Protease activated receptor 4: a backup receptor or a dark horse as a target in antiplatelet therapy?
Platelets are the primary component of pathological arterial thrombi. As such, antiplatelet therapy is a key strategy for treatment of acute coronary syndrome (ACS). The recent publication by Wong and colleagues earlier this year reported a compound, BMS-986120, that targets protease activated receptor 4 (PAR4) in a reversible manner (1). BMS-986120 effectively inhibits thrombus formation with minimal bleeding complications in their animal model (1). Their paper demonstrates the potential of targeting PAR4 as a promising antiplatelet therapeutic approach (1).
Platelet activation
To achieve the delicate balance between maintaining free flow of blood and, when necessary, platelet aggregation at the site of damage, platelet activation and aggregation needs to be precisely regulated. This requires accurate coordination of many molecules and processes. Once an injury breaks the endothelium, the A1 domain of the von Willebrand factor binds to the ligand binding domain of the GPIbα in GPIb-IX-V complex on the surface of the platelet in order to slow down and to facilitate platelet adhesion (2). This “handshake” between the rapid moving platelet and relatively still blood vessel also generates a shear force that is between 10–20 pN (3). This force is strong enough to unfold the mechanosensory domain of the GPIbα, which induces GPIb signaling in platelets through the PI3K-Src pathway (Figure 1A) (2). Meanwhile, collagen also binds to one of its receptors, Glycoprotein VI (GPVI)-FcRγ, to recruit LAT as an adaptor protein to induce downstream signaling (4). Both GPIb and GPVI signaling can activate phospholipase Cγ (PLCγ) mediated granule release, to form unstable plug (4). Damaged endothelium results in exposure of collagen and tissue factor, which initiates thrombin generation (5). Activation of platelets by thrombin results in platelet granule release increasing the local concentration of ADP and thromboxane A2 (TxA2) around the injury site for further platelet activation (5). These agonists signal through series G-protein coupled receptors (GPCRs) on the surface of the platelet, including protease activated receptor 1 and 4 (PAR1 and PAR4) (thrombin receptors), P2Y1 and P2Y12 (ADP receptors), and thromboxane receptor (TP) (4). These platelet GPCRs couple to multiple G-proteins (Gq, G12/13, Gi and Gs) to facilitate platelet activation and thus accelerate thrombus formation (Figure 1A) (4,6). This several highly-coordinated series of events between GPIb, GPVI and GPCR signaling provides potential therapeutic targets to prevent unwanted clots.
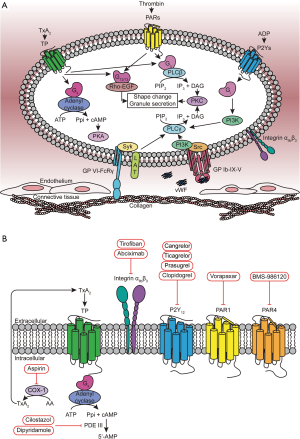
Current platelet therapies
The current antiplatelet agents can be divided into three general categories based on their mechanism of action (Figure 1B). The first category is comprised of compounds that target platelet signaling pathways and include irreversible cyclooxygenase (COX) inhibitors, phosphodiesterase (PDE) inhibitors, and adenosine reuptake inhibitors. Aspirin is one of the most commonly prescribed antiplatelet therapies for extended intervention. Its mechanism of action is covalent acetylation of COX thereby irreversibly decreasing the generation of TxA2 in platelets (7). Cilostazol (brand name Pletal) is a prodrug whose metabolites inhibit cAMP PDE III (8). Cilostazol increases the concentration of cAMP to inhibit platelet aggregation by blocking the degradation of cAMP in the cytosol of platelets (8). The adenosine reuptake inhibitor, dipyridamole (brand name Persantine), prevents platelet aggregation through the similar mechanism of action (9). Several therapeutics, such as Integrin αIIbβ3 (also known as Glycoprotein IIb/IIIa, GPIIb/IIIa) inhibitors, fall into the second category that target platelet adhesion. The αIIbβ3 inhibitor abciximab (brand name ReoPro) is a Fab fragment of the human monoclonal antibody 7E3 that binds to αIIbβ3 to block its interaction with fibrinogen and vWF for essential platelet adhesion (10). Abciximab can only be administrated intravenously, which limits its use to hospital setting. Small molecules can also be used to block integrin signaling. Tirofiban (brand name Aggrastat) is a reversible non-peptide antagonist of αIIbβ3 (10). Unfortunately, αIIbβ3 is the primary adhesion molecule, whose inhibition leads to bleeding complications. The third category of antiplatelet compounds is receptor inhibitors, such as ADP receptor and PAR1 antagonists. There are two types of P2Y12 antagonist: thienopyridines (clopidogrel and prasugrel) and the nonthienopyridines (ticagrelor and cangrelor). All of these compounds, except ticagrelor, are irreversible antagonists of P2Y12, which can make patient management challenging. In addition, clopidogrel is a prodrug that needs to be metabolized by several CYP450 enzymes, such as CYP2C19, to generate the active metabolites (11,12). Polymorphisms of CYP2C19 (e.g., 681G to A) impairs the metabolism of clopidogrel; these patients are non-responders (12). Nevertheless, clopidogrel is still the first-line ADP receptor inhibitor that is normally prescribed together with aspirin routinely as the standard of care unless patients show a high risk of bleeding. The first PAR1 antagonist, vorapaxar (brand name Zontivity) was approved by FDA in 2014 (13). However, this is not widely used clinically due to its pharmacodynamic profile leading to increased bleeding risk. Despite its practical difficulties, co-administration of both aspirin and a P2Y12 antagonist is the standard of care antiplatelet therapy for ACS. Since there are individuals who do not respond to this therapeutic approach, developing novel targets is still necessary. The publication by Wong et al., earlier this year highlighted the possibility of targeting the second thrombin receptor, PAR4, as a safe and effective antiplatelet therapy (1).
Dual-receptor system for thrombin signaling on human platelet
Thrombin is the most potent platelet agonist in vivo and signals through two GPCRs on the surface of human platelets, PAR1 and PAR4 (14). Thrombin activates these receptors by cleaving the N-terminus of PAR1 and PAR4 to unmask their tethered ligands, which interact with their binding pocket at extracellular side (Figure 2A) (15). In platelets, PAR1 and PAR4 couple to Gq and G12/13. The G-protein activation further promotes platelet shape change and granule secretion (16). At first glance, the dual-receptor system may appear to be an evolutionary redundant. However, PAR1 and PAR4 have functional differences that are complementary and both are essential for platelet activation (17). PAR1 can be activated by low thrombin concentrations to induce a rapid and transient calcium flux that is rapidly desensitized (18). PAR1 mainly contributes to unstable platelet aggregation (18). PAR4, on the other hand, requires a higher concentration of thrombin to be activated and initiates sustained calcium signaling, which makes it essential for stable plug generation (18). Moreover, the sustained signaling from PAR4 induces Rap1 activation, which further triggers the integrin αIIbβ3 inside-out signaling (16,19) (Figure 2A).
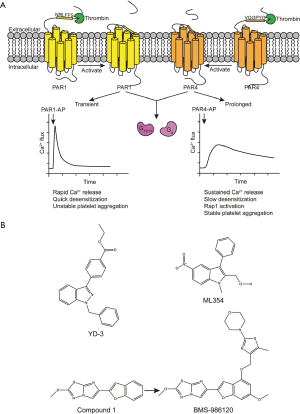
Targeting PAR4 therapeutically
PAR4 has received little consideration as a target for antiplatelet therapy. Most attention to date has focused on PAR1. As discussed above, PAR1 responds to low concentration of thrombin and PAR4 has long been thought of as a backup, or in some cases, an unnecessary receptor. However, an interesting hypothesis is that PAR4 inhibition may, in fact, be safer and more effective given its signaling kinetics. Blocking the sustained signaling from PAR4 may prevent unwanted stable thrombus growth, while leaving PAR1 transient signaling to preserve initial thrombus formation.
The few efforts to block PAR4 have focused on inhibitory antibodies, intracellular peptides, and small molecules. In the context of small molecules, the indazole core structure shows promising PAR4 antagonism activity. This chemical scaffold was used in discovery of YD-3 and its derivatives (Figure 2B, top panel). 1-benzyl-3-(ethoxycarbonylphenyl)-indazole (YD-3) was the first non-peptide PAR4 antagonist (20). It selectively inhibits washed human platelet aggregation induced by GYPGKF (IC50 =130 nM) in a competitive manner but only has a limited inhibitory effect on aggregation induced by thrombin, SFLLRN, collagen or U46619 (20). YD-3 inhibits thrombin-induced activation more significantly when PAR1 is desensitized (21). This agent selectively inhibits PAR4-induced platelet activation across species (20,21). However, the data from mouse and rabbit experiments need to be interpreted with caution because the platelet PAR expression profile across species is different. The research groups of Hamm and Lindsley are also developing PAR4 antagonist by library screening and organic chemistry optimization to achieve compounds with better drug metabolism and pharmacokinetics (DMPK) profiles (22-24). One of their agents, ML354, was discovered with an IC50 of 140 nM and a selectivity for PAR4 over PAR1 (22-24). Unfortunately, neither of these two compounds has been approved for any clinical trial. Currently, both ML354 and YD-3 still serve as experimental tools in research field to facilitate further characterization of PAR4.
BMS-986120
In early 2017, Wong et al. reported a reversible PAR4 antagonist, BMS-986120 (BMS) that selectively binds to PAR4 as a potent and highly efficacious antithrombotic reagent (1). In their paper published in Science Translational Medicine, Wong et al. performed a high-throughput screening of 1.1 million compounds from the Bristol-Myers-Squibb (BMS) library using a PAR4 activation peptide (PAR4-AP)-induced calcium signaling as a read out. This screen resulted in the discovery of imidazothiadiazole compound 1 (Figure 2B, bottom panel). Optimization of this lead compound led to BMS-986120, which has better oral bioavailability with higher potency and selectivity (1). Preliminary in vitro experiments determined that BMS-986120 has high binding affinity to PAR4 expressed on HEK293 cells and inhibition of PAR4-induced calcium mobilization with an IC50 of 0.56 nM (1). Further studies showed that it selectively inhibits multiple signaling events downstream of PAR4 and does not have agonist activity (1). With these promising results on cell lines, they next turned their attention to human platelets. BMS-986120 blocked human platelet activation in PRP by γ-thrombin and PAR4-AP with an IC50 <10 nM. The selectivity of BMS-986120 for PAR4 over PAR1 was confirmed in calcium signaling studies using washed platelets stimulated with α-thrombin. In these experiments, the calcium profile was the same as platelets stimulated with PAR1-AP indicating no cross reactivity of BMS-986120 with PAR1.
Pharmacological characterization of BMS-986120 showed specific, saturable and reversible binding to PAR4. In order to facilitate in vivo studies, they determined that it also binds to monkey PAR4 with a high affinity. This is in contrast to other species where there is a weak effect on mouse platelets, and no effect on guinea pig or rat platelet aggregation induced by PAR4-AP. The most striking data in the paper is that BMS-986120 was able to reduce thrombus weight by >80% in the nonhuman primate thrombosis model while at the same concentration (1 mg/kg) it only increased bleeding 2-fold. In contrast, the concentration of clopidogrel that achieved >80% reduction in thrombus weight led to >8-fold increase in bleeding. This demonstrates in a nonhuman primate model a lower bleeding risk and a significantly wider therapeutic window compared to the first line antiplatelet therapy, clopidogrel.
The study from Wong et al. is original and stunning. First, BMS demonstrates a potential for being the first FDA-approved antiplatelet compound specifically targeting PAR4. The compound completed two phase I clinical trials in 2015 and 2016 respectively in UK (NCT02439190) and is currently in phase II clinical trial for thrombosis which will provide more information about its safety and comparison with current standard antiplatelet therapeutics. Second, and more generally, this study highlighted the importance of PAR4 in platelet aggregation and provided a strong proof of targeting PAR4 as a novel clinical antithrombotic strategy. Third, this paper not only highlighted the side effect of current standard antiplatelet therapeutics which is the extension of bleeding time and potential hemorrhage, but also provided three key features to improve the safety clinically. One important feature is reversible binding to the target. Compared to irreversible antagonists, such as clopidogrel and aspirin, the reversible antagonist does not inhibit affected platelets throughout their lifetime and takes less time to be removed from the circulation. Another characteristic that may increase safety is inhibition of PAR4 reactivity and maintaining PAR1 function unaffected. This strategy may preserve initial hemostasis while avoiding uncontrolled thrombus growth, which may be beneficial for as a means to prevent thrombosis with minimized bleeding risk. The last critical feature is the high selectivity to the target receptor. The off-target binding to PAR1 of some compounds that have PAR4 antagonism effect could be deadly since thrombin-induced platelet activation is essential for primary platelet activation. Finally, regardless of the results from the clinical trial, BMS-986120 will be a useful tool for the thrombosis field to expand understanding of PAR4 structure and function. Using vorapaxar to stabilize the conformation of the protein, the crystal structure of vorapaxar/PAR1 was solved in 2012 (25), which provided valuable information about PAR1 overall architecture and ligand binding mechanism. Getting inspired by this, BMS-986120 can also facilitate and promote elucidation of PAR4 structure.
Acknowledgements
Funding: This study was supported by National Institutes of Health Grant R01 HL098217.
Footnote
Conflicts of Interest: The authors have no conflicts of interest to declare.
References
- Wong PC, Seiffert D, Bird JE, et al. Blockade of protease-activated receptor-4 (PAR4) provides robust antithrombotic activity with low bleeding. Sci Transl Med 2017;9:eaaf5294. [Crossref] [PubMed]
- Deng W, Xu Y, Chen W, et al. Platelet clearance via shear-induced unfolding of a membrane mechanoreceptor. Nat Commun 2016;7:12863. [Crossref] [PubMed]
- Zhang W, Deng W, Zhou L, et al. Identification of a juxtamembrane mechanosensitive domain in the platelet mechanosensor glycoprotein Ib-IX complex. Blood 2015;125:562-9. [Crossref] [PubMed]
- Versteeg HH, Heemskerk JW, Levi M, et al. New fundamentals in hemostasis. Physiol Rev 2013;93:327-58. [Crossref] [PubMed]
- Rivera J, Lozano ML, Navarro-Nunez L, et al. Platelet receptors and signaling in the dynamics of thrombus formation. Haematologica 2009;94:700-11. [Crossref] [PubMed]
- Zhao P, Metcalf M, Bunnett NW. Biased signaling of protease-activated receptors. Front Endocrinol (Lausanne) 2014;5:67. [Crossref] [PubMed]
- Patrono C. Aspirin as an antiplatelet drug. N Engl J Med 1994;330:1287-94. [Crossref] [PubMed]
- Nishi T, Tabusa F, Tanaka T, et al. Studies on 2-oxoquinoline derivatives as blood platelet aggregation inhibitors. II. 6-[3-(1-cyclohexyl-5-tetrazolyl)propoxy]-1,2-dihydro-2-oxoquinoline and related compounds. Chem Pharm Bull (Tokyo) 1983;31:1151-7. [Crossref] [PubMed]
- Gresele P, Momi S, Falcinelli E. Anti-platelet therapy: phosphodiesterase inhibitors. Br J Clin Pharmacol 2011;72:634-46. [Crossref] [PubMed]
- Schneider DJ. Anti-platelet therapy: glycoprotein IIb-IIIa antagonists. Br J Clin Pharmacol 2011;72:672-82. [Crossref] [PubMed]
- Dean L. Clopidogrel Therapy and CYP2C19 Genotype. In: Pratt V, McLeod H, Dean L, et al., editors. Medical Genetics Summaries. Bethesda (MD): National Center for Biotechnology Information (US), 2012.
- Scott SA, Sangkuhl K, Stein CM, et al. Clinical Pharmacogenetics Implementation Consortium guidelines for CYP2C19 genotype and clopidogrel therapy: 2013 update. Clin Pharmacol Ther 2013;94:317-23. [Crossref] [PubMed]
- Abdulsattar Y, Ternas T, Garcia D. Vorapaxar: targeting a novel antiplatelet pathway. P T 2011;36:564-8. [PubMed]
- Kahn ML, Nakanishi-Matsui M, Shapiro MJ, et al. Protease-activated receptors 1 and 4 mediate activation of human platelets by thrombin. J Clin Invest 1999;103:879-87. [Crossref] [PubMed]
- Coughlin SR. Thrombin signalling and protease-activated receptors. Nature 2000;407:258-64. [Crossref] [PubMed]
- Holinstat M, Voss B, Bilodeau ML, et al. PAR4, but not PAR1, signals human platelet aggregation via Ca2+ mobilization and synergistic P2Y12 receptor activation. J Biol Chem 2006;281:26665-74. [Crossref] [PubMed]
- Xu WF, Andersen H, Whitmore TE, et al. Cloning and characterization of human protease-activated receptor 4. Proc Natl Acad Sci U S A 1998;95:6642-6. [Crossref] [PubMed]
- Sidhu TS, French SL, Hamilton JR. Differential signaling by protease-activated receptors: implications for therapeutic targeting. Int J Mol Sci 2014;15:6169-83. [Crossref] [PubMed]
- Duvernay M, Young S, Gailani D, et al. Protease-activated receptor (PAR) 1 and PAR4 differentially regulate factor V expression from human platelets. Mol Pharmacol 2013;83:781-92. [Crossref] [PubMed]
- Wu CC, Huang SW, Hwang TL, et al. YD-3, a novel inhibitor of protease-induced platelet activation. Br J Pharmacol 2000;130:1289-96. [Crossref] [PubMed]
- Wu CC, Hwang TL, Liao CH, et al. Selective inhibition of protease-activated receptor 4-dependent platelet activation by YD-3. Thromb Haemost 2002;87:1026-33. [PubMed]
- Young SE, Duvernay MT, Schulte ML, et al. A Novel and Selective PAR4 Antagonist: ML354. Probe Reports from the NIH Molecular Libraries Program. Bethesda (MD): National Center for Biotechnology Information (US), 2010.
- Young SE, Duvernay MT, Schulte ML, et al. Synthesis of indole derived protease-activated receptor 4 antagonists and characterization in human platelets. PLoS One 2013;8:e65528. [Crossref] [PubMed]
- Temple KJ, Duvernay MT, Maeng JG, et al. Identification of the minimum PAR4 inhibitor pharmacophore and optimization of a series of 2-methoxy-6-arylimidazo[2,1-b][1,3,4]thiadiazoles. Bioorg Med Chem Lett 2016;26:5481-6. [Crossref] [PubMed]
- Zhang C, Srinivasan Y, Arlow DH, et al. High-resolution crystal structure of human protease-activated receptor 1. Nature 2012;492:387-92. [Crossref] [PubMed]