Molecular mechanisms of mechanotransduction in psoriasis
Introduction
Psoriasis represents a skin disease which is characterized by chronic inflammation with a severe physical and psychological burden (1). In fact, the psychological burden is compared measurably to other chronic diseases that are threatening for the patients’ life, such as cancer, depression, myocardial infarction and congestive heart failure (2). While psoriasis has been recognized as a distinct clinical entity from the 19th century, nowadays it still has a major impact on the patients’ quality of life (1). It affects approximately 2–4% of the population with various differences depending on age, gender and geographic location (3). Adults present more often with the disease compared to children, whereas countries that are more distant from the equator exhibit higher prevalence compared to the ones closer to it, which benefit from the stronger ultraviolet (UV) radiation (4,5). Prevalence is generally equal in the western countries and it seems that females have a higher incidence when they are <18 years old and adult males have a higher incidence compared to adult females (6,7).
Patients that suffer from psoriasis complain for itch, bleeding and pain (2). There are five distinct clinical types of psoriasis with different histological features that have been described: plaque-type (psoriasis vulgaris) that represents the most common type of the diseases with an incidence of 90%, guttate (droplet) psoriasis, inverse psoriasis, pustular psoriasis that is characterized by blisters, and erythrodermic psoriasis (2). Psoriasis affects the whole skin, however it seems that it has a preference for the extensor surfaces of forearms and shin, peri-anal, peri-umbilical, nails and the scalp (2). Psoriasis is also accompanied by other comorbid diseases such as psoriatic arthritis, which develops in approximately 20–30% of the patients suffering from psoriasis, metabolic syndrome, cardiovascular diseases, diabetes, cancer and depression (1).
Experimental studies regarding the molecular pathology, molecular genetics and epigenetics of psoriasis have revealed distinct molecular features that govern the onset and the development of the disease (8-10). The engagement of individual molecules has enriched the treatment strategies regarding targeted treatment against psoriasis (11,12). However, many of the aspects of the molecular pathology of keratinocytes and the underpinning mechanisms of the events that trigger psoriasis remain elusive. In this context, a number of studies highlight the impact of mechanical forces and mechanotransduction during triggering events of the disease, therefore inducing mechano-regulated signaling pathways in keratinocytes (13-16). In the present review we discuss the impact of the application of mechanical/physical forces on keratinocytes and molecular mechanisms of mechanotransduction that promote skin pathogenesis and psoriasis, as well as putative therapeutic strategies that can be generated from this specific aspect.
Molecular pathogenesis of psoriasis
Immunopathology
The onset of the illness represents an immune response and it is distinguished in three phases: the sensitization phase, the resting phase, and finally the active phase. At the onset of psoriasis, immature dendritic cells, which are found in tissues, recognize and ingest exogenous antigens, and finally migrate to the secondary lymphoid organs in order to treat and present the antigen to T-cells (17). Following antigen uptake, dendritic cells undergo a maturation process to cope with the inflammatory conditions, largely due to cytokines such as tumor necrosis factor-α (TNF-α) and interleukin-1β (IL-1β), or to deal with microbial products that excite microbial cells through specific receptors (18).
At the active phase, when psoriatic plaques are formed, the first visible events are surface vascular infiltration of lymphocytes and monocytes and the distension of blood vessels in dermal papillae (19). The passage of immune cells through the endothelial wall of the vessels is called dialysis and is probably performed through pores formed between endothelial cells. This process seems to be dependent on the integrins (19). Immune cells can be activated after they migrate to the skin. In dermis and epidermis various groups of antigen presenting cells can stimulate T cells and vice versa. T cells that have migrated to the epidermis may additionally be activated by keratinocytes or also proliferate as a consequence of activation on the skin (20).
According to specific studies that have associated the expression of T-cell cytokines, IL-22 and IL-17 cytokines exhibit the highest expression in altered psoriatic epidermis (21). IL-22 has also been found in the blood of patients suffering from psoriasis and its concentration correlates to the severity of the disease (22). IL-22 is produced by activated T-cells and natural killer T (NKT)-cells, but not by other immune cells or tissue cells. The primary source of IL-22 in psoriatic skin is the T-helper 17 (Th17) and Th1 cells (22). Recently, increased IL-17 expression in epidermal psoriatic lesions has also been demonstrated (23). Furthermore, it is also known that in psoriatic skin lesions the number of macrophages is increased and that the amount of NKT-cells is also increased compared to normal skin (24).
Immune cells can be activated following their migration to the skin. In dermis and epidermis various groups of antigen-presenting cells can stimulate T-cells and vice versa. T-cells that have migrated to the epidermis may additionally be activated by keratinocytes or also proliferate as a consequence of their activation in the skin (25). In the epidermis, migrated T-cells can also be activated by keratinocytes. Keratinocytes appear to express molecules of the major histocompatibility complex (MHC) class II and CD54 (cluster of differentiation 54) after exposure to interferon-γ (INF-γ), thus promoting antigen presentation and invitation of T-cells (26). Activated keratinocytes are also able to promote T-cell proliferation from bacterially-derived superantigens (27).
Aberrant function of keratinocytes in psoriasis
Keratinocytes appear to play a role in the activation of NKT-cells through the CD1d molecule. In psoriatic skin, CD1d is overexpressed, and it can also be rapidly induced in healthy skin in cases of trauma that stops the barrier function. In in vitro experiments, after exposure to IFN-γ, keratinocytes showed an increased expression of CD1d. By combining CD1d+ keratinocytes with clones of human NKT-cells, they were activated to produce large amounts of INF-γ (28). T-cells, NKT-cells and NK cells produce amounts of IFN-γ, which appear to activate the monocytes/macrophages and dendritic cells that are filtered into the skin. Activation may also include heat-shock proteins produced by keratinocytes. Cutaneous macrophages in altered dermis may be the major source of TNF-α production (28).
Keratinocytes can also be activated during the onset of psoriatic lesions mainly from mediators produced by Th1 cells (IFN-γ and IL-22) (29). However, many other mediators have been found who may play a role. Activation of keratinocytes leads first to the increasing of their proliferation and to the modification of their maturation. In addition, activated keratinocytes produce numerous and diverse mediators that can cause further migration of the immune system cells, activation of the stromal cells of the dermis and promotion of angiogenesis. Proliferation of the keratinocytes in psoriatic lesions presents an approximately 50-fold increase (30). The cells of the immune system may in part be responsible for their proliferation. It has been found that activated and non-activated T-cells release agents that can increase the proliferation of keratinocytes (31). Of the T-cells mediators that have been studied, there are various, such as IFN-γ and tumor growth factor-β (TGF-β), that appear to inhibit keratinocyte proliferation, while others do not affect or increase their proliferation at high concentrations (32).
The impressive therapeutic effects of TNF-α inhibition in psoriasis make us assume that this cytokine increases keratinocyte proliferation strongly. The macrophages in papillary dermis may be the major source of TNF-α in psoriatic skin (33). Accordingly, macrophages seem to be the main responsible cell type for psoriasis skin lesions. TNF-α promotes the production of TGF-α factor in keratinocytes (34). TGF-α mRNA and the corresponding protein are found with increased expression in psoriatic skin than in seemingly healthy skin (35). TGF-α binds to the epidermal growth factor receptor (EGFR) and stimulates the proliferation of keratinocytes, but also accelerates skin regeneration (36). In addition to TGF-α, there are other factors that are produced by keratinocytes and play a role in their proliferation [e.g., the nerve growth factor (NGF)].
In normal skin, maturation of keratinocytes from the base layer to the keratinized layer lasts about 28 days. The final stage of the maturation process is the final differentiation, an apoptotic stage resulting in the creation of a mechanically resistant keratinized layer (37). In addition to IL-22, IL-20 appears to have similar effects because it acts through a similar receptor. Interestingly, TNF-α increases keratinocyte susceptibility to IL-20 (38). In general, IL-20 shows increased expression levels in psoriatic skin, and polymorphisms of a nucleotide in the IL-20 gene are associated with psoriasis (39). IL-22 and IL-20 activate core proliferating and differentiating pathways such as the signal transducers and activators of transcription-3 (STAT-3) pathway in keratinocytes (38).
Mechanotransduction in keratinocytes and psoriasis
Among factors that have been implicated for triggering psoriasis, the application of mechanical stress features prominently. A typical example, widely known as Koebner phenomenon, involves the development of psoriatic plaques in apparently healthy skin following trauma and/or mechanical stress (scratches, abrasion, pressure from tight shoes, shaving, etc.) (13,15).
Physical interactions of keratinocytes and modulation of their properties by mechanical forces is regulated by mechanotransduction, this means the process by which cells sense and convert mechanical cues into intracellular biochemical signals in order to evoke a cellular response. Mechanotransduction has been involved in the regulation of proliferation, differentiation, morphology and migration of keratinocytes, ultimately preserving cellular homeostasis (16). The Koebner effect as a phenomenon has been described in many diseases. According to literature, it appears that in an average of 25% of patients with psoriasis, they will develop psoriatic plaques in apparently healthy tissue after injury, but the trauma may not be recognized or be forgotten (40). The localization of psoriatic plaques can be tested experimentally using specific activating agents to cause psoriatic plaques in subjects with skin sensitivity. These triggering factors do not cause psoriasis but are factors or events that determine the localization of psoriatic plaques (41). Factors that have been implicated to trigger the phenomenon are friction, abrasion, pressure from narrow shoes, shaving, finger sucking, and so on (42). The Koebner effect can be induced in a prone person with a variety of experimental techniques, all of which mainly cause epidermal cell injury and skin inflammation (43).
Mechano-induced signaling pathways in psoriasis
Cells interact with the extracellular matrix and their environment and respond to various stimuli. Air, fluid movement and mechanical pressure are some factors that cells are called upon to recognize and react (Figure 1). Mechanotransduction regulates the function of cells, as it is able to control their proliferation, cytoskeleton structure and broader gene expression.
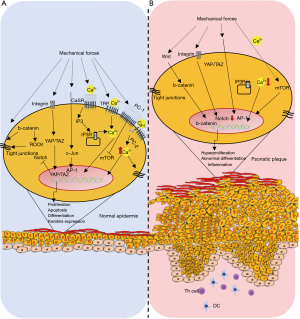
On the epidermis, mechanical stimulation plays a very important role as epidermis is the first tissue that contacts directly with the strongest mechanical forces. Epidermal keratinocytes have developed protective mechanisms to maintain their structure and function after mechanical stimulation. It has been shown that weakness of keratinocyte responsiveness to mechanical stimuli can lead to clamping of adhesive structure (44), resulting in the entry of immune cells into upper layers of the epidermis and their wider aberrant signaling, leading to pathological phenotypes such as psoriasis (45).
Wnt pathway
Catenins are cytoplasmic proteins and can potentially work as transcriptional factors. They also play an important role in the maintenance of normal tissue architecture (46) as cell-to-cell adhesion proteins and by binding to the cytoskeleton and maintain intracellular adhesiveness (47). Beta-catenin (β-catenin) is of central importance in regulating cellular signal transduction through the Wnt pathway and is a crucial mediator of skin development (48). Recent findings suggest that β-catenin regulates self-renewal of interfollicular epidermal stem cells via the Wnt pathway (49). B-catenin seems to mediate mechanotransduction through Rho-associated protein kinase (ROCK) activation that generates collagen production, hyperproliferation of keratinocytes and epidermal stiffness (Figure 1) (50). It has been demonstrated in vivo that β-catenin protects the epidermis from mechanical stresses by supporting the tight junctions as cell adhesion molecules (51). Several studies which investigated the role of β-catenin in the inhibition of epithelization and wound healing claim that no nuclear β-catenin was detected in normal epidermis (52). Furthermore, it was found that β-catenin was detected only in the cell membrane of the normal epidermis, and not in nucleus (53).
In psoriatic tissue there has been detected increased nuclear β-catenin in suprabasal lesional epidermis (54). The enhanced distribution of β-catenin in the membrane of normal cells compared to the diminished nuclear localization may be due to its role in the establishment and regulation of adherens junctions by interaction with E-cadherin through alpha (α)-catenin with the actin cytoskeleton. Furthermore, it has also been suggested that β-catenin may be translocated from the nucleus back to the cell membrane, presumably through a soluble, cytosolic intermediate state (55).
Yes-associated protein (YAP)/TAZ coactivators
The YAP family and its transcriptional coactivator with PDZ-binding motif (TAZ) function as skin size, tissue overgrowth and stem cell proliferation mediators (56). YAP/TAZ are mechanotransducers and effectors of the Hippo signalling pathway (57). At the canonical Hippo pathway, YAP/TAZ is regulated by several extracellular stimuli including the G-protein-coupled receptors (GPCRs), Wnt signaling, and mechanical forces. They also interact with a plethora of factors such as α-catenin to regulate cellular activities (58).
YAP is activated and transferred to the nucleus during wound healing of the skin in order to activate the differentiation of stem cells by promoting epidermal proliferation and inhibiting terminal differentiation (Figure 1) (59-61). In vitro models of keratinocytes suggest that mechanical forces act via integrin adhesions and the actin cytoskeleton to induce the nuclear translocation of YAP/TAZ co-activators in order to upregulate cell proliferation (Figure 1) (62). A remaining suprabasal integrin expression alongside with expression of terminal, epidermal differentiation markers has been observed in psoriatic lesions (63). Thus, the hyperproliferation of keratinocytes and the failure to downregulate integrin expression during initiation of terminal differentiation can prevent YAP from its translocation into the nucleus and inhibit terminal proliferation. Furthermore, YAP/TAZ have been found inactivated in cells sensing low mechanical forces, in order to orchestrate the loss of stemness and differentiation. This problem is overlapped by Notch signalling, which is a fundamental negative regulator of epidermal stemness and an inducer of terminal differentiation (64). In epidermal progenitors and inside an environment of high tension, YAP/TAZ interact with Notch activators and inhibit them (65). In conclusion, we can assume that continuous increased mechanical stimuli can induce YAP/TAZ to inhibit Notch signaling and promote abnormal phenotype in psoriatic skin (Figure 1).
Activator protein-1 (AP-1)
The transcription factor AP-1 participates in the regulation of crucial cellular processes, including proliferation, apoptosis, differentiation, survival and migration (66). Extracellular stimuli, such as mechanical forces, can activate AP-1 and mediate gene expression (Figure 1) (67). The AP-1 family consists of dimeric proteins, which are members of Jun, Fos, and activating transcription factor (ATF) protein families. The AP-1 complex binds to the promoters of specific genes in specific sequences and transactivates or represses them, therefore it has been recognized as regulator of carcinogenesis and inflammation (51,68).
In skin, AP-1 is expressed in keratinocytes and regulates proliferation and differentiation of the epidermis. AP-1 binds to specific DNA-binding sites and induces transcription of keratins, which are important proteins for terminal keratinocyte differentiation (Figure 1) (69). Since mechanotransduction is a modulator of skin gene expression, previous research studies showed that AP-1 was upregulated in mechanically stretched skin (70). In psoriasis, Fos/Jun and Jun/AP-1 proteins are downregulated and this leads to increased cell proliferation and deregulated cytokine expression (71). Since mechanical stimuli can activate AP-1 proteins, impaired cell mechanosensing can induce AP-1 inactivation, followed by aberrant inflammation and proliferation status that characterizes the psoriatic plaques (Figure 1).
Mechanosensitive receptors and ion channels
It has been reported that applied mechanical forces cause calcium waves in keratinocytes (72). Calcium homeostasis plays important roles in various aspects of epidermal physiology. Maintenance of an intracellular calcium concentration in strictly specific levels is strongly associated with the epidermal barrier homeostasis and differentiation (73). Extracellular calcium is a crucial mediator of differentiation and proliferation of keratinocytes. Mechanical stimuli can induce calcium signaling through various receptors and second messengers. Mechanosensitive ion channels are distributed across the plasma membrane of keratinocytes and sense the changes of calcium followed by the proper signalling (74). Signal transducers, such as GPCRs and ion channels, are the main operators of mechanical-stress-induced calcium signaling.
Calcium sensing receptor (CaSR)
CaSR belongs to the GPCR protein family. The expression of the epidermal CaSR gene takes place in the suprabasal keratinocyte layers (75). When extracellular calcium gradient is elevated, calcium ions bind to the extracellular domain of CaSR, which allows this protein to monitor and regulate the concentration of calcium in the blood (76). Via G-protein signaling, the inositol triphosphate (IP3) receptor is generated and binds in the endoplasmic reticulum (ER). IP3 binds to its receptor and causes depletion of calcium internal stores. As a result, concentration of calcium increases in the cytoplasm and through second messages affects gene expression (Figure 1) (77).
Studies in transgenic knock-out mouse models for the CaSR gene in keratinocytes showed reduced terminal differentiation (78). Regarding the role of the CaSR on the Wnt/β-catenin signaling pathway, which plays an important role in the regulation of keratinocytes, high extracellular calcium leads to the differentiation of keratinocytes via the activation of the CaSR, which depends on Wnt pathway effectors (79). Defective CaSR can probably lead to defective Wnt signaling and acquisition of psoriatic characteristics in the skin (Figure 1).
Apart from its role in calcium signaling, CaSR modulates E-cadherin-mediated cell adhesion in keratinocytes (80). As a result, catenins activate and form the E-cadherin/catenin complex followed by PI3K-dependent activation of downstream calcium effectors, PLC-1 (phospholipase C), IP3 (Inositol-3,4,5-trisphosphate), DAG (diacylglycerol) and STIM (stromal interaction molecules) (81). In psoriatic plaques, cell junctions are altered (82). Aberrant CaSR function may destroy tight junctions and the barriers that they construct and allow inflammatory cells to enter the keratinocyte environment.
Transient receptor potential (TRP) channels
TRP channels constitute a protein family of non-selective ion channels, which display great diversity in activation mechanisms and selectivities. They are activated by a variety of different stimuli and function as signal integrators (83). TRP channels are crucial for cell survival and this explains why there are so many homologs of them in different mammalian species. They are categorized according to sequence similarity into seven groups: TRPC (canonical), TRPV (vanilloid), TRPM (melastatin), TRPN, TRPA (ankyrin), TRPP (polycystins), TRPML (mucolipin) (84).
The main role of TRP channels in the skin is to respond to mechanical stimuli and induce calcium signaling through activation of fundamental calcium signaling elements. Therefore it regulates cellular homeostasis, vesicles formation, enzymatic activity and gene expression (84). As a result, TRP channels play a critical role in cellular homeostasis and growth control, but also in the regulation of keratinocyte fate and survival, immune, and inflammatory mechanisms in the epidermis (Figure 1) (85).
TRPVs, TRPA1 and TRPCs are the main subgroups that are expressed in epidermal keratinocytes. TRPV1, TRPV3, TRPV4, TRPV6 belong to the subfamily of TRPVs. TRPV1 is activated and promotes calcium-dependent inhibition of cell proliferation and increases apoptosis (86). Activation of TRPV1 can also lead to expression of proinflammatory cytokine genes and protect the epidermis from inflammation (87). TRPV3 is involved with EGFR signalling pathway and TRPV4 is functionally co-expressed with junctional proteins (e.g., β-catenin and E-cadherin), thus forming the proper epidermal barrier (88,89). TRPV4 and TRPV6 orchestrate the terminal differentiation process of keratinocytes by modulating the expression of keratins and involucrin (90). TRPA1 is a member of the TRPA subfamily in the epidermis. TRPA1 can induce the expression of tight junction proteins in mechanically disrupted epidermis and regenerate epidermal barrier (91).
TRPC1, TRPC4, TRPC5, TRPC6 and TRPC7 are key representatives of TRP channels in epidermal keratinocytes (92). TRPC1, TRPC4, TRPC6 mediate calcium influx and induce terminal differentiation of keratinocytes (93). TRPC4 can, also, stimulate via DAG and enhance calcium entry to the cytoplasm (94). Impaired function of TRCs induces keratinocyte proliferation and increases terminal differentiation. Accumulating evidence suggests that TRPC channels show suppressed expression levels in psoriatic plaques (Figure 1) (95).
Polycystins as putative mechano-induced molecules in keratinocytes
Polycystins constitute a subfamily of proteins that have emerged as chief mechanosensors in epithelial cells and. The main representatives of this family are polycystin-1 (PC1) and polycystin-2 (PC2). PC1 is a transmembrane protein with a large, pliable, mechanosensitive N-extracellular end, and a C-terminus that produces transcriptionally active cleavages. PC2 constitutes a non-selective cation channel permeable for Ca2+ ions, which belongs to the family of TRP (TRPP) channels (96). Studies generated from our research group revealed that polycystins participate as transcriptional regulators of the expression of osteoblastic genes upon mechanical stimulation, but also during pathogenic mechanisms of different clinical entities such as colorectal cancer and atheromatosis (97-100). It was conceivable to hypothesize that since polycystins regulate mechanotransduction and calcium homeostasis, which in turn have been incriminated in psoriasis pathogenesis, polycystins could get involved in molecular mechanisms responsible for the development of the disease.
Our preliminary data show that keratinocytes demonstrate basic levels of endogenous expression of PC1 and PC2 and that PC1 seems to be downregulated in psoriatic lesions. Advancing further in elucidating a potential molecular mechanism through which PC1 downregulation could be a part of the development of psoriatic plaques, the mammalian target of rapamycin (mTOR) pathway emerges as a powerful link that could mediate PC1 impact on psoriasis pathogenesis. The PI3K/Akt/mTOR pathway has been associated with immunopathogenic mechanisms, hyperplasia of the epidermis and angiogenesis (101). mTOR and its downstream substrates have been found activated in psoriatic lesions and mTOR inhibition attenuates cell proliferation in keratinocytes (102,103). Given our emerging findings showing PC1 downregulation in psoriatic lesions, a potential mechanism that associates PC1 downregulation with mTOR activation in psoriatic lesions and keratinocytes in vitro seems appealing in order to be explored (Figure 1).
Putative treatment strategies against psoriasis
Current treatments include conventional therapies with adverse effects and a short-term remission of symptoms, but also targeted therapy with monoclonal antibodies and a receptor fusion protein against TNF-α, inhibitors against IL-12/IL-23p40, IL-17, IL-22, IL-23, IL-36, IL-1, type I IFN-γ and small molecules against Janus kinase (JAK) and phosphodiesterase-4 (PDE-4), which show promising results (2,11). Given this fact, targeted therapy seems to be the answer and an area where research is employed to cover unmet needs regarding psoriasis treatment. In this context, mechanobiology and the exploitation of mechanical forces emerge as a novel field that it could be very useful in order to reveal new predictive biomarkers that will assess individual response to treatment and help tailor individual treatment protocols, but also novel molecular targets in order to develop new agents.
Mechanosensing is being thoroughly investigated in carcinogenesis as a field that regulates interactions between cancer cells, extracellular matrix and stromal cells (104). Aberrant tumor mechanosensing has been strongly associated with resistance to applied treatments (104,105). Such mechanisms are mediated through activation of YAP/TAZ coactivators, which were described previously to be also activated in mechano-induced events in keratinocytes (104,105). Therefore, such mechanisms could be investigated in psoriasis as potential mediators of treatment resistance, but also as novel therapeutic targets. Investigation of mechanotransduction and its impact on the development of the psoriatic plaques may also give rise to novel molecules that may serve as predictive biomarkers or new pharmaceutical targets. In this context, polycystins have been already associated with cancer progression and it would be interesting to decipher their role in keratinocyte aberrant proliferation and psoriasis.
Conclusions
The development of psoriasis upon triggering events such as the Koebner phenomenon implies a decisive impact of mechanical cues on the onset of the disease. However, distorted function of mechanosensitive molecules potentially creates a vicious circle during the development of the disease. The field of mechanobiology and mechanotransduction offers novel routes in order to decipher the molecular mechanisms that accompany the formation of psoriatic lesions, but also reveal signalling networks with diagnostic and therapeutic potential.
Acknowledgements
None.
Footnote
Conflicts of Interest: The authors have no conflicts of interest to declare.
References
- Di Meglio P, Villanova F, Nestle FO. Psoriasis. Cold Spring Harb Perspect Med 2014;4. [Crossref] [PubMed]
- Boehncke WH, Schon MP. Psoriasis. Lancet 2015;386:983-94. [Crossref] [PubMed]
- Chandran V, Raychaudhuri SP. Geoepidemiology and environmental factors of psoriasis and psoriatic arthritis. J Autoimmun 2010;34:J314-21. [Crossref] [PubMed]
- Hart PH, Gorman S, Finlay-Jones JJ. Modulation of the immune system by UV radiation: more than just the effects of vitamin D? Nat Rev Immunol 2011;11:584-96. [Crossref] [PubMed]
- Parisi R, Symmons DP, Griffiths CE, et al. Global epidemiology of psoriasis: a systematic review of incidence and prevalence. J Invest Dermatol 2013;133:377-85. [Crossref] [PubMed]
- Icen M, Crowson CS, McEvoy MT, et al. Trends in incidence of adult-onset psoriasis over three decades: a population-based study. J Am Acad Dermatol 2009;60:394-401. [Crossref] [PubMed]
- Tollefson MM, Crowson CS, McEvoy MT, et al. Incidence of psoriasis in children: a population-based study. J Am Acad Dermatol 2010;62:979-87. [Crossref] [PubMed]
- Mahil SK, Capon F, Barker JN. Genetics of psoriasis. Dermatol Clin 2015;33:1-11. [Crossref] [PubMed]
- Pollock RA, Abji F, Gladman DD. Epigenetics of psoriatic disease: A systematic review and critical appraisal. J Autoimmun 2017;78:29-38. [Crossref] [PubMed]
- Woo YR, Cho DH, Park HJ. Molecular Mechanisms and Management of a Cutaneous Inflammatory Disorder: Psoriasis. Int J Mol Sci 2017;18. [Crossref] [PubMed]
- Conrad C, Gilliet M. Psoriasis: from Pathogenesis to Targeted Therapies. Clin Rev Allergy Immunol 2018;54:102-13. [Crossref] [PubMed]
- Tan WS, Kelly S, Pitzalis C. Targeted therapies: what they teach us about the pathogenesis of psoriasis and psoriatic arthritis. Expert Rev Clin Immunol 2017;13:207-22. [Crossref] [PubMed]
- Arias-Santiago S, Espineira-Carmona MJ, Aneiros-Fernandez J. The Koebner phenomenon: psoriasis in tattoos. CMAJ 2013;185:585. [Crossref] [PubMed]
- Blaydon DC, Kelsell DP. Defective channels lead to an impaired skin barrier. J Cell Sci 2014;127:4343-50. [Crossref] [PubMed]
- Sagi L, Trau H. The Koebner phenomenon. Clin Dermatol 2011;29:231-6. [Crossref] [PubMed]
- Wong VW, Akaishi S, Longaker MT, et al. Pushing back: wound mechanotransduction in repair and regeneration. J Invest Dermatol 2011;131:2186-96. [Crossref] [PubMed]
- Nickoloff BJ, Qin JZ, Nestle FO. Immunopathogenesis of psoriasis. Clin Rev Allergy Immunol 2007;33:45-56. [Crossref] [PubMed]
- De Smedt T, Pajak B, Muraille E, et al. Regulation of dendritic cell numbers and maturation by lipopolysaccharide in vivo. J Exp Med 1996;184:1413-24. [Crossref] [PubMed]
- Cai Y, Fleming C, Yan J. New insights of T cells in the pathogenesis of psoriasis. Cell Mol Immunol 2012;9:302-9. [Crossref] [PubMed]
- Chiricozzi A, Romanelli P, Volpe E, et al. Scanning the Immunopathogenesis of Psoriasis. Int J Mol Sci 2018;19. [Crossref] [PubMed]
- Kunz S, Wolk K, Witte E, et al. Interleukin (IL)-19, IL-20 and IL-24 are produced by and act on keratinocytes and are distinct from classical ILs. Exp Dermatol 2006;15:991-1004. [Crossref] [PubMed]
- Wolk K, Kunz S, Witte E, et al. IL-22 increases the innate immunity of tissues. Immunity 2004;21:241-54. [Crossref] [PubMed]
- Chan JR, Blumenschein W, Murphy E, et al. IL-23 stimulates epidermal hyperplasia via TNF and IL-20R2-dependent mechanisms with implications for psoriasis pathogenesis. J Exp Med 2006;203:2577-87. [Crossref] [PubMed]
- Cameron AL, Kirby B, Fei W, et al. Natural killer and natural killer-T cells in psoriasis. Arch Dermatol Res 2002;294:363-9. [Crossref] [PubMed]
- Boyman O, Hefti HP, Conrad C, et al. Spontaneous development of psoriasis in a new animal model shows an essential role for resident T cells and tumor necrosis factor-alpha. J Exp Med 2004;199:731-6. [Crossref] [PubMed]
- Griffiths CE, Voorhees JJ, Nickoloff BJ. Gamma interferon induces different keratinocyte cellular patterns of expression of HLA-DR and DQ and intercellular adhesion molecule-I (ICAM-I) antigens. Br J Dermatol 1989;120:1-8. [Crossref] [PubMed]
- Nickoloff BJ, Mitra RS, Green J, et al. Accessory cell function of keratinocytes for superantigens. Dependence on lymphocyte function-associated antigen-1/intercellular adhesion molecule-1 interaction. J Immunol 1993;150:2148-59. [PubMed]
- Bonish B, Jullien D, Dutronc Y, et al. Overexpression of CD1d by keratinocytes in psoriasis and CD1d-dependent IFN-gamma production by NK-T cells. J Immunol 2000;165:4076-85. [Crossref] [PubMed]
- Baliwag J, Barnes DH, Johnston A. Cytokines in psoriasis. Cytokine 2015;73:342-50. [Crossref] [PubMed]
- Ogawa E, Sato Y, Minagawa A, et al. Pathogenesis of psoriasis and development of treatment. J Dermatol 2018;45:264-72. [Crossref] [PubMed]
- Nedoszytko B, Lange M, Sokolowska-Wojdylo M, et al. The role of regulatory T cells and genes involved in their differentiation in pathogenesis of selected inflammatory and neoplastic skin diseases. Part II: The Treg role in skin diseases pathogenesis. Postepy Dermatol Alergol 2017;34:405-17. [Crossref] [PubMed]
- Sferra R, Fargnoli MC, Corbelli E, et al. Immunopathogenesis of psoriasis: a possible role of TGFbeta/Smads pathway. Ital J Anat Embryol 2014;119:277-85. [PubMed]
- Nickoloff BJ, Karabin GD, Barker JN, et al. Cellular localization of interleukin-8 and its inducer, tumor necrosis factor-alpha in psoriasis. Am J Pathol 1991;138:129-40. [PubMed]
- Valyi-Nagy I, Jensen PJ, Albelda SM, et al. Cytokine-induced expression of transforming growth factor-alpha and the epidermal growth factor receptor in neonatal skin explants. J Invest Dermatol 1992;99:350-6. [Crossref] [PubMed]
- Elder JT, Fisher GJ, Lindquist PB, et al. Overexpression of transforming growth factor alpha in psoriatic epidermis. Science 1989;243:811-4. [Crossref] [PubMed]
- Schultz GS, White M, Mitchell R, et al. Epithelial wound healing enhanced by transforming growth factor-alpha and vaccinia growth factor. Science 1987;235:350-2. [Crossref] [PubMed]
- Candi E, Schmidt R, Melino G. The cornified envelope: a model of cell death in the skin. Nat Rev Mol Cell Biol 2005;6:328-40. [Crossref] [PubMed]
- Blumberg H, Conklin D, Xu WF, et al. Interleukin 20: discovery, receptor identification, and role in epidermal function. Cell 2001;104:9-19. [Crossref] [PubMed]
- Koks S, Kingo K, Ratsep R, et al. Combined haplotype analysis of the interleukin-19 and -20 genes: relationship to plaque-type psoriasis. Genes Immun 2004;5:662-7. [Crossref] [PubMed]
- Boyd AS, Neldner KH. The isomorphic response of Koebner. Int J Dermatol 1990;29:401-10. [Crossref] [PubMed]
- Miller RA. The Koebner phenomenon. Int J Dermatol 1982;21:192-7. [Crossref] [PubMed]
- Farber EM, Roth RJ, Aschheim E, et al. Role of Trauma in Isomorphic Response in Psoriasis. Arch Dermatol 1965;91:246-51. [Crossref] [PubMed]
- Yamamoto T. Psoriatic arthritis: from a dermatological perspective. Eur J Dermatol 2011;21:660-6. [PubMed]
- Seetharaman S, Etienne-Manneville S. Integrin diversity brings specificity in mechanotransduction. Biol Cell 2018;110:49-64. [Crossref] [PubMed]
- Aubin F. What's new in dermatological research? Ann Dermatol Venereol 2011;138 Suppl 4:S233-40. [Crossref] [PubMed]
- Hardman MJ, Liu K, Avilion AA, et al. Desmosomal cadherin misexpression alters beta-catenin stability and epidermal differentiation. Mol Cell Biol 2005;25:969-78. [Crossref] [PubMed]
- Shirakata Y. Regulation of epidermal keratinocytes by growth factors. J Dermatol Sci 2010;59:73-80. [Crossref] [PubMed]
- Lim X, Nusse R. Wnt signaling in skin development, homeostasis, and disease. Cold Spring Harb Perspect Biol 2013;5. [Crossref] [PubMed]
- Lim X, Tan SH, Koh WL, et al. Interfollicular epidermal stem cells self-renew via autocrine Wnt signaling. Science 2013;342:1226-30. [Crossref] [PubMed]
- Samuel MS, Lopez JI, McGhee EJ, et al. Actomyosin-mediated cellular tension drives increased tissue stiffness and beta-catenin activation to induce epidermal hyperplasia and tumor growth. Cancer Cell 2011;19:776-91. [Crossref] [PubMed]
- Ray S, Foote HP, Lechler T. beta-Catenin protects the epidermis from mechanical stresses. J Cell Biol 2013;202:45-52. [Crossref] [PubMed]
- Stojadinovic O, Brem H, Vouthounis C, et al. Molecular pathogenesis of chronic wounds: the role of beta-catenin and c-myc in the inhibition of epithelialization and wound healing. Am J Pathol 2005;167:59-69. [Crossref] [PubMed]
- Butz S, Larue L. Expression of catenins during mouse embryonic development and in adult tissues. Cell Adhes Commun 1995;3:337-52. [Crossref] [PubMed]
- Hampton PJ, Ross OK, Reynolds NJ. Increased nuclear beta-catenin in suprabasal involved psoriatic epidermis. Br J Dermatol 2007;157:1168-77. [Crossref] [PubMed]
- Armstrong DD, Wong VL, Esser KA. Expression of beta-catenin is necessary for physiological growth of adult skeletal muscle. Am J Physiol Cell Physiol 2006;291:C185-8. [Crossref] [PubMed]
- Camargo FD, Gokhale S, Johnnidis JB, et al. YAP1 increases organ size and expands undifferentiated progenitor cells. Curr Biol 2007;17:2054-60. [Crossref] [PubMed]
- Dupont S, Morsut L, Aragona M, et al. Role of YAP/TAZ in mechanotransduction. Nature 2011;474:179-83. [Crossref] [PubMed]
- Schlegelmilch K, Mohseni M, Kirak O, et al. Yap1 acts downstream of alpha-catenin to control epidermal proliferation. Cell 2011;144:782-95. [Crossref] [PubMed]
- Beverdam A, Claxton C, Zhang X, et al. Yap controls stem/progenitor cell proliferation in the mouse postnatal epidermis. J Invest Dermatol 2013;133:1497-505. [Crossref] [PubMed]
- Lee MJ, Byun MR, Furutani-Seiki M, et al. YAP and TAZ regulate skin wound healing. J Invest Dermatol 2014;134:518-25. [Crossref] [PubMed]
- Zhang H, Pasolli HA, Fuchs E. Yes-associated protein (YAP) transcriptional coactivator functions in balancing growth and differentiation in skin. Proc Natl Acad Sci U S A 2011;108:2270-5. [Crossref] [PubMed]
- Fan R, Kim NG, Gumbiner BM. Regulation of Hippo pathway by mitogenic growth factors via phosphoinositide 3-kinase and phosphoinositide-dependent kinase-1. Proc Natl Acad Sci U S A 2013;110:2569-74. [Crossref] [PubMed]
- Hertle MD, Kubler MD, Leigh IM, et al. Aberrant integrin expression during epidermal wound healing and in psoriatic epidermis. J Clin Invest 1992;89:1892-901. [Crossref] [PubMed]
- Blanpain C, Lowry WE, Pasolli HA, et al. Canonical notch signaling functions as a commitment switch in the epidermal lineage. Genes Dev 2006;20:3022-35. [Crossref] [PubMed]
- Totaro A, Castellan M, Battilana G, et al. YAP/TAZ link cell mechanics to Notch signalling to control epidermal stem cell fate. Nat Commun 2017;8:15206. [Crossref] [PubMed]
- Wagner EF, Eferl R. Fos/AP-1 proteins in bone and the immune system. Immunol Rev 2005;208:126-40. [Crossref] [PubMed]
- Zenz R, Eferl R, Scheinecker C, et al. Activator protein 1 (Fos/Jun) functions in inflammatory bone and skin disease. Arthritis Res Ther 2008;10:201. [Crossref] [PubMed]
- Angel P, Szabowski A, Schorpp-Kistner M. Function and regulation of AP-1 subunits in skin physiology and pathology. Oncogene 2001;20:2413-23. [Crossref] [PubMed]
- Eckert RL, Welter JF. Transcription factor regulation of epidermal keratinocyte gene expression. Mol Biol Rep 1996;23:59-70. [Crossref] [PubMed]
- Yang M, Liang Y, Sheng L, et al. A preliminary study of differentially expressed genes in expanded skin and normal skin: implications for adult skin regeneration. Arch Dermatol Res 2011;303:125-33. [Crossref] [PubMed]
- Southall MD, Li T, Gharibova LS, et al. Activation of epidermal vanilloid receptor-1 induces release of proinflammatory mediators in human keratinocytes. J Pharmacol Exp Ther 2003;304:217-22. [Crossref] [PubMed]
- Koizumi S, Fujishita K, Inoue K, et al. Ca2+ waves in keratinocytes are transmitted to sensory neurons: the involvement of extracellular ATP and P2Y2 receptor activation. Biochem J 2004;380:329-38. [Crossref] [PubMed]
- Elias PM, Ahn SK, Denda M, et al. Modulations in epidermal calcium regulate the expression of differentiation-specific markers. J Invest Dermatol 2002;119:1128-36. [Crossref] [PubMed]
- Trepat X, Deng L, An SS, et al. Universal physical responses to stretch in the living cell. Nature 2007;447:592-5. [Crossref] [PubMed]
- Lehrer RI. In defense of skin. J Invest Dermatol 2005;125:viii-ix. discussion x-xi. [Crossref] [PubMed]
- Vezzoli G, Soldati L, Gambaro G. Roles of calcium-sensing receptor (CaSR) in renal mineral ion transport. Curr Pharm Biotechnol 2009;10:302-10. [Crossref] [PubMed]
- Menon GK, Elias PM, Feingold KR. Integrity of the permeability barrier is crucial for maintenance of the epidermal calcium gradient. Br J Dermatol 1994;130:139-47. [Crossref] [PubMed]
- Wang J, Zhang Y, Zhang N, et al. An updated review of mechanotransduction in skin disorders: transcriptional regulators, ion channels, and microRNAs. Cell Mol Life Sci 2015;72:2091-106. [Crossref] [PubMed]
- Komuves L, Oda Y, Tu CL, et al. Epidermal expression of the full-length extracellular calcium-sensing receptor is required for normal keratinocyte differentiation. J Cell Physiol 2002;192:45-54. [Crossref] [PubMed]
- Calautti E, Cabodi S, Stein PL, et al. Tyrosine phosphorylation and src family kinases control keratinocyte cell-cell adhesion. J Cell Biol 1998;141:1449-65. [Crossref] [PubMed]
- Berridge MJ. Cell signalling. A tale of two messengers. Nature 1993;365:388-9. [Crossref] [PubMed]
- Kirschner N, Poetzl C, von den Driesch P, et al. Alteration of tight junction proteins is an early event in psoriasis: putative involvement of proinflammatory cytokines. Am J Pathol 2009;175:1095-106. [Crossref] [PubMed]
- Zheng J. Molecular mechanism of TRP channels. Compr Physiol 2013;3:221-42. [PubMed]
- Clapham DE, Runnels LW, Strubing C. The TRP ion channel family. Nat Rev Neurosci 2001;2:387-96. [Crossref] [PubMed]
- Tsutsumi M, Denda S, Ikeyama K, et al. Exposure to low temperature induces elevation of intracellular calcium in cultured human keratinocytes. J Invest Dermatol 2010;130:1945-8. [Crossref] [PubMed]
- Bodo E, Biro T, Telek A, et al. A hot new twist to hair biology: involvement of vanilloid receptor-1 (VR1/TRPV1) signaling in human hair growth control. Am J Pathol 2005;166:985-98. [Crossref] [PubMed]
- Toth BI, Dobrosi N, Dajnoki A, et al. Endocannabinoids modulate human epidermal keratinocyte proliferation and survival via the sequential engagement of cannabinoid receptor-1 and transient receptor potential vanilloid-1. J Invest Dermatol 2011;131:1095-104. [Crossref] [PubMed]
- Alexander SP, Benson HE, Faccenda E, et al. The Concise Guide to PHARMACOLOGY 2013/14: catalytic receptors. Br J Pharmacol 2013;170:1676-705. [Crossref] [PubMed]
- Kida N, Sokabe T, Kashio M, et al. Importance of transient receptor potential vanilloid 4 (TRPV4) in epidermal barrier function in human skin keratinocytes. Pflugers Arch 2012;463:715-25. [Crossref] [PubMed]
- Lehen'kyi V, Beck B, Polakowska R, et al. TRPV6 is a Ca2+ entry channel essential for Ca2+-induced differentiation of human keratinocytes. J Biol Chem 2007;282:22582-91. [Crossref] [PubMed]
- Denda M, Tsutsumi M, Goto M, et al. Topical application of TRPA1 agonists and brief cold exposure accelerate skin permeability barrier recovery. J Invest Dermatol 2010;130:1942-5. [Crossref] [PubMed]
- Bezzerides VJ, Ramsey IS, Kotecha S, et al. Rapid vesicular translocation and insertion of TRP channels. Nat Cell Biol 2004;6:709-20. [Crossref] [PubMed]
- Muller M, Essin K, Hill K, et al. Specific TRPC6 channel activation, a novel approach to stimulate keratinocyte differentiation. J Biol Chem 2008;283:33942-54. [Crossref] [PubMed]
- Venkatachalam K, Zheng F, Gill DL. Control of TRPC and store-operated channels by protein kinase C. Novartis Found Symp 2004;258:172-85; discussion 185-8, 263-6.
- Leuner K, Kraus M, Woelfle U, et al. Reduced TRPC channel expression in psoriatic keratinocytes is associated with impaired differentiation and enhanced proliferation. PLoS One 2011;6. [Crossref] [PubMed]
- Gargalionis AN, Papavassiliou KA, Basdra EK, et al. Polycystins: Mechanosensors with Diagnostic and Prognostic Potential in Cancer. Trends Mol Med 2016;22:7-9. [Crossref] [PubMed]
- Dalagiorgou G, Piperi C, Adamopoulos C, et al. Mechanosensor polycystin-1 potentiates differentiation of human osteoblastic cells by upregulating Runx2 expression via induction of JAK2/STAT3 signaling axis. Cell Mol Life Sci 2017;74:921-36. [Crossref] [PubMed]
- Dalagiorgou G, Piperi C, Georgopoulou U, et al. Mechanical stimulation of polycystin-1 induces human osteoblastic gene expression via potentiation of the calcineurin/NFAT signaling axis. Cell Mol Life Sci 2013;70:167-80. [Crossref] [PubMed]
- Gargalionis AN, Korkolopoulou P, Farmaki E, et al. Polycystin-1 and polycystin-2 are involved in the acquisition of aggressive phenotypes in colorectal cancer. Int J Cancer 2015;136:1515-27. [Crossref] [PubMed]
- Varela A, Piperi C, Sigala F, et al. Elevated expression of mechanosensory polycystins in human carotid atherosclerotic plaques: association with p53 activation and disease severity. Sci Rep 2015;5:13461. [Crossref] [PubMed]
- Huang T, Lin X, Meng X, et al. Phosphoinositide-3 kinase/protein kinase-B/mammalian target of rapamycin pathway in psoriasis pathogenesis. A potential therapeutic target? Acta Derm Venereol 2014;94:371-9. [Crossref] [PubMed]
- Buerger C, Malisiewicz B, Eiser A, et al. Mammalian target of rapamycin and its downstream signalling components are activated in psoriatic skin. Br J Dermatol 2013;169:156-9. [Crossref] [PubMed]
- Raychaudhuri SK, Raychaudhuri SP. mTOR Signaling Cascade in Psoriatic Disease: Double Kinase mTOR Inhibitor a Novel Therapeutic Target. Indian J Dermatol 2014;59:67-70. [Crossref] [PubMed]
- Gargalionis AN, Basdra EK, Papavassiliou AG. Tumor mechanosensing and its therapeutic potential. J Cell Biochem 2018. [Epub ahead of print]. [Crossref] [PubMed]
- Gargalionis AN, Basdra EK, Papavassiliou AG. Cancer mechanobiology: Effects and therapeutic perspectives. Int J Cancer 2018;142:1298-9. [Crossref] [PubMed]