,28).
Taken together, PET-CT parameter has a limitation in predicting pCR or histological response after preoperative chemoradiation. This limitation is considered due to inflammatory changes caused by chemoradiation. Inflammation and ulceration can increase SUV uptake (29,30). Further refinements are needed for better correlations.
Volumetry PET parameter
SUVmax represents maximum metabolic activity at one point in the tumor bed. Therefore alternative parameters have been proposed to assess heterogeneity by computed volumetric analyses, such as tumor volume, tumor shape, total glycolytic volume, and spatial patterns (texture features) (31-33). TLG is calculated by the product of average of SUV and metabolic tumor volume (MTV) (34). Therefore, TLG can represent more accurate whole tumor metabolic activity than SUVmax. We reported a prospective phase II trial to assess the SUVmax or TLG could predict pCR, but noted that TLG was prognostic but none of the PET variables was predictive of pCR (35). However, this study was very small. A larger but retrospective study from our institution assessed whether baseline and post-chemoradiation PET changes including texture analysis can improve prediction of pCR in 217 EAC patients (36). Especially TLG improved prediction of pCR, but was not sufficient for clinical implementation (36). Hatt et al. reported in 50 patients who underwent chemoradiation (EAC: 28%) and noted that initial SUV parameter was similar between clinical responders and no-responders, while TLG parameter was significantly less in patients with clinical CR (37). And pretreatment TLG of <58 predicted clinical CR with 75% sensitivity and 92% specificity, which was more valuable than SUV (37). Furthermore, Roedl et al. reported that a decrease of TLG by >78% had better predictive values (91% sensitivity and 93% specificity) than SUV did (38). However, another study with 79 patients who underwent preoperative chemoradiation could not demonstrate the correlation between TLG after treatment and pCR (39). A study of 50 EC patients with preoperative chemotherapy reported that a certain TLG change (>40% reduction) was an independent prognosticator in multivariable analysis (multivariate HR: 3.89; 95% CI: 1.46–10.3; P=0.006), but SUV change was not independent prognosticator (40). Further studies are expected.
PET guided treatment
If PET-parameters could identify exquisitely treatment sensitive tumors, we could avoid surgery in some patients. Conversely for patients with resistant tumor, we could avoid toxic and ineffective therapies. Therefore, PET-guided treatment algorithm in EAC has been evaluated. The Municon I trial is the first phase II trial which assessed the PET-response guided treatment algorithm for EAC patients (14). PET responders (>35% SUV reduction) after one cycle chemotherapy continued the same chemotherapy then had surgery, but non-responder stopped chemotherapy and had surgery. The prognosis of responders was better than that for non-responders. In Municon II trial, non-responder received additional chemoradiation, but still the non-responders had poor prognosis compared to responders (41). This suggests that empirically changing therapy cannot overcome primary resistance and in depth analyses are needed to derive benefits.
A Phase II trial evaluating induction chemotherapy followed by concurrent chemoradiation in EC showed that PET responder for induction chemotherapy (>35% SUV reduction) had higher frequency of pCR and favorable prognosis (42). Ongoing CALGB 80803 study has evaluated the strategy of changing concurrent chemotherapy with chemoradiation based on PET response to induction chemotherapy (43). A total of 257 EAC patients assigned to the FOLFOX or Carbo/Taxol group, and then PET non-responder were crossed over to alternative regimen. pCR rate of PET responder was 26% and that of PET non-responder was 18%, but this is not significant. This study design is not ideal to figure out if pCR can be improved by changing concurrent chemotherapy. In the ideal design, one would continue would have two non responding cohorts: one cohort will receive the same chemotherapy with radiation and the other cohort will receive the alternate chemotherapy.
Ancillary analysis of the CROSS trial reported whether the early assessment could predict response for preoperative chemoradiation (44). PET-CT was performed before treatment and 14 days after the start of treatment. The median SUV reduction of histopathologic responders (less than 10% residual tumor) was significantly higher than that of non-responders; 30.9% for histopathologic responders and 1.7% for non-responders (P=0.001). This confirms previous reports. However, when 0% SUV reduction was used as cutoff value, PET identified histopathologic response with 91% sensitivity and 50% specificity. This low specificity indicates difficulty of predicting non-responder by early PET evaluation (44).
Magnetic resonance imaging (MRI)
Diffusion-weighted MRI (DW-MRI) has been evaluated for prediction of treatment response in various cancers (45-47). The apparent diffusion coefficient (ADC) is calculated by diffusion or microstructural density (48). Because diffusion within tumor is interrupted by cellular membranes or macromolecular structures, presence or residual tumor cell can be detected as ADC decrease, conversely treatment response can be detected as ADC increase (49). So far, three studies evaluate the benefit of DW-MRI for predicting preoperative treatment (50-52). A prospective study found that change in ADC during preoperative chemoradiation was associated with pCR (50). One study reported similar result that change in ADC was associated with TRG (52), but another study did not (51). These studies were small, therefore a multi-center study assessing the value of MRI and PET-CT for predicting of preoperative treatment response (NCT02125448).
Discussion
Approximately 25% patients achieve a pCR after preoperative chemoradiation (6). If pCR could be predicted before surgery, one could consider an esophageal preservation strategy. Our group reported that clinical CR defined as negative endoscopic biopsies and PET with physiologic uptake led to favorable OS, but did not predict pCR (53,54). Therefore, we recommend that all operable EAC patients proceed to surgery. To date, the proposed Surgery-as-Needed Approach in Esophageal Cancer (SANO) trial is ongoing, evaluating whether clinical CR at 2-point evaluation (PET and EUS) after preoperative chemoradiation could predict pCR (55). New PET parameter including texture analysis or MRI might provide better pCR prediction.
Identifying who might achieve pCR after chemoradiation is important, but PET parameters are currently unable. Early PET changes can potentially play a role (14,41).
Recently, whole genome analyses of EAC are shedding light on subtypes that exist (56-60). Several gene expression analyses are exploring predictive biomarkers including glucose transporters-1 and hexokinase (61-64).
Conclusions
This review describes the current state of understanding and future possibilities of images for predicting preoperative therapy response and for guiding personalized therapy in EAC patients. New PET parameters such as TLG or MRI may provide better response prediction. Furthermore, PET parameters combined with genomic profiling might enhance better treatment selection, prediction, and prognostication.
Acknowledgements
Funding: This work was supported by generous grants from the Caporella, Dallas, Sultan, Park, Smith, Frazier, Oaks, Vanstekelenberg, Planjery, and Cantu Families. From the Schecter Private Foundation, Rivercreek Foundation, Kevin Fund, Myer Fund, Dio Fund, Milrod Fund, and Multidisciplinary Grants from the University of Texas M. D. Anderson Cancer Center, Houston, USA. Supported in part by the National Cancer Institute and Department of Defense awards CA138671, CA172741, CA129926, CA150334 (JA Ajani). It was also supported by a grant from Japan Society for the Promotion of Science Overseas Research Fellowships and Program for Advancing Strategic International Networks to Accelerate the Circulation of Talented Researchers from Japan Society for the Promotion of Science (K Harada).
Footnote
Conflicts of Interest: The authors have no conflicts of interest to declare.
References
- Ferlay J, Soerjomataram I, Dikshit R, et al. Cancer incidence and mortality worldwide: sources, methods and major patterns in GLOBOCAN 2012. Int J Cancer 2015;136:E359-86. [Crossref] [PubMed]
- Lagergren J, Bergstrom R, Lindgren A, et al. Symptomatic gastroesophageal reflux as a risk factor for esophageal adenocarcinoma. N Engl J Med 1999;340:825-31. [Crossref] [PubMed]
- Allum WH, Stenning SP, Bancewicz J, et al. Long-term results of a randomized trial of surgery with or without preoperative chemotherapy in esophageal cancer. J Clin Oncol 2009;27:5062-7. [Crossref] [PubMed]
- Gertler R, Stein HJ, Langer R, et al. Long-term outcome of 2920 patients with cancers of the esophagus and esophagogastric junction: evaluation of the New Union Internationale Contre le Cancer/American Joint Cancer Committee staging system. Ann Surg 2011;253:689-98. [Crossref] [PubMed]
- Mariette C, Balon JM, Piessen G, et al. Pattern of recurrence following complete resection of esophageal carcinoma and factors predictive of recurrent disease. Cancer 2003;97:1616-23. [Crossref] [PubMed]
- van Hagen P, Hulshof MC, van Lanschot JJ, et al. Preoperative chemoradiotherapy for esophageal or junctional cancer. N Engl J Med 2012;366:2074-84. [Crossref] [PubMed]
- Donahue JM, Nichols FC, Li Z, et al. Complete pathologic response after neoadjuvant chemoradiotherapy for esophageal cancer is associated with enhanced survival. Ann Thorac Surg 2009;87:392-8; discussion 8-9. [Crossref] [PubMed]
- Berger AC, Farma J, Scott WJ, et al. Complete response to neoadjuvant chemoradiotherapy in esophageal carcinoma is associated with significantly improved survival. J Clin Oncol 2005;23:4330-7. [Crossref] [PubMed]
- Swisher SG, Moughan J, Komaki RU, et al. Final Results of NRG Oncology RTOG 0246: An Organ-Preserving Selective Resection Strategy in Esophageal Cancer Patients Treated with Definitive Chemoradiation. J Thorac Oncol 2017;12:368-74. [Crossref] [PubMed]
- Findlay JM, Gillies RS, Franklin JM, et al. Restaging oesophageal cancer after neoadjuvant therapy with (18)F-FDG PET-CT: identifying interval metastases and predicting incurable disease at surgery. Eur Radiol 2016;26:3519-33. [Crossref] [PubMed]
- Weber WA, Ott K, Becker K, et al. Prediction of response to preoperative chemotherapy in adenocarcinomas of the esophagogastric junction by metabolic imaging. J Clin Oncol 2001;19:3058-65. [Crossref] [PubMed]
- Ott K, Weber WA, Lordick F, et al. Metabolic imaging predicts response, survival, and recurrence in adenocarcinomas of the esophagogastric junction. J Clin Oncol 2006;24:4692-8. [Crossref] [PubMed]
- Wieder HA, Ott K, Lordick F, et al. Prediction of tumor response by FDG-PET: comparison of the accuracy of single and sequential studies in patients with adenocarcinomas of the esophagogastric junction. Eur J Nucl Med Mol Imaging 2007;34:1925-32. [Crossref] [PubMed]
- Lordick F, Ott K, Krause BJ, et al. PET to assess early metabolic response and to guide treatment of adenocarcinoma of the oesophagogastric junction: the MUNICON phase II trial. Lancet Oncol 2007;8:797-805. [Crossref] [PubMed]
- Kauppi JT, Oksala N, Salo JA, et al. Locally advanced esophageal adenocarcinoma: response to neoadjuvant chemotherapy and survival predicted by ([18F])FDG-PET/CT. Acta Oncol 2012;51:636-44. [Crossref] [PubMed]
- Port JL, Lee PC, Korst RJ, et al. Positron emission tomographic scanning predicts survival after induction chemotherapy for esophageal carcinoma. Ann Thorac Surg 2007;84:393-400; discussion 400. [Crossref] [PubMed]
- Findlay JM, Bradley KM, Wang LM, et al. Predicting Pathologic Response of Esophageal Cancer to Neoadjuvant Chemotherapy: The Implications of Metabolic Nodal Response for Personalized Therapy. J Nucl Med 2017;58:266-75. [Crossref] [PubMed]
- Baksh K, Prithviraj G, Kim Y, et al. Correlation Between Standardized Uptake Value in Preneoadjuvant and Postneoadjuvant Chemoradiotherapy and Tumor Regression Grade in Patients With Locally Advanced Esophageal Cancer. Am J Clin Oncol 2015. [Epub ahead of print]. [Crossref] [PubMed]
- Mandard AM, Dalibard F, Mandard JC, et al. Pathologic assessment of tumor regression after preoperative chemoradiotherapy of esophageal carcinoma. Clinicopathologic correlations. Cancer 1994;73:2680-6. [Crossref] [PubMed]
- Wahl RL, Jacene H, Kasamon Y, et al. From RECIST to PERCIST: Evolving Considerations for PET response criteria in solid tumors. J Nucl Med 2009;50 Suppl 1:122S-50S. [Crossref] [PubMed]
- Vallbohmer D, Holscher AH, Dietlein M, et al. [18F]-Fluorodeoxyglucose-positron emission tomography for the assessment of histopathologic response and prognosis after completion of neoadjuvant chemoradiation in esophageal cancer. Ann Surg 2009;250:888-94. [Crossref] [PubMed]
- Javeri H, Xiao L, Rohren E, et al. The higher the decrease in the standardized uptake value of positron emission tomography after chemoradiation, the better the survival of patients with gastroesophageal adenocarcinoma. Cancer 2009;115:5184-92. [Crossref] [PubMed]
- Piessen G, Petyt G, Duhamel A, et al. Ineffectiveness of (1)(8)F-fluorodeoxyglucose positron emission tomography in the evaluation of tumor response after completion of neoadjuvant chemoradiation in esophageal cancer. Ann Surg 2013;258:66-76. [Crossref] [PubMed]
- Elliott JA, O'Farrell NJ, King S, et al. Value of CT-PET after neoadjuvant chemoradiation in the prediction of histological tumour regression, nodal status and survival in oesophageal adenocarcinoma. Br J Surg 2014;101:1702-11. [Crossref] [PubMed]
- Kukar M, Alnaji RM, Jabi F, et al. Role of Repeat 18F-Fluorodeoxyglucose Positron Emission Tomography Examination in Predicting Pathologic Response Following Neoadjuvant Chemoradiotherapy for Esophageal Adenocarcinoma. JAMA Surg 2015;150:555-62. [Crossref] [PubMed]
- Kim SJ, Koo PJ, Chang S. Predictive value of repeated F-18 FDG PET/CT parameters changes during preoperative chemoradiotherapy to predict pathologic response and overall survival in locally advanced esophageal adenocarcinoma patients. Cancer Chemother Pharmacol 2016;77:723-31. [Crossref] [PubMed]
- Heneghan HM, Donohoe C, Elliot J, et al. Can CT-PET and Endoscopic Assessment Post-Neoadjuvant Chemoradiotherapy Predict Residual Disease in Esophageal Cancer? Ann Surg 2016;264:831-8. [Crossref] [PubMed]
- Myslivecek M, Neoral C, Vrba R, et al. The value of (1)(8)F-FDG PET/CT in assessment of metabolic response in esophageal cancer for prediction of histopathological response and survival after preoperative chemoradiotherapy. Biomed Pap Med Fac Univ Palacky Olomouc Czech Repub 2012;156:171-9. [Crossref] [PubMed]
- Erasmus JJ, Munden RF, Truong MT, et al. Preoperative chemo-radiation-induced ulceration in patients with esophageal cancer: a confounding factor in tumor response assessment in integrated computed tomographic-positron emission tomographic imaging. J Thorac Oncol 2006;1:478-86. [Crossref] [PubMed]
- Hautzel H, Muller-Gartner HW. Early changes in fluorine-18-FDG uptake during radiotherapy. J Nucl Med 1997;38:1384-6. [PubMed]
- Prasad SR, Jhaveri KS, Saini S, et al. CT tumor measurement for therapeutic response assessment: comparison of unidimensional, bidimensional, and volumetric techniques initial observations. Radiology 2002;225:416-9. [Crossref] [PubMed]
- O'Sullivan F, Roy S, O'Sullivan J, et al. Incorporation of tumor shape into an assessment of spatial heterogeneity for human sarcomas imaged with FDG-PET. Biostatistics 2005;6:293-301. [Crossref] [PubMed]
- Tixier F, Le Rest CC, Hatt M, et al. Intratumor heterogeneity characterized by textural features on baseline 18F-FDG PET images predicts response to concomitant radiochemotherapy in esophageal cancer. J Nucl Med 2011;52:369-78. [Crossref] [PubMed]
- Larson SM, Erdi Y, Akhurst T, et al. Tumor Treatment Response Based on Visual and Quantitative Changes in Global Tumor Glycolysis Using PET-FDG Imaging. The Visual Response Score and the Change in Total Lesion Glycolysis. Clin Positron Imaging 1999;2:159-71. [Crossref] [PubMed]
- Elimova E, Wang X, Etchebehere E, et al. 18-fluorodeoxy-glucose positron emission computed tomography as predictive of response after chemoradiation in oesophageal cancer patients. Eur J Cancer 2015;51:2545-52. [Crossref] [PubMed]
- van Rossum PS, Fried DV, Zhang L, et al. The Incremental Value of Subjective and Quantitative Assessment of 18F-FDG PET for the Prediction of Pathologic Complete Response to Preoperative Chemoradiotherapy in Esophageal Cancer. J Nucl Med 2016;57:691-700. [Crossref] [PubMed]
- Hatt M, Visvikis D, Pradier O, et al. Baseline 18F-FDG PET image-derived parameters for therapy response prediction in oesophageal cancer. Eur J Nucl Med Mol Imaging 2011;38:1595-606. [Crossref] [PubMed]
- Roedl JB, Colen RR, Holalkere NS, et al. Adenocarcinomas of the esophagus: response to chemoradiotherapy is associated with decrease of metabolic tumor volume as measured on PET-CT. Comparison to histopathologic and clinical response evaluation. Radiother Oncol 2008;89:278-86. [Crossref] [PubMed]
- Blom RL, Steenbakkers IR, Lammering G, et al. PET/CT-based metabolic tumour volume for response prediction of neoadjuvant chemoradiotherapy in oesophageal carcinoma. Eur J Nucl Med Mol Imaging 2013;40:1500-6. [Crossref] [PubMed]
- Tamandl D, Gore RM, Fueger B, et al. Change in volume parameters induced by neoadjuvant chemotherapy provide accurate prediction of overall survival after resection in patients with oesophageal cancer. Eur Radiol 2016;26:311-21. [Crossref] [PubMed]
- zum Buschenfelde CM, Herrmann K, Schuster T, et al. 18F-FDG PET-guided salvage neoadjuvant radiochemotherapy of adenocarcinoma of the esophagogastric junction: the MUNICON II trial. J Nucl Med 2011;52:1189-96. [Crossref] [PubMed]
- Ilson DH, Minsky BD, Ku GY, et al. Phase 2 trial of induction and concurrent chemoradiotherapy with weekly irinotecan and cisplatin followed by surgery for esophageal cancer. Cancer 2012;118:2820-7. [Crossref] [PubMed]
- Goodman KA, Niedzwiecki D, Hall N, et al. Initial results of CALGB 80803 (Alliance): A randomized phase II trial of PET scan-directed combined modality therapy for esophageal cancer. J Clin Oncol 2017;35:1. [Crossref] [PubMed]
- van Heijl M, Omloo JM, van Berge Henegouwen MI, et al. Fluorodeoxyglucose positron emission tomography for evaluating early response during neoadjuvant chemoradiotherapy in patients with potentially curable esophageal cancer. Ann Surg 2011;253:56-63. [Crossref] [PubMed]
- Mardor Y, Pfeffer R, Spiegelmann R, et al. Early detection of response to radiation therapy in patients with brain malignancies using conventional and high b-value diffusion-weighted magnetic resonance imaging. J Clin Oncol 2003;21:1094-100. [Crossref] [PubMed]
- Joye I, Deroose CM, Vandecaveye V, et al. The role of diffusion-weighted MRI and (18)F-FDG PET/CT in the prediction of pathologic complete response after radiochemotherapy for rectal cancer: a systematic review. Radiother Oncol 2014;113:158-65. [Crossref] [PubMed]
- Powell C, Schmidt M, Borri M, et al. Changes in functional imaging parameters following induction chemotherapy have important implications for individualised patient-based treatment regimens for advanced head and neck cancer. Radiother Oncol 2013;106:112-7. [Crossref] [PubMed]
- Koh DM, Collins DJ. Diffusion-weighted MRI in the body: applications and challenges in oncology. AJR Am J Roentgenol 2007;188:1622-35. [Crossref] [PubMed]
- Thoeny HC, Ross BD. Predicting and monitoring cancer treatment response with diffusion-weighted MRI. J Magn Reson Imaging 2010;32:2-16. [Crossref] [PubMed]
- van Rossum PS, van Lier AL, van Vulpen M, et al. Diffusion-weighted magnetic resonance imaging for the prediction of pathologic response to neoadjuvant chemoradiotherapy in esophageal cancer. Radiother Oncol 2015;115:163-70. [Crossref] [PubMed]
- Kwee RM, Dik AK, Sosef MN, et al. Interobserver reproducibility of diffusion-weighted MRI in monitoring tumor response to neoadjuvant therapy in esophageal cancer. PLoS One 2014;9:e92211. [Crossref] [PubMed]
- De Cobelli F, Giganti F, Orsenigo E, et al. Apparent diffusion coefficient modifications in assessing gastro-oesophageal cancer response to neoadjuvant treatment: comparison with tumour regression grade at histology. Eur Radiol 2013;23:2165-74. [Crossref] [PubMed]
- Suzuki A, Xiao L, Taketa T, et al. Results of the baseline positron emission tomography can customize therapy of localized esophageal adenocarcinoma patients who achieve a clinical complete response after chemoradiation. Ann Oncol 2013;24:2854-9. [Crossref] [PubMed]
- Taketa T, Correa AM, Suzuki A, et al. Outcome of trimodality-eligible esophagogastric cancer patients who declined surgery after preoperative chemoradiation. Oncology 2012;83:300-4. [Crossref] [PubMed]
- Noordman BJ, Shapiro J, Spaander MC, et al. Accuracy of Detecting Residual Disease After Cross Neoadjuvant Chemoradiotherapy for Esophageal Cancer (preSANO Trial): Rationale and Protocol. JMIR Res Protoc 2015;4:e79. [Crossref] [PubMed]
- Nones K, Waddell N, Wayte N, et al. Genomic catastrophes frequently arise in esophageal adenocarcinoma and drive tumorigenesis. Nat Commun 2014;5:5224. [Crossref] [PubMed]
- Dulak AM, Stojanov P, Peng S, et al. Exome and whole-genome sequencing of esophageal adenocarcinoma identifies recurrent driver events and mutational complexity. Nat Genet 2013;45:478-86. [Crossref] [PubMed]
- Wang K, Johnson A, Ali SM, et al. Comprehensive Genomic Profiling of Advanced Esophageal Squamous Cell Carcinomas and Esophageal Adenocarcinomas Reveals Similarities and Differences. Oncologist 2015;20:1132-9. [Crossref] [PubMed]
- Secrier M, Li X, de Silva N, et al. Mutational signatures in esophageal adenocarcinoma define etiologically distinct subgroups with therapeutic relevance. Nat Genet 2016;48:1131-41. [Crossref] [PubMed]
- Cancer Genome Atlas Research Network. Analysis Working Group: Asan University; BC Cancer Agency; et al. Integrated genomic characterization of oesophageal carcinoma. Nature 2017;541:169-75. [Crossref] [PubMed]
- Sawayama H, Ishimoto T, Watanabe M, et al. High expression of glucose transporter 1 on primary lesions of esophageal squamous cell carcinoma is associated with hematogenous recurrence. Ann Surg Oncol 2014;21:1756-62. [Crossref] [PubMed]
- Younes M, Lechago LV, Somoano JR, et al. Wide expression of the human erythrocyte glucose transporter Glut1 in human cancers. Cancer Res 1996;56:1164-7. [PubMed]
- Izuishi K, Yamamoto Y, Sano T, et al. Molecular mechanism underlying the detection of colorectal cancer by 18F-2-fluoro-2-deoxy-D-glucose positron emission tomography. J Gastrointest Surg 2012;16:394-400. [Crossref] [PubMed]
- Fareed KR, Kaye P, Soomro IN, et al. Biomarkers of response to therapy in oesophago-gastric cancer. Gut 2009;58:127-43. [Crossref] [PubMed]