The physiological roles of secretin and its receptor
General background
The gastrointestinal peptide hormone, secretin, was originally identified by Bayliss and Starling in 1902 (1). Secretin is a member of the secretin-glucagon family and is secreted by S cells of the duodenum in the crypts of Lieberkühn (2). Secretin affects the function of a number of organ systems and cell types (1-7). Secretin exerts its biological effects through G-protein coupled secretin receptors (SR), which are expressed in the basolateral domain of several cells (3,5,8-11). In addition to regulating the pH of the duodenal content by the control of gastric acid secretion (12), secretin regulates the secretion of bicarbonate ions into the duodenum from the epithelia lining the pancreatic and biliary ducts (3,6,7,13). In addition to regulating water homeostasis (4), secretin has been considered as a neuropeptide hormone since it is also expressed in the central nervous system (CNS) (14-16). Recent evidence has indicated that secretin has pleiotropic effects in several organ systems (including the biliary epithelium) (17) and has been termed a neuroendocrine hormone (18).
The neuroendocrine hormone secretin
Structure of secretin
The peptide sequence of secretin was first determined with porcine secretin in 1970 (19). The human secretin gene is 514 bp, which is longer than that of mouse or rat, 507 bp and 405 bp, respectively (20,21), NCBI Reference Sequence: NM_022670.2. The human secretin gene shows 42.7% homology to the mouse secretin gene, and 46.8% homology to the rat secretin gene. Homology was analyzed using SCAN2 software from Softberry, http://linux1.softberry.com/berry.phtml?topic=scan2&group=programs&subgroup=scanh subsequently, % homology was calculated independently. Secretin is a 27-amino acid peptide and is the active form of pro-secretin, which is known as a preprohormone and is synthesized as a larger precursor like other regulatory peptides (22,23). Secretin is initially synthesized as a 120 amino acid precursor protein. This precursor contains an N-terminal signal peptide, spacer, secretin itself (residues 28-54), and a 72-amino acid C-terminal peptide (24). This peptide is proteolytically processed to yield a single linear 27-amino acid peptide hormone with a molecular weight of 3,055 kD by removal of the signal peptide, plus amino and carboxy-terminal extensions (25). The sequence of the mature peptide has homology to that of other peptides isolated from the gastrointestinal tract including glucagon, vasoactive intestinal peptide (VIP) and gastric inhibitory peptide (PHI-27), which are the members of the secretin family (25,26). The gene and amino acid sequence of the human secretin is depicted in Figure 1.
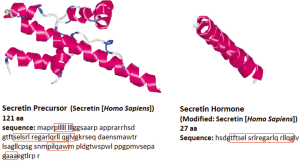
Anatomical sites of secretin synthesis and secretion
The predominant anatomical site of secretin synthesis is in the cytoplasmic secretory granules of S-cells that are located in the mucosa of the small intestine in the crypts of Lieberkühn (2). Secretin is also secreted by specific endocrine cells in the mucosa of the proximal small intestine and expressed in smaller numbers in the jejunum of duodenum (2,29). In addition to being localized in the duodenum (2,24), secretin is also expressed in the CNS (30,31). Indeed, a study has demonstrated that secretin is expressed in both the pituitary and pineal glands and at lower levels in the hypothalamus, thalamus, and olfactory lobe (32-34). The mRNA encoding secretin is also expressed in the Purkinje cells of the rat cerebellar cortex (35). The fact that secretin precursor mRNA in the brain has the same coding sequence as that of the duodenum suggests the secretin precursor protein in the small intestine is perhaps the same as the one synthesized in the brain. Conversely, another study has shown low secretin immunoreactivity in the brain (24). Further studies are warranted to clarify this discrepancy. The secretin precursor gene is also expressed in the heart, lung, kidney, testis, and brain as well as the gastrointestinal tract including the biliary epithelium (36,37). The mRNA for secretin and its receptor have been also demonstrated in the intestine, heart, and pancreas (24,38,39). Preliminary data from our laboratory have also shown that: (I) cholangiocytes express the mRNA for secretin and synthesize secretin; and (II) knockout of the gene for secretin reduces biliary growth in cholestatic mice likely by an autocrine mechanism (37).
Brief general background on secretin biological effects
Secretin exerts pharmacological effects in a number of organs including the heart, kidney, lung, and brain (40-43). Secretin has been show to stimulate bile and bicarbonate secretion in the duodenum (44), pancreatic (45) and biliary (3,7,46) ducts as well gastric pepsin secretion (47). Also, secretin inhibits gastric acid secretin and food-stimulated gastrin release (48) as well as upper small intestinal motility and lower esophageal sphincter pressure (49). Secretin induces the release of insulin from the pancreas following ingestion of glucose and may be important for the management of blood sugar levels (50).
The G-protein coupled secretin receptor
Expression of secretin in various tissues and cell types
Human secretin receptor was originally isolated from lung cells (51) and found on the basolateral domain of the epithelia within the tertiary bronchus (52). The expression of the secretin receptor has been demonstrated in a number of organs including the brain (cerebellum, hippocampus and central amygdala) of humans and rodents (33,53,54), pancreas (14), stomach (14,55), kidney (14,41) as well the biliary epithelium in the liver (3,8,56-58). Immunoreactivity for secretin receptors is present in the renal medulla, proximal tubules, and ascending thick segment of the loop of Henle in the kidney (53).
A number of studies have also shown that secretin receptors are only expressed in cholangiocytes in the liver (3,8,38,57,59,60). An in vivo autoradiography study in rat liver has shown specific binding of 125I-labeled secretin to bile duct areas of normal and BDL rats, binding that increased following BDL (59). In vitro studies have shown that secretin stimulates exocytosis in normal cholangiocytes by increasing intracellular adenosine 3',5'-cyclic monophosphate (cAMP) levels by interaction with secretin receptors (61). Conclusive evidence came from our recent studies showing that: (I) large (but not small) cholangiocytes in rodent liver express secretin receptors (3,57); and (II) the expression of this receptor increases in models of intra- and extra-hepatic cholestasis such α-naphthylisothiocyanate feeding (62) and BDL (8,56) and decrease in models of biliary damage loss (e.g., after CCl4 administration) (63,64). Small cholangiocytes (which normally do not express secretin receptor) (63,64) acquire de novo this receptor only during damage of large cAMP-responsive cholangiocytes (63-65). The secretin receptor is also expressed and up-regulated in kidney and cholangiocytes in rodent models of polycystic kidney and liver disease such as in Pkd2(-/WS25) mice (66). A study (analyzing the expression of secretin receptor in human samples) has demonstrated the presence of these receptors in normal bile ducts and ductules but not in hepatocytes (67). The expression of this receptor was higher in ductules during liver cirrhosis and cholangiocarcinoma, whereas no immunohistochemical reaction was observed in hepatocellular carcinomas (67). Conclusive evidence for the presence of secretin receptor in cholangiocytes came from a recent study showing that knockout of secretin receptor reduces large cholangiocyte hyperplasia in cholestatic BDL mice (17). Thus, changes in the expression of this receptor may be a unique tool for managing the balance between biliary growth/damage in chronic cholestatic liver diseases (17,62-65).
Signaling mechanisms
The effects of secretin on the gastrointestinal tract and other organ systems are mediated by interaction with basolateral SR (15). The secretin receptor has seven membrane-spanning domains and it is a typical G protein-coupled receptor (GPCR) under the class B GPCR subfamily (5). The messenger system, cAMP, is classical signaling that is activated by secretin in a number of systems such as the pancreas, brain, kidney as well as the biliary epithelium. For example, in bile ducts the activation of cAMP signaling by secretin induces phosphorylation of protein kinase A (PKA) that causes activation of cystic fibrosis transmembrane conductance regulator (CFTR), which in turn induces activation of the Cl-/HCO3- anion exchanger 2 (AE2) (3,7,57,68). Also, impaired pancreatic ductal bicarbonate secretion has been observed in cystic fibrosis (6,69). The cAMP signaling system plays a key role in the modulation of large biliary secretion and proliferation since it is activated by secretin (stimulating bicarbonate secretion) (3,57,68) and also stimulates large cholangiocyte proliferation (17,56). Down- or up-regulation of cAMP signaling (for example by somatostatin, gastrin, endothelin-1, the α2-adrenergic receptor agonist, UK14, 304, or the α1-adrenergic agonist, phenylephrine) (70-74) has also been associated with decreases/increases of secretin-stimulated ductal secretion. While some of the inhibitory effects on secretin-induced choleresis are mediated by direct downregulation of cAMP signaling, others depend on the activation of Ca2+-dependent PKC isoforms that subsequently induce (by Ca2+→cAMP cross-talk) changes in cAMP levels and secretin-stimulated ductal secretion (70-74). The cAMP second messenger system (that is not constitutively active in small cholangiocytes) (63,64) is de novo activated in these cells during the damage of large, cAMP-responsive bile ducts.
In the pancreas, secretin receptors are key for the maintenance of healthy ductal epithelial cells, but they are functionally altered in ductal pancreatic adenocarcinomas. Recently, silencing of secretin receptor function by dimerization with a misspliced variant secretin receptor has been shown in ductal pancreatic adenocarcinoma (75). Although wild-type secretin receptor mRNAs were detected in the primary tumors in these studies, the lack of biological response to secretin is likely due to the co-expression of a second and predominant transcript in these tumor lines (75). This represented a variant of the secretin receptor in which the third exon is spliced out to eliminate residues 44-79 from the NH[2]-terminal tail (75). The study suggested that suppression of SR activity in pancreatic carcinoma might facilitate tumor growth and progression of this neoplasm. Wild-type and splice-variant secretin receptors have been shown in tumor and non-tumor lung pathology as well (76). Dominant negative action of an abnormal SR arising from mRNA missplicing has been detected in gastrinoma (77). Reduced secretin binding has also been shown in pancreatic ductal tumors and likely relate to (alternatively spliced) secretin receptor isoforms (78). Targeting secretin receptors may be important in the management of pancreatic tumors.
Regulation of gastric acid secretion by secretin
A number of studies and review articles exist regarding the role of secretin in the regulation of acid secretion from oxyntic cells (12,79-82). For example, secretin inhibits gastric acid secretion and motility via a vagal afferent pathway in rats (79). These results indicate that inhibition of pentagastrin-stimulated acid secretion by secretin is mediated by a capsaicin-sensitive vagal afferent pathway (79). Other studies in rats have demonstrated that the inhibitory action of secretin on pentagastrin-stimulated gastric acid secretion is regulated by both somatostatin and prostaglandins (80,81). This topic is beyond the purpose of this review and we refer to other studies for further detial (12,79-82).
Regulation of pancreatic bicarbonate secretion
Secretin stimulates the secretion of a bicarbonate-rich pancreatic fluid (45). Secretin enters the blood stream or intestinal lumen and stimulates bicarbonate secretion (by interacting with pancreatic ductal cells), which neutralizes the pH of the gastric chyme upon entering the small intestine (83,84). cAMP signaling plays a key role for the secretion of bicarbonate ions from the pancreatic ducts. Secretin-induced bicarbonate secretion depends on the activation of the cAMP-dependent anion channel, CFTR, which is localized in the apical membrane of various epithelia including pancreas and bile ducts (6). In fact, pancreatic ducts from CFTR-null mice secrete water and bicarbonate at lower levels compared to wild-type animals (85).
Role of secretin in water homeostasis
Body water homeostasis is a critical phenomenon for the survival of cells since it maintains the balance between water intake and excretion in the body. Kidney are essential parts of the urinary system and serve homeostatic functions by regulating acid-base balance as well as blood-pressure via maintaining salt and water balance (86). Several hormones have direct influence on the regulation of renal handling of water and electrolytes by anti-diuretic or diuretic effects. As an anti-diuretic hormone, vasopressin regulates the osmolarity of extracellular fluids by modulating the amount of free water excreted by the kidney. Vasopressin has an effect on the collecting ducts, where it induces the insertion of aquaporin 2 (AQP2) water channels on the apical membranes of these cells (87,88). Regarding secretin, a study suggested that this gastrointestinal hormone has diuretic effects in a number of species including humans and dogs (89). Secretin has been shown to induce an increase in urinary volume and bicarbonate excretion in normal human subjects (89,90). A study by Waldum et al. has shown that secretin induced an increase in renal plasma flow that may be due to enhanced renal vasodilation or cardiac output, or a combination of both that impairs tubular sodium reabsorption (91). Another study has demonstrated the diuretic effect of secretin in dogs, which resulted in diuresis and significant increases in sodium and potassium output (92).
Role of secretin in the central nervous system
A number of studies suggest that secretin is expressed in the brain and regulates the function of the central nervous system (CNS) (24,35,36). For example, impaired hippocampal synaptic function has been demonstrated in secretin-deficient mice (16). Impaired synaptic plasticity and social behavior has been shown in secretin receptor-deficient mice (14). The studies demonstrate that the secretin/secretin receptor axis may be important in the regulation of the function of the CNS (14,16). Other studies have shown that the secretin gene is expressed in serotoninergic mesencephalic neurons during development (93). It was shown that secretin has trophic effects on these neurons, effects that are lost in neurodegenerative disorders (93). Additional evidence suggests that secretin can act as a neuropeptide within the CNS (94).
Role of secretin in the heart and cardiovascular system
Sympathetic and parasympathetic nerve fibers have been shown to regulate cardiovascular function through an autonomic system (95). Indeed, several neuropeptides are secreted in the heart (95-97) and may play an important role in the autonomic regulation of cardiac function as neurotransmitters or neuromodulators (95). In this review, we discuss the role of secretin in the regulation of heart function, which acts not only a hormone, but also as a neurotransmitter or neuromodulators (98). Generally speaking, secretin effects are mediated by activation of cAMP synthesis that is decreased during cardiac pathologies. For example, reduced responsiveness of cardiac secretin-stimulated adenylate cyclase was observed in the spontaneous hypertensive rat heart model (99,100). Similarly, in the hypertrophic rat heart model there was reduced secretin stimulated adenylate cyclase that was likely due to a decrease in the number of secretin receptors in cardiac myocytes (101). In cats and dogs, secretin has been shown to increase cardiac output and heart rate, whereas it decreases systemic arteriolar resistance and left ventricular end-diastolic pressure, with no significant change in stroke volume (102,103).
Role of secretin in cholangiocytes
Secretin-induced choleresis
A number of studies have shown that secretin stimulates the secretion of water and electrolytes in cholangiocytes by activating cAMP synthesis that induces phosphorylation of PKA, opening of CFTR and activation of the apically located Cl-/HCO3- exchanger AE2 (3,7,56,57,61,68,70,72,104) (Figure 2). Consistent with these data regarding the role of cAMP in the modulation of secretin-induced choleresis, a study has shown that chronic administration of cAMP agonists (i.e., forskolin) enhances secretin-stimulated bile and bile secretion (105). Other studies have shown that maintenance of the bile acid pool is important for bile secretion, an effect that is mediated by the function of the chloride-bicarbonate exchange AE2 (106). Further mechanistic studies have also shown that secretin-stimulated bile secretion is mediated by the microtubule-dependent insertion of aquaporin-1 water channels (AQP1) into the apical membrane of rodent cholangiocytes.
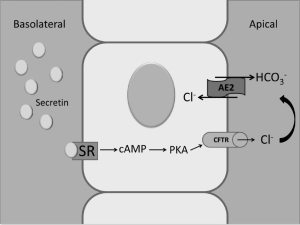
Recent studies have demonstrated that large but not small cholangiocytes (lining small and large bile ducts, respectively) (3,57,107) are the anatomical sites of secretin-stimulated water and bicarbonate secretion (3,56,57) (Figure 3). In fact, these cells are the only epithelia in the liver to express SR, CFTR and Cl-/HCO3- exchanger AE2 and to respond to secretin with enhanced cAMP levels, Cl- efflux and chloride bicarbonate exchanger (3,56,57). Large (but not small) bile ducts have also been shown to express SSTR2 receptors and to respond to somatostatin with inhibition of cAMP levels, exocytosis and ductal bile secretion (56,70).
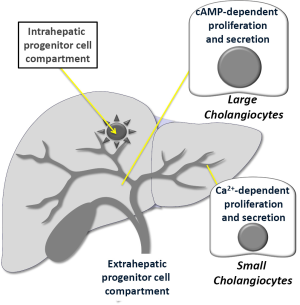
Small cholangiocytes (which do not express CFTR) (3,58) secrete water and electrolytes by activation of Ca2+-dependent pathways (Figure 3). For example, adenosine triphosphate release and purinergic (P2) receptor-mediated secretion has been demonstrated in small mouse cholangiocytes (108). The identification of TMEM16A channels and Ca2+-activated Cl- efflux in small cholangiocytes in response to extracellular nucleotides supports the concept of an alternate, non-cystic fibrosis transmembrane conductance regulator, Cl- channel in cholangiocytes that may be an important compensatory secretory mechanism during damage of larger, cAMP-responsive bile ducts (109). Also, small, mitotically dormant cholangiocytes de novo proliferate and secrete (by amplification of Ca2+-dependent signaling) and acquire markers of large cholangiocytes following damage of these cells for example after treatment with CCl4 or GABA (63-65).
Factors that modulate secretin-stimulated ductal bile secretion
Several studies have shown that secretin-stimulated choleresis is modulated by a number of factors including gastrointestinal hormones, peptides and nerve agonists (104). For example, somatostatin has been shown to inhibit secretin-stimulated ductal bile secretion both in vivo and in vitro by selectively interacting with SSTR2 receptors by downregulation of cAMP-dependent exocytosis (70). While a study has shown that bombesin enhances bile flow, stimulated by infusion of acid into the duodenum by increasing secretin secretion, somatostatin inhibits bile flow produced likely by decreasing the release of secretin from the duodenal mucosa (110). We have shown that gastrin inhibits secretin-induced ductal secretion by interaction with specific receptors (CCK-B) on rat cholangiocytes by down-regulation of SR expression and secretin-stimulated cAMP levels (72,111); gastrin effects were mediated by translocation of Ca2+-dependent PKC isoforms (72,111). Similarly, another study has demonstrated that gamma-interferon decreases collagen content and intrahepatic ductal mass in cirrhotic mice, which was associated with inhibition of secretin-induced choleresis (112). An inhibitory effect on secretin-stimulated choleresis has been demonstrated for the vasoactive peptide, endothelin-1 (ET-1), by interaction with ETA receptors by downregulation of cAMP levels (71). We have demonstrated that insulin receptors are expressed by cholangiocytes, and that insulin inhibits secretin-induced ductal secretion in BDL rats by activation of PKCα and inhibition of secretin-stimulated cAMP levels and PKA activity (113).
With regard to the role of the nervous system in the modulation of biliary secretion, we have shown that: (I) D2 (but not D1 and D3) dopaminergic receptors are expressed by cholangiocytes; and (II) the D2 dopaminergic agonist, quinelorane, decreases the choleretic effect of secretin on bile and bicarbonate secretion, inhibition that was mediated by increased expression of the Ca2+-dependent, PKC gamma, and decreased PKA activity (114). The role of nerve fibers in the modulation of biliary secretion has been supported by additional studies as follows. For example, a recent study has shown that M3 ACh receptors are present in rat cholangiocytes and that acetylcholine potentiated secretin-stimulated ductal secretion by activation of the Cl-/HCO3- exchanger AE2 by a Ca2+-dependent, protein kinase C-insensitive pathway that potentiates the secretin stimulation of adenylyl cyclase (115). Interruption of the parasympathetic system (by total vagotomy in BDL rats) induces biliary apoptosis and functional damage of cholangiocytes with loss of cAMP signaling and secretin-stimulated choleresis (116), that was prevented by the administration of forskolin or feeding bile acids such as taurocholic acid (117) and both ursodeoxycholic and tauroursodeoxycholic acids (118). Also, adrenergic denervation (by the intraportal administration of 6-hydroxydopamine) induces the functional damage of bile ducts with loss of secretin-stimulated choleresis (119), effects that were prevented by the administration of forskolin (an adenylyl cyclase activator), β1- and β2-adrenergic receptor agonists (clenbuterol or dobutamine, respectively) (119) or feeding the bile acid, taurocholic acid (120). A recent study by Francis et al. has shown that cholangiocytes express α2A-, α2B-, and α2C-adrenergic receptors and that the α2-adrenergic receptor agonist, UK14, 304, inhibits secretin-stimulated ductal secretion by downregulation of cAMP signaling in cholestatic rats (73). The α-1 adrenergic receptor agonist, phenylephrine, increases secretin-stimulated cAMP levels and ductal secretion of cholestatic rats by Ca2+-dependent activation of PKCα and βII (74). Thus, coordinated regulation (by stimulatory and inhibitory factors) of secretin-stimulated choleresis may be key in maintaining the functional homeostasis/integrity of the biliary epithelium in pathological conditions associated excessive growth or biliary loss.
Bile acids have also been shown to prevent biliary damage (e.g., by caffeic acid and CCl4) and loss of secretin-stimulated ductal secretion (121). For example, feeding the bile acid, taurocholic acid, to cholestatic rats prevents caffeic acid-induced biliary damage by enhanced cholangiocyte vascular endothelial growth factor (VEGF) expression (121). Also, taurocholic acid feeding prevents tumor necrosis factor-α-induced biliary damage and loss of secretin-induced bicarbonate-rich choleresis by a PI3K-mediated pathway (122).
Regarding the mechanisms of secretin-stimulated ductal secretion, a study has shown that the adenylyl cyclase isoform, AC8, is mostly expressed by large cholangiocytes (123) that are the only cell types expressing the secretin receptors and the target of secretin choleresis (57,124). Thus, this study suggests that AC8 may be a key player in the regulation secretin-induced choleresis in large bile ducts (123). In addition, inhibition of the biliary expression of arylalkylamine N-acetyltransferase (the enzyme key in melatonin synthesis) by administration of AANAT Vivo-Morpholinos increase the autocrine proliferative responses of cholangiocytes and the expression of cAMP/CFTR/Cl-/HCO3- AE2 signaling and ductal secretion (125).
A number of sex hormones have been shown to modulate biliary functions. Following castration in cholestatic rats, testosterone serum levels decreased (prevented by the administration of testosterone), and were associated with reduced biliary proliferation and secretin-stimulated cAMP levels and bile and bicarbonate secretion (126). Also, follicle-stimulating hormone increases biliary proliferation and secretin-stimulated cAMP-dependent bile secretion (127). Some of the factors regulating secretin-stimulated choleresis are also summarized in Table 1.
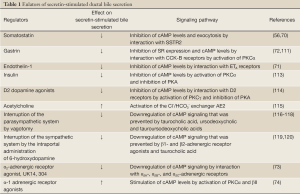
Full Table
Effect of secretin on biliary hyperplasia
A number of studies have suggested that the functional expression of SR may be an index of biliary growth since in models with enhanced biliary hyperplasia there is increased expression of SR and augmented response to secretin (7,8,17,56,62-65,105,127). Conversely, in models of biliary damage/apoptosis (e.g., after CCl4 or GABA treatment) we have observed a reduction of SR expression and choleretic response to secretin (7,8,17,56,62-65,105,127). Recent findings have shown that secretin is a trophic factor for mouse cholangiocytes and that in vivo and in vitro ablation of SR reduces biliary hyperplasia in BDL mice (Figure 4) and in cholangiocyte lines (17). The study suggests the potential use of secretin as a therapy for ductopenic liver diseases (17). Further studies are needed to determine if secretin is an autocrine hormone secreted by cholangiocytes.
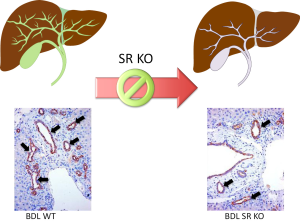
Role of secretin in human biliary diseases
There is growing information regarding the role of secretin and its receptor in the diagnosis and management of biliary disorders. For example, Prieto et al. have used positron emission tomography in humans for evaluating basal and secretin-stimulated biliary bicarbonate secretion as index of functionality of the biliary epithelium in normal and cholestatic (e.g., PBC) conditions (128). Consistent with the importance of secretin in biliary diseases, absence of choleretic response to secretin was observed in cholestatic and untreated PBC patients (128). Recently, secretin-mediated gene delivery has been developed to specifically target the intracellular pathways potentially important for the treatment of biliary and pancreatic disease in cystic fibrosis (9). Secretin stimulated magnetic resonance cholangiopancreatography has been used as diagnostic tool in diseases of the biliary and pancreatic ducts (129). The diagnostic role of secretin-enhanced cholangiopancreatography (MRCP) has been suggested in patients with unsuccessful endoscopic retrograde cholangiopancreatography (ERCP) (130).
Recent studies support the concept that biliary bicarbonate may be an important protective mechanism in cholangiopathies since a defective HCO3- umbrella has been observed in biliary disorders such as in PBC, and primary sclerosing cholangitis (131-134). In support of these findings, a recent study has shown that miR-506 is up-regulated in cholangiocytes from PBC, binds the 3'UTR region of AE2 mRNA, prevents protein translation thus causing diminished AE2 activity and impaired ductal secretion (135). The study suggests that miR-506 may constitute a potential therapeutic target for the management of PBC (135).
Conclusions/future perspectives
We have discussed that secretin and its receptors regulate the secretory activity of a number of organ tissues including the stomach, intestine, pancreas, heart and the biliary epithelium in the liver. Regarding the biliary epithelium, we have shown that secretin and its receptor (only expressed by cholangiocytes in the liver) play key role in the secretory and proliferative activity of large cholangiocytes, the only cell types that express SR and respond to secretin. Small cholangiocytes (which constitutively do not express SR) acquire large cholangiocyte phenotypes in pathological conditions associated with damage of large cholangiocytes and amplify their Ca2+-dependent signaling to compensate for loss of large biliary functions and to maintain the homeostasis of the liver. We have also shown that secretin may be an important trophic autocrine factor that may sustain biliary proliferation during ductopenic states. It has also suggested the diagnostic role of secretin in biliary diseases such as PBC and biliary atresia. Further studies are necessary to determine the prognostic role of secretin in the diagnosis of ductopenic biliary diseases.
Acknowledgements
Portion of this work was supported by the Dr. Nicholas C. Hightower Centennial Chair of Gastroenterology from Scott & White Hospital, a VA Merit award and the NIH grant DK58411, DK062975 to Drs. Alpini and Glaser, DK081442 and Veterans Administration CDA-2 Award to Dr. Glaser.
Disclosure: The authors declare no conflict of interest.
References
- Bayliss WM, Starling EH. The mechanism of pancreatic secretion. J Physiol 1902;28:325-53. [PubMed]
- Häcki WH. Secretin. Clin Gastroenterol 1980;9:609-32. [PubMed]
- Alpini G, Glaser S, Robertson W, et al. Large but not small intrahepatic bile ducts are involved in secretin-regulated ductal bile secretion. Am J Physiol 1997;272:G1064-74. [PubMed]
- Chu JY, Cheng CY, Lee VH, et al. Secretin and body fluid homeostasis. Kidney Int 2011;79:280-7. [PubMed]
- Dong M, Miller LJ. Molecular pharmacology of the secretin receptor. Receptors Channels 2002;8:189-200. [PubMed]
- Ishiguro H, Yamamoto A, Nakakuki M, et al. Physiology and pathophysiology of bicarbonate secretion by pancreatic duct epithelium. Nagoya J Med Sci 2012;74:1-18. [PubMed]
- Alpini G, Lenzi R, Sarkozi L, et al. Biliary physiology in rats with bile ductular cell hyperplasia. Evidence for a secretory function of proliferated bile ductules. J Clin Invest 1988;81:569-78. [PubMed]
- Alpini G, Ulrich CD 2nd, Phillips JO, et al. Upregulation of secretin receptor gene expression in rat cholangiocytes after bile duct ligation. Am J Physiol 1994;266:G922-8. [PubMed]
- McKay T, Reynolds P, Jezzard S, et al. Secretin-mediated gene delivery, a specific targeting mechanism with potential for treatment of biliary and pancreatic disease in cystic fibrosis. Mol Ther 2002;5:447-54. [PubMed]
- Chow BK, Cheung KH, Tsang EM, et al. Secretin controls anion secretion in the rat epididymis in an autocrine/paracrine fashion. Biol Reprod 2004;70:1594-9. [PubMed]
- Onori P, Wise C, Gaudio E, et al. Secretin inhibits cholangiocarcinoma growth via dysregulation of the cAMP-dependent signaling mechanisms of secretin receptor. Int J Cancer 2010;127:43-54. [PubMed]
- Schubert ML. Hormonal regulation of gastric acid secretion. Curr Gastroenterol Rep 2008;10:523-7. [PubMed]
- Alpini G, Ueno Y, Glaser SS, et al. Bile acid feeding increased proliferative activity and apical bile acid transporter expression in both small and large rat cholangiocytes. Hepatology 2001;34:868-76. [PubMed]
- Nishijima I, Yamagata T, Spencer CM, et al. Secretin receptor-deficient mice exhibit impaired synaptic plasticity and social behavior. Hum Mol Genet 2006;15:3241-50. [PubMed]
- Siu FK, Lam IP, Chu JY, et al. Signaling mechanisms of secretin receptor. Regul Pept 2006;137:95-104. [PubMed]
- Yamagata T, Urano H, Weeber EJ, et al. Impaired hippocampal synaptic function in secretin deficient mice. Neuroscience 2008;154:1417-22. [PubMed]
- Glaser S, Lam IP, Franchitto A, et al. Knockout of secretin receptor reduces large cholangiocyte hyperplasia in mice with extrahepatic cholestasis induced by bile duct ligation. Hepatology 2010;52:204-14. [PubMed]
- Portela-Gomes GM, Johansson H, Olding L, et al. Co-localization of neuroendocrine hormones in the human fetal pancreas. Eur J Endocrinol 1999;141:526-33. [PubMed]
- Mutt V, Jorpes JE, Magnusson S. Structure of porcine secretin. The amino acid sequence. Eur J Biochem 1970;15:513-9. [PubMed]
- Whitmore TE, Holloway JL, Lofton-Day CE, et al. Human secretin (SCT): gene structure, chromosome location, and distribution of mRNA. Cytogenet Cell Genet 2000;90:47-52. [PubMed]
- Lan MS, Kajiyama W, Donadel G, et al. cDNA sequence and genomic organization of mouse secretin. Biochem Biophys Res Commun 1994;200:1066-71. [PubMed]
- Bonetto V, Jörnvall H, Mutt V, et al. Two alternative processing pathways for a preprohormone: a bioactive form of secretin. Proc Natl Acad Sci U S A 1995;92:11985-9. [PubMed]
- Gafvelin G, Jörnvall H, Mutt V. Processing of prosecretin: isolation of a secretin precursor from porcine intestine. Proc Natl Acad Sci U S A 1990;87:6781-5. [PubMed]
- Kopin AS, Wheeler MB, Leiter AB. Secretin: structure of the precursor and tissue distribution of the mRNA. Proc Natl Acad Sci U S A 1990;87:2299-303. [PubMed]
- Williams RH. eds. Textbook of endocrinology. 6th ed. Philadelphia: Saunders, 1981: xxiii, 1270.
- DeGroot LJ. eds. Endocrinology. 2nd ed. Philadelphia: Saunders, 1989.
- Roy A, Kucukural A, Zhang Y. I-TASSER: a unified platform for automated protein structure and function prediction. Nat Protoc 2010;5:725-38. [PubMed]
- Zhang Y. I-TASSER server for protein 3D structure prediction. BMC Bioinformatics 2008;9:40. [PubMed]
- Polak JM, Coulling I, Bloom S, et al. Immunofluorescent localization of secretin and enteroglucagon in human intestinal mucosa. Scand J Gastroenterol 1971;6:739-44. [PubMed]
- Samson WK, Lumpkin MD, Vijayan E, et al. Gastrointestinal hormones: central nervous system localization and sites of neuroendocrine actions. Endocrinol Exp 1982;16:177-89. [PubMed]
- Wang T, Palkovits M, Rusnak M, et al. Distribution of parathyroid hormone-2 receptor-like immunoreactivity and messenger RNA in the rat nervous system. Neuroscience 2000;100:629-49. [PubMed]
- Charlton CG, O’Donohue TL, Miller RL, et al. Secretin immunoreactivity in rat and pig brain. Peptides 1981;2:45-9. [PubMed]
- Samson WK, Lumpkin MD, McCann SM. Presence and possible site of action of secretin in the rat pituitary and hypothalamus. Life Sci 1984;34:155-63. [PubMed]
- Fremeau RT Jr, Jensen RT, Charlton CG, et al. Secretin: specific binding to rat brain membranes. J Neurosci 1983;3:1620-5. [PubMed]
- Yung WH, Leung PS, Ng SS, et al. Secretin facilitates GABA transmission in the cerebellum. J Neurosci 2001;21:7063-8. [PubMed]
- Ohta M, Funakoshi S, Kawasaki T, et al. Tissue-specific expression of the rat secretin precursor gene. Biochem Biophys Res Commun 1992;183:390-5. [PubMed]
- Glaser S, Gaudio E, Onori P, et al. Knockout of the gene for secretin inhibits biliary hyperplasia of large cholangiocytes in cholestatic mice by an autocrine mechanism. Hepatology 2009;50:A1075.
- Ishihara T, Nakamura S, Kaziro Y, et al. Molecular cloning and expression of a cDNA encoding the secretin receptor. EMBO J 1991;10:1635-41. [PubMed]
- Kopin AS, Wheeler MB, Nishitani J, et al. The secretin gene: evolutionary history, alternative splicing, and developmental regulation. Proc Natl Acad Sci U S A 1991;88:5335-9. [PubMed]
- Carstairs JR, Barnes PJ. Visualization of vasoactive intestinal peptide receptors in human and guinea pig lung. J Pharmacol Exp Ther 1986;239:249-55. [PubMed]
- Charlton CG, Quirion R, Handelmann GE, et al. Secretin receptors in the rat kidney: adenylate cyclase activation and renal effects. Peptides 1986;7:865-71. [PubMed]
- Christophe J, Waelbroeck M, Chatelain P, et al. Heart receptors for VIP, PHI and secretin are able to activate adenylate cyclase and to mediate inotropic and chronotropic effects. Species variations and physiopathology. Peptides 1984;5:341-53. [PubMed]
- Fremeau RT Jr, Korman LY, Moody TW. Secretin stimulates cyclic AMP formation in the rat brain. J Neurochem 1986;46:1947-55. [PubMed]
- Isenberg JI, Wallin B, Johansson C, et al. Secretin, VIP, and PHI stimulate rat proximal duodenal surface epithelial bicarbonate secretion in vivo. Regul Pept 1984;8:315-20. [PubMed]
- Christodoulopoulos JB, Jacobs WH, Klotz AP. Action of secretin on pancreatic secretion. Am J Physiol 1961;201:1020-4. [PubMed]
- Jones RS, Geist RE, Hall AD. The choleretic effects of glucagon and secretin in the dog. Gastroenterology 1971;60:64-8. [PubMed]
- Sanders MJ, Amirian DA, Ayalon A, et al. Regulation of pepsinogen release from canine chief cells in primary monolayer culture. Am J Physiol 1983;245:G641-6. [PubMed]
- Straus E, Greenstein AJ, Yalow RS. Effect of secretin on release of heterogeneous forms of gastrin. Gut 1975;16:999-1005. [PubMed]
- Hubel KA. Secretin: a long progress note. Gastroenterology 1972;62:318-41. [PubMed]
- Kraegen EW, Chisholm DJ, Young JD, et al. The gastrointestinal stimulus to insulin release. II. A dual action of secretin. J Clin Invest 1970;49:524-9. [PubMed]
- Patel DR, Kong Y, Sreedharan SP. Molecular cloning and expression of a human secretin receptor. Mol Pharmacol 1995;47:467-73. [PubMed]
- Davis RJ, Page KJ, Dos Santos Cruz GJ, et al. Expression and functions of the duodenal peptide secretin and its receptor in human lung. Am J Respir Cell Mol Biol 2004;31:302-8. [PubMed]
- Yuan Y, Lee LT, Ng SS, et al. Extragastrointestinal functions and transcriptional regulation of secretin and secretin receptors. Ann N Y Acad Sci 2011;1220:23-33. [PubMed]
- Tay J, Goulet M, Rusche J, et al. Age-related and regional differences in secretin and secretin receptor mRNA levels in the rat brain. Neurosci Lett 2004;366:176-81. [PubMed]
- Steiner TS, Mangel AW, McVey DC, et al. Secretin receptors mediating rat forestomach relaxation. Am J Physiol 1993;264:G863-7. [PubMed]
- Alpini G, Glaser SS, Ueno Y, et al. Heterogeneity of the proliferative capacity of rat cholangiocytes after bile duct ligation. Am J Physiol 1998;274:G767-75. [PubMed]
- Alpini G, Roberts S, Kuntz SM, et al. Morphological, molecular, and functional heterogeneity of cholangiocytes from normal rat liver. Gastroenterology 1996;110:1636-43. [PubMed]
- Alpini G, Ulrich C, Roberts S, et al. Molecular and functional heterogeneity of cholangiocytes from rat liver after bile duct ligation. Am J Physiol 1997;272:G289-97. [PubMed]
- Farouk M, Vigna SR, McVey DC, et al. Localization and characterization of secretin binding sites expressed by rat bile duct epithelium. Gastroenterology 1992;102:963-8. [PubMed]
- Farouk M, Vigna SR, Haebig JE, et al. Secretin receptors in a new preparation of plasma membranes from intrahepatic biliary epithelium. J Surg Res 1993;54:1-6. [PubMed]
- Kato A, Gores GJ, LaRusso NF. Secretin stimulates exocytosis in isolated bile duct epithelial cells by a cyclic AMP-mediated mechanism. J Biol Chem 1992;267:15523-9. [PubMed]
- Lesage G, Glaser S, Ueno Y, et al. Regression of cholangiocyte proliferation after cessation of ANIT feeding is coupled with increased apoptosis. Am J Physiol Gastrointest Liver Physiol 2001;281:G182-90. [PubMed]
- LeSage GD, Benedetti A, Glaser S, et al. Acute carbon tetrachloride feeding selectively damages large, but not small, cholangiocytes from normal rat liver. Hepatology 1999;29:307-19. [PubMed]
- LeSage GD, Glaser SS, Marucci L, et al. Acute carbon tetrachloride feeding induces damage of large but not small cholangiocytes from BDL rat liver. Am J Physiol 1999;276:G1289-301. [PubMed]
- Mancinelli R, Franchitto A, Gaudio E, et al. After damage of large bile ducts by gamma-aminobutyric acid, small ducts replenish the biliary tree by amplification of calcium-dependent signaling and de novo acquisition of large cholangiocyte phenotypes. Am J Pathol 2010;176:1790-800. [PubMed]
- Wang X, Ye H, Ward CJ, et al. Insignificant effect of secretin in rodent models of polycystic kidney and liver disease. Am J Physiol Renal Physiol 2012;303:F1089-98. [PubMed]
- Körner M, Hayes GM, Rehmann R, et al. Secretin receptors in the human liver: expression in biliary tract and cholangiocarcinoma, but not in hepatocytes or hepatocellular carcinoma. J Hepatol 2006;45:825-35. [PubMed]
- Alvaro D, Cho WK, Mennone A, et al. Effect of secretion on intracellular pH regulation in isolated rat bile duct epithelial cells. J Clin Invest 1993;92:1314-25. [PubMed]
- Soleimani M. Impaired pancreatic ductal bicarbonate secretion in cystic fibrosis. JOP 2001;2:237-42. [PubMed]
- Tietz PS, Alpini G, Pham LD, et al. Somatostatin inhibits secretin-induced ductal hypercholeresis and exocytosis by cholangiocytes. Am J Physiol 1995;269:G110-8. [PubMed]
- Caligiuri A, Glaser S, Rodgers RE, et al. Endothelin-1 inhibits secretin-stimulated ductal secretion by interacting with ETA receptors on large cholangiocytes. Am J Physiol 1998;275:G835-46. [PubMed]
- Glaser SS, Rodgers RE, Phinizy JL, et al. Gastrin inhibits secretin-induced ductal secretion by interaction with specific receptors on rat cholangiocytes. Am J Physiol 1997;273:G1061-70. [PubMed]
- Francis H, LeSage G, DeMorrow S, et al. The alpha2-adrenergic receptor agonist UK 14,304 inhibits secretin-stimulated ductal secretion by downregulation of the cAMP system in bile duct-ligated rats. Am J Physiol Cell Physiol 2007;293:C1252-62. [PubMed]
- LeSage GD, Alvaro D, Glaser S, et al. Alpha-1 adrenergic receptor agonists modulate ductal secretion of BDL rats via Ca(2+)- and PKC-dependent stimulation of cAMP. Hepatology 2004;40:1116-27. [PubMed]
- Ding WQ, Cheng ZJ, McElhiney J, et al. Silencing of secretin receptor function by dimerization with a misspliced variant secretin receptor in ductal pancreatic adenocarcinoma. Cancer Res 2002;62:5223-9. [PubMed]
- Körner MU, Hayes GM, Carrigan PE, et al. Wild-type and splice-variant secretin receptors in lung cancer: overexpression in carcinoid tumors and peritumoral lung tissue. Mod Pathol 2008;21:387-95. [PubMed]
- Ding WQ, Kuntz S, Böhmig M, et al. Dominant negative action of an abnormal secretin receptor arising from mRNA missplicing in a gastrinoma. Gastroenterology 2002;122:500-11. [PubMed]
- Körner M, Hayes GM, Rehmann R, et al. Secretin receptors in normal and diseased human pancreas: marked reduction of receptor binding in ductal neoplasia. Am J Pathol 2005;167:959-68. [PubMed]
- Li P, Chang TM, Chey WY. Secretin inhibits gastric acid secretion via a vagal afferent pathway in rats. Am J Physiol 1998;275:G22-8. [PubMed]
- Shimizu K, Li P, Lee KY, et al. The mechanism of inhibitory action of secretin on gastric acid secretion in conscious rats. J Physiol 1995;488:501-8. [PubMed]
- Chung I, Li P, Lee K, et al. Dual inhibitory mechanism of secretin action on acid secretion in totally isolated, vascularly perfused rat stomach. Gastroenterology 1994;107:1751-8. [PubMed]
- Jordan PH Jr, Peterson ND. Effects of secretin upon gastric secretion. Ann Surg 1962;156:914-23. [PubMed]
- Osnes M, Hanssen LE, Flaten O, et al. Exocrine pancreatic secretion and immunoreactive secretin (IRS) release after intraduodenal instillation of bile in man. Gut 1978;19:180-4. [PubMed]
- Rominger JM, Chey WY, Chang TM. Plasma secretin concentrations and gastric pH in healthy subjects and patients with digestive diseases. Dig Dis Sci 1981;26:591-7. [PubMed]
- Pascua P, García M, Fernández-Salazar MP, et al. Ducts isolated from the pancreas of CFTR-null mice secrete fluid. Pflugers Arch 2009;459:203-14. [PubMed]
- Cheng CY, Chu JY, Chow BK. Vasopressin-independent mechanisms in controlling water homeostasis. J Mol Endocrinol 2009;43:81-92. [PubMed]
- Nielsen S, DiGiovanni SR, Christensen EI, et al. Cellular and subcellular immunolocalization of vasopressin-regulated water channel in rat kidney. Proc Natl Acad Sci U S A 1993;90:11663-7. [PubMed]
- Nielsen S, Chou CL, Marples D, et al. Vasopressin increases water permeability of kidney collecting duct by inducing translocation of aquaporin-CD water channels to plasma membrane. Proc Natl Acad Sci U S A 1995;92:1013-7. [PubMed]
- Baron DN, Newman F, Warrick A. The effects of secretin on urinary volume and electrolytes in normal subjects and patients with chronic pancreatic disease. Experientia 1957;14:30-32.
- Viteri AL, Poppell JW, Lasater JM, et al. Renal response to secretin. J Appl Physiol 1975;38:661-4. [PubMed]
- Waldum HL, Sundsfjord JA, Aanstad U, et al. The effect of secretin on renal haemodynamics in man. Scand J Clin Lab Invest 1980;40:475-8. [PubMed]
- Barbezat GO, Isenberg JI, Grossman MI. Diuretic action of secretin in dog. Proc Soc Exp Biol Med 1972;139:211-5. [PubMed]
- Lossi L, Bottarelli L, Candusso ME, et al. Transient expression of secretin in serotoninergic neurons of mouse brain during development. Eur J Neurosci 2004;20:3259-69. [PubMed]
- Yang B, Goulet M, Boismenu R, et al. Secretin depolarizes nucleus tractus solitarius neurons through activation of a nonselective cationic conductance. Am J Physiol Regul Integr Comp Physiol 2004;286:R927-34. [PubMed]
- Campbell G. Cotransmission. Annu Rev Pharmacol Toxicol 1987;27:51-70. [PubMed]
- Guyton AC. eds. Rythmical excitation of the heart. Human Physiolology of Mechanisms of Disease, 5 ed. Philadelphia: Saunders, 1992:89-94.
- Hökfelt T, Johansson O, Ljungdahl A, et al. Peptidergic neurones. Nature 1980;284:515-21. [PubMed]
- Gozes I, Brenneman DE. VIP: molecular biology and neurobiological function. Mol Neurobiol 1989;3:201-36. [PubMed]
- Chatelain P, Robberecht P, de Neef P, et al. Low responsiveness of cardiac adenylate cyclase activity to peptide hormones in spontaneously hypertensive rats. FEBS Lett 1979;107:86-90. [PubMed]
- Robberecht P, Gillet L, Chatelain P, et al. Specific decrease of secretin/VIP-stimulated adenylate cyclase in the heart from the Lyon strain of hypertensive rats. Peptides 1984;5:355-8. [PubMed]
- Chatelain P, Robberecht P, De Neef P, et al. Impairment of hormone-stimulated cardiac adenylate cyclase activity in the genetically obese (fa/fa) Zucker rat. Pflugers Arch 1981;390:10-6. [PubMed]
- Ross G. Cardiovascular effects of secretin. Am J Physiol 1970;218:1166-70. [PubMed]
- Chiba S. Effect of secretin on pacemaker activity and contractility in the isolated blood-perfused atrium of the dog. Clin Exp Pharmacol Physiol 1976;3:167-72. [PubMed]
- Kanno N, LeSage G, Glaser S, et al. Regulation of cholangiocyte bicarbonate secretion. Am J Physiol Gastrointest Liver Physiol 2001;281:G612-25. [PubMed]
- Francis H, Glaser S, Ueno Y, et al. cAMP stimulates the secretory and proliferative capacity of the rat intrahepatic biliary epithelium through changes in the PKA/Src/MEK/ERK1/2 pathway. J Hepatol 2004;41:528-37. [PubMed]
- Banales JM, Arenas F, Rodríguez-Ortigosa CM, et al. Bicarbonate-rich choleresis induced by secretin in normal rat is taurocholate-dependent and involves AE2 anion exchanger. Hepatology 2006;43:266-75. [PubMed]
- Glaser SS, Gaudio E, Rao A, et al. Morphological and functional heterogeneity of the mouse intrahepatic biliary epithelium. Lab Invest 2009;89:456-69. [PubMed]
- Woo K, Sathe M, Kresge C, et al. Adenosine triphosphate release and purinergic (P2) receptor-mediated secretion in small and large mouse cholangiocytes. Hepatology 2010;52:1819-28. [PubMed]
- Dutta AK, Khimji AK, Kresge C, et al. Identification and functional characterization of TMEM16A, a Ca2+-activated Cl- channel activated by extracellular nucleotides, in biliary epithelium. J Biol Chem 2011;286:766-76. [PubMed]
- Kaminski DL, Deshpande YG. Effect of somatostatin and bombesin on secretin-stimulated ductular bile flow in dogs. Gastroenterology 1983;85:1239-47. [PubMed]
- Glaser S, Alvaro D, Ueno Y, et al. Gastrin reverses established cholangiocyte proliferation and enhanced secretin-stimulated ductal secretion of BDL rats by activation of apoptosis through increased expression of Ca2+- dependent PKC isoforms. Liver Int 2003;23:78-88. [PubMed]
- Alpini G, Elias I, Glaser SS, et al. gamma-Interferon inhibits secretin-induced choleresis and cholangiocyte proliferation in a murine model of cirrhosis. J Hepatol 1997;27:371-80. [PubMed]
- Lesage GD, Marucci L, Alvaro D, et al. Insulin inhibits secretin-induced ductal secretion by activation of PKC alpha and inhibition of PKA activity. Hepatology 2002;36:641-51. [PubMed]
- Glaser S, Alvaro D, Roskams T, et al. Dopaminergic inhibition of secretin-stimulated choleresis by increased PKC-gamma expression and decrease of PKA activity. Am J Physiol Gastrointest Liver Physiol 2003;284:G683-94. [PubMed]
- Alvaro D, Alpini G, Jezequel AM, et al. Role and mechanisms of action of acetylcholine in the regulation of rat cholangiocyte secretory functions. J Clin Invest 1997;100:1349-62. [PubMed]
- LeSagE G, Alvaro D, Benedetti A, et al. Cholinergic system modulates growth, apoptosis, and secretion of cholangiocytes from bile duct-ligated rats. Gastroenterology 1999;117:191-9. [PubMed]
- Marzioni M, LeSage GD, Glaser S, et al. Taurocholate prevents the loss of intrahepatic bile ducts due to vagotomy in bile duct-ligated rats. Am J Physiol Gastrointest Liver Physiol 2003;284:G837-52. [PubMed]
- Marzioni M, Francis H, Benedetti A, et al. Ca2+-dependent cytoprotective effects of ursodeoxycholic and tauroursodeoxycholic acid on the biliary epithelium in a rat model of cholestasis and loss of bile ducts. Am J Pathol 2006;168:398-409. [PubMed]
- Glaser S, Alvaro D, Francis H, et al. Adrenergic receptor agonists prevent bile duct injury induced by adrenergic denervation by increased cAMP levels and activation of Akt. Am J Physiol Gastrointest Liver Physiol 2006;290:G813-26. [PubMed]
- Marzioni M, Ueno Y, Glaser S, et al. Cytoprotective effects of taurocholic acid feeding on the biliary tree after adrenergic denervation of the liver. Liver Int 2007;27:558-68. [PubMed]
- Mancinelli R, Onori P, Gaudio E, et al. Taurocholate feeding to bile duct ligated rats prevents caffeic acid-induced bile duct damage by changes in cholangiocyte VEGF expression. Exp Biol Med (Maywood) 2009;234:462-74. [PubMed]
- Ueno Y, Francis H, Glaser S, et al. Taurocholic acid feeding prevents tumor necrosis factor-alpha-induced damage of cholangiocytes by a PI3K-mediated pathway. Exp Biol Med (Maywood) 2007;232:942-9. [PubMed]
- Strazzabosco M, Fiorotto R, Melero S, et al. Differentially expressed adenylyl cyclase isoforms mediate secretory functions in cholangiocyte subpopulation. Hepatology 2009;50:244-52. [PubMed]
- Alpini G, Ulrich C, Roberts S, et al. Molecular and functional heterogeneity of cholangiocytes from rat liver after bile duct ligation. Am J Physiol 1997;272:G289-97. [PubMed]
- Renzi A, Demorrow S, Onori P, et al. Modulation of the biliary expression of arylalkylamine N-acetyltransferase alters the autocrine proliferative responses of cholangiocytes. Hepatology 2013;57:1130-41. [PubMed]
- Yang F, Priester S, Onori P, et al. Castration inhibits biliary proliferation induced by bile duct obstruction: novel role for the autocrine trophic effect of testosterone. Am J Physiol Gastrointest Liver Physiol 2011;301:G981-91. [PubMed]
- Mancinelli R, Onori P, Gaudio E, et al. Follicle-stimulating hormone increases cholangiocyte proliferation by an autocrine mechanism via cAMP-dependent phosphorylation of ERK1/2 and Elk-1. Am J Physiol Gastrointest Liver Physiol 2009;297:G11-26. [PubMed]
- Prieto J, García N, Martí-Climent JM, et al. Assessment of biliary bicarbonate secretion in humans by positron emission tomography. Gastroenterology 1999;117:167-72. [PubMed]
- Hellund JC, Geitung JT, Meo AM, et al. Secretin stimulated magnetic resonance cholangiopancreatography in diseases of the biliary and pancreatic ducts. Tidsskr Nor Laegeforen 2002;122:691-4. [PubMed]
- Czakó L, Takács T, Morvay Z, et al. Diagnostic role of secretin-enhanced MRCP in patients with unsuccessful ERCP. World J Gastroenterol 2004;10:3034-8. [PubMed]
- Hohenester S, Maillette de Buy Wenniger L, Jefferson DM, et al. Biliary bicarbonate secretion constitutes a protective mechanism against bile acid-induced injury in man. Dig Dis 2011;29:62-5. [PubMed]
- Hohenester S, Wenniger LM, Paulusma CC, et al. A biliary HCO3- umbrella constitutes a protective mechanism against bile acid-induced injury in human cholangiocytes. Hepatology 2012;55:173-83. [PubMed]
- Erlinger S. Chronic fibrosing cholangiopathies: a consequence of a defective HCO3- “umbrella”? Clin Res Hepatol Gastroenterol 2011;35:85-8. [PubMed]
- Beuers U, Hohenester S, de Buy Wenniger LJ, et al. The biliary HCO(3)(-) umbrella: a unifying hypothesis on pathogenetic and therapeutic aspects of fibrosing cholangiopathies. Hepatology 2010;52:1489-96. [PubMed]
- Banales JM, Sáez E, Uriz M, et al. Up-regulation of microRNA 506 leads to decreased Cl-/HCO3- anion exchanger 2 expression in biliary epithelium of patients with primary biliary cirrhosis. Hepatology 2012;56:687-97. [PubMed]