Cardiovascular failure and weaning
Introduction
Weaning failure from the ventilator has been estimated to involve up to 42% of mechanically ventilated patients, according to the definition used and underlying comorbidities (1). This clinical event is commonly defined as either the failure of a spontaneous breathing trial (SBT), or the need for tracheal reintubation within 48 h following extubation. Many factors contribute to weaning failure. Failure of extubation is associated with morbidity secondary to prolonged invasive mechanical ventilation and with high mortality rate (1).
Weaning from positive-pressure ventilation has been compared to an exercise stress test for the cardiovascular system (2). Cessation of positive-pressure ventilation induces abrupt hemodynamic changes and an increase in work of breathing which may ultimately result in increased pulmonary venous pressure with associated risk of weaning-induced (hydrostatic) pulmonary oedema (WiPO) (3). Accordingly, inadequate cardiovascular response to spontaneous breathing is one of the leading mechanisms of SBT or extubation failure (1). Patients with underlying left heart disease are at high risk of WiPO, irrespective of the indication of invasive mechanical ventilation (3). Nevertheless, occult cardiovascular insufficiency may also be a primary cause of weaning failure in critically ill patients without known cardiac disease (2).
The present manuscript will concisely summarize the mechanisms underlying weaning failure of cardiovascular origin, describe the diagnostic work-up, and illustrate the use of hemodynamic monitoring—especially with critical care echocardiography (CCE)—for guiding tailored therapeutic management in patients at high-risk of unsuccessful weaning from the ventilator due to a cardiovascular failure during the transition from mechanical ventilation to spontaneous breathing.
Hemodynamic effects induced by the transition from positive-pressure ventilation to spontaneous breathing
The transfer of a patient from invasive mechanical ventilation to spontaneous breathing during a SBT or after extubation is associated with an abrupt increase of both the venous return to the heart and left ventricular (LV) afterload secondary to the inspiratory fall of intra-thoracic pressure (3). In addition, the conjunction of sudden increase in both the work of breathing and adrenergic tone may also raise myocardial oxygen consumption (3). Taken together, these hemodynamic effects may exert an excessive burden on the cardiovascular system and induce myocardial ischemia in predisposed patients, or even participate in weaning failure in many patients without known cardiac disease (2). Marked increase of pulmonary artery occlusion pressure (PAOP) has been measured using right heart catheterization during failure to wean patients with underlying left cardiac disease from the ventilator (3). This increase of PAOP is observed within a few minutes after the disconnection of the patient from the ventilator. Indeed, the abrupt increase of LV filling pressure results from acute changes of cardiac loading conditions and from potential myocardial ischemia induced by the transition from positive-pressure to spontaneous ventilation (4). The inability of the cardiovascular system to sustain the sudden additional workload of breathing in increasing cardiac output without rising LV filling pressure results in WiPO. This accounts for the increased risk of WiPO in heart failure patients (5), and explains the early occurrence of this frequent cause of weaning failure after the transfer from mechanical ventilation to spontaneous breathing (3). In patients with chronic obstructive pulmonary disease (COPD), the exaggerated inspiratory fall of intrathoracic pressure associated with the further increase of myocardial oxygen consumption due to the amplified increase in work of breathing tend to amplify the raise in LV filling pressure. Accordingly, these patients are also at high risk of weaning failure of cardiac origin (3).
Clinical settings and conduction of the SBT
Causes of failure to wean a patient from mechanical ventilation are numerous (6). Leading mechanisms of SBT failure are cardiovascular dysfunction or inability of the respiratory system to support the additional work of breathing (1). Heart failure has been shown to be responsible for up to 42% of unsuccessful SBT in a large cohort of medical ICU patients (7). Recently, WiPO was evidenced in 59% of weaning failure, and both cardiac disease and COPD were evidenced as main independent risk factors (8). Due to the aforementioned effects of the transition from mechanical to spontaneous ventilation on the cardiovascular system, weaning failure of cardiac origin typically occurs early during SBT, rather than later after extubation. Extubation failure after a successful SBT may be related to the same causes, but may also result from upper airway obstruction or from excessive secretions (1). With the exception of post-extubation laryngeal edema which occurs shortly after the tracheal tube removal (9), respiratory or neurologic causes of weaning failure typically develop later after extubation. A high index of suspicion of WiPO typically results from early failure of SBT in a patient with pre-existing left heart failure. Patients with COPD are also at high-risk of unsuccessful weaning of cardiac origin since the increase in airway resistance amplifies LV filling pressure raise during spontaneous breathing (3). Importantly, patients with more subtle or transient myocardial dysfunction may fail only when exposed to the additional workload of weaning (1). In the latter case, patients are not known at high risk of weaning failure of cardiac origin and should therefore be adequately identify to allow prompt diagnosis and adapted therapeutic management (10). Finally, Liu et al. (8) recently showed that 93% of patients who exhibited WiPO had a negative passive leg rising, whereas this maneuver was always positive in patients who succeeded weaning. Dres et al. (11) lately confirmed that a negative passive leg rising was predictive of WiPO. Taken together, these results underline the pivotal contribution of cumulated hydric balance and potentially associated volume overload reflected by a negative passive leg rising (i.e., the absence of preload dependence) in WiPO.
Since prolonged invasive mechanical ventilation is associated with significant morbidity and mortality, readiness to wean should be considered as early as possible in a stabilized patient (1), and subsequently assessed daily (12,13). Current recommendations advocate performing a 30-min SBT consisting in either a T-tube breathing or low levels of pressure support ventilation [typically 5–8 cmH2O with or without a positive end-expiratory pressure (PEEP) of 5 cmH2O] (1). In heart failure patients, a low-pressure support and a low level of PEEP can have significant beneficial hemodynamic effects (5). In contrast, withdrawing even a low PEEP level can result in rapid worsening of LV function, increased filling pressures, and ultimately can precipitate the development of WiPO (4). In a small sample of patients who failed initially a 60-min T-piece trial while exhibiting a baseline median PAOP of 15 mmHg (interquartile range: 12–18 mmHg), Cabello et al. (7) assessed the respiratory and hemodynamic responses to three types of SBTs performed in random order: T-piece trial, pressure support ventilation (7 cmH2O) with low PEEP (5 cmH2O), and pressure support ventilation (7 cmH2O) without PEEP. All patients failed the T-piece trial, whereas 8 (57%) and 11 (79%) of them successfully completed the SBT using pressure support ventilation (7 cmH2O) without PEEP and with low PEEP (5 cmH2O), respectively. Interestingly, both the work of breathing and PAOP level increased to a higher extend in the absence of PEEP, with median values of PAOP reaching 20 to 21 mmHg (7). Taken together, these data suggest that SBT failure of cardiac origin is common in difficult-to-wean patients and that the T-piece trial elicits the greatest burden on respiratory and cardiocirculatory functions during SBT. Accordingly, the T-piece trial appears as a reasonable choice to unmask the development of LV heart failure during spontaneous breathing in patients with impaired cardiac function. Since heart failure patients require a higher threshold for extubation, a successful T-piece trial appears to sensitively assess their ability to tolerate fully unsupported spontaneous breathing (5,13). This strategy may be extended to any patient at high risk of weaning failure, such as suspected or documented marginal LV systolic function and/or LV diastolic dysfunction, especially in the presence of volume overload. In these patients, a failed T-piece trial indicates the need for further optimization of cardiac loading conditions prior to and after a planned extubation to prevent re-intubation. Hemodynamic assessment at the time of SBT failure is then crucial to best guide further therapeutic strategy in order to optimize the weaning process (10).
Diagnosing weaning failure of cardiac origin
Diagnosis is straightforward when a florid WiPO develops shortly after the initiation of a SBT in a cardiac patient. In contrast, weaning failure of cardiac origin may be more challenging to identify in the setting of previously unrecognized left heart disease (6). In addition, the precise mechanism of the cardiovascular failure requires a comprehensive hemodynamic assessment at the precise time of SBT failure (4). The diagnosis of WiPO historically relies on the measurement of an increased PAOP, with 18 mmHg being widely considered as a classical cut-off value (3). Nevertheless, the increase of PAOP during SBT is fairly limited in patients without cardiac disease (3), and the use of right heart catheterization in ICU patients regularly diminishes over time due to its invasiveness and intrinsic limitations (14). Accordingly, the diagnosis of WiPO currently relies on non-invasive approaches, including transthoracic echocardiography, cardiac biomarkers, biological signs of hemoconcentration, or measurement of extravascular pulmonary lung water (6,15). Nevertheless, studies failed to assess and compare the respective diagnostic value of these various approaches to identify weaning failure of cardiac origin specifically in heart failure patients.
Transthoracic echocardiography
Critical care ultrasonography is best suited to precisely identify both the diagnosis and mechanism of weaning failure of cardiac origin, since it combines transthoracic echocardiography and chest ultrasonography which are portable, non-invasive, and easy-to-use imaging methods (10,16). In patients with acute respiratory failure, the echocardiographic diagnosis of cardiogenic pulmonary edema relies on the identification of elevated LV filling pressures which are usually associated with a LV diastolic dysfunction of variable severity, irrespective of systolic function (17). Accordingly, the same diagnostic approach applies for WiPO resulting in a failed SBT or in a failed extubation. Not surprisingly, patients with LV diastolic failure—especially of high grade—have a higher rate of SBT failure than patients with normal relaxation (18). Most of echocardiographic studies have emphasized the important role of LV diastolic dysfunction rather than LV systolic failure during unsuccessful weaning (15).
Mitral pulse wave Doppler in conjunction with Tissue Doppler Imaging applied at the level of the mitral ring are commonly used to assess LV diastolic properties and resulting filling pressures. The derived index E/E’ (i.e., the maximal spectral Doppler velocity of early diastolic mitral inflow normalized by the maximal tissue Doppler velocity measured at the lateral and/or septal aspects of the mitral ring) is widely advocated to estimate LV filling pressure. Lateral E/E’ value ≤8 is consistent with a PAOP ≤18 mmHg (19). In contrast, higher E/E’ values have been reported in patients who underwent unsuccessful SBT. Threshold values of E/E’ to predict SBT failure differ between studies (range: 7.8 to 14.5) due to distinct sites of E’ measurement (lateral, septal, or both aspects of mitral ring), different characteristics of study populations, and variable cumulative fluid balance at the time of SBT (20-22). We (23) and others (24) have previously showed that patients who failed SBT exhibited a significantly lower LV ejection fraction and higher E/E’ before the T-piece trial, when compared to those who were successfully weaned. Not surprisingly, E/E’ values tend to be higher during a T-piece trial than during a low-level pressure support trial without PEEP (10), the difference being not significant in a small study sample (25). During SBT, CCE studies uniformly reported an increase of LV filling pressure (i.e., higher E/E’ and/or E/A) (20,21,23,26,27). In a recent meta-analysis including 7 studies with a total of 433 patients, a higher baseline E/E’ ratio and an increase of LV filling pressure during SBT were significantly associated with weaning failure (18). Nevertheless, a marked heterogeneity among studies precluded the determination of a consistent and reliable E/E’ cutoff value to accurately predict weaning failure (18). Finally, Moschietto et al. (21) showed that E’ maximal velocity significantly increased during SBT in patients who were successfully weaned, whereas it failed to do so in patients who failed. This suggests that the inability to improve relaxation during the adrenergic release at the time of SBT may represent an important factor contributing to weaning failure of cardiac origin (21). In keeping with these results, Ruiz-Bailén et al. (28) recently showed that silent diastolic and systolic dysfunction could have a major impact on ventilator weaning in performing stress echocardiography in a population of cardiac patients who had been mechanically ventilated. In patients who failed weaning, dobutamine echocardiography disclosed an increased E/E’ ratio and a deterioration of LV systolic performance (reduced strain and strain rate), when compared to those who were successfully weaned (28).
Transthoracic echocardiography also allows clear depiction of alternative mechanisms of WiPO, such as SBT-induced or SBT-increased mitral regurgitation. Although not operant in cardiogenic pulmonary edema with preserved LV ejection fraction (29), the volume of ischemic mitral regurgitation may abruptly increase during exercise and changes in cardiac loading conditions in patients with LV systolic dysfunction (30). Similarly, the severity of mitral regurgitation tends to increase during SBT (26). These results have been elegantly confirmed in a recent study using ephedrine stress echocardiography in patients without apparent LV systolic or diastolic dysfunction, but functional moderate-to-severe mitral regurgitation (28). Effective regurgitant volume and orifice area significantly increased as a result of raised LV afterload, reflecting the potential contribution of transiently worsened mitral regurgitation during the weaning process (28). Occult mitral regurgitation is frequently encountered in patients with coronary artery disease (31). Since ischemic mitral insufficiency is a “dynamic” disease which severity may vary over time (30), regurgitant volume may worsen during weaning as a result of increased adrenergic tone and LV afterload, or even due to SBT-induced myocardial ischemia. Rarely, weaning pulmonary edema may be related to a severe mitral regurgitation secondary to a systolic anterior motion of the mitral valve in patients with hypertrophic cardiomyopathy. In this case, transthoracic echocardiography is diagnostic in depicting the association of a dynamic LV outflow tract obstruction and severe mitral regurgitation, whereas right cardiac catheterization leads to the erroneous diagnosis of left heart failure due to the presence of markedly elevated PAOP (32). Finally, transthoracic echocardiography is an unparalleled approach to identify associated cardiac disease at the origin of exaggerated raise in LV filling pressures during SBT (e.g., ischemic, hypertrophic or infiltrative cardiomyopathy). Although of great clinical value, CCE is operator-dependent, requires a specific training to reach advanced competency, and may be limited by inadequate image quality, especially in COPD patients.
Cardiac biomarkers
B-type natriuretic peptide (BNP) and N-terminal proBNP (NT-proBNP) are peptides which are released by the ventricular myocytes in response to stretch, hence any distention of ventricular walls secondary to increased intra-cavitary pressure. Accordingly, numerous studies have evaluated the diagnostic accuracy of these peptides to predict weaning failure in measuring circulating levels prior to and during SBT (3,15). Mekontso-Dessap et al. (33) showed that plasma BNP concentration before SBT was higher in patients with weaning failure. Nevertheless, no hemodynamic assessment was performed to ascertain the cardiac origin of weaning failure. Chien et al. (34) reported that SBT-induced increase of circulating BNP level predicted weaning failure, rather than baseline concentration. Similar results were reported for NT-proBNP and increased circulating level of the peptide was related to SBT-induced rise in LV filling pressure (26). Zapata et al. (35) showed that both BNP and NT-proBNP could predict weaning failure of cardiac origin (PAOP >18 mmHg or Doppler consistent with elevated LV filling pressure), and found that BNP performed better than NT-proBNP. In another study, an increase of BNP concentration exceeding 12% during SBT was shown predictive of WiPO with a sensitivity of 78% and a specificity of 78% (36). In contrast, Ait-Oufella et al. (37) reported significant increase of plasma BNP concentration during SBT, whether patients could be successfully weaned from the ventilator or not. Finally, Mekontso Dessap et al. (38) have shown that guiding fluid management of ventilated ICU patients based on daily measurement of plasma BNP concentration significantly reduced fluid balance and shortened the duration of mechanical ventilation, especially in the presence of LV dysfunction. Nevertheless, this therapeutic strategy was not tested in patients with WiPO.
Overall, the ability of BNP and NT-proBNP to accurately predict weaning failure of cardiac origin remains debated in the current literature. Since SBT is easy to perform and provides valuable clinical information on the ability of the patient to sustain spontaneous breathing, the additional diagnostic value of these peptide concentrations remains questionable (15). In addition, BNP and NT-proBNP plasma concentrations can be significantly increased in the absence of left heart dysfunction (e.g., advanced age, sepsis, renal failure, pulmonary hypertension…). Whether routine measurement of BNP or NT-proBNP plasma concentration before SBT could be used as a screening tool to identify patients with severe global condition who are at high-risk of weaning failure due to underlying elevated cardiac filling pressure and who should therefore be monitored closely (e.g., transthoracic echocardiography) to best guide ventilator weaning remains to elucidate.
Biological markers of hemoconcentration
As a hydrostatic pulmonary edema, WiPO is thought to be associated with a transfer of hypo-oncotic fluid from pulmonary capillaries towards lung interstitium which may result in hemoconcentration (3). Anguel et al. (39) found that an increase of plasma protein concentration greater than 6% during SBT predicted WiPO with a sensitivity of 87% and a specificity of 95%. When compared to transthoracic echocardiography, Pottier et al. (40) reported the absence of additional value of biological markers of hemoconcentration (protein and hemoglobin levels) for the diagnosis of WiPO. Similar to cardiac biomarkers, this simple biological test fails to directly quantify the elevation of LV filling pressure and to identify potentially unsuspected related mechanisms (e.g., transient mitral regurgitation and/or myocardial ischemia).
Increased extravascular lung water
In the absence of LV filling pressure assessment, the detection of extravascular lung water during a SBT allows linking a potential failure of the test to the development of WiPO (15). Lung ultrasonography has been validated for the diagnosis of interstitial pulmonary edema, when depicting B-lines (instead of A-lines) (10,16). A semi-quantitative assessment of the severity of interstitial pulmonary edema has been proposed (41). The presence of pulmonary consolidation suggests underlying alveolar filling (42). Nevertheless, lung ultrasonography fails to distinguish between cardiogenic and inflammatory causes of pulmonary edema, irrespective of its severity (10). Accordingly, lung ultrasonography should be associated with echocardiography to ascertain that weaning failure is of cardiac origin (43).
Transpulmonary thermodilution allows measuring extravascular lung water using a specific algorithm. In difficult-to-wean patients, Dres et al. (36) showed that an increase of extravascular lung water greater or equal to 14% during a SBT indicated the development of a WiPO with a sensitivity of 67% and a specificity of 100%. Nevertheless, this approach requires the presence of both a dedicated femoral artery catheter and central venous line at the time of SBT, and fails to directly document the increase of LV filling pressure and its underlying mechanism (15).
Use of critical care echocardiography for conducting SBT in high-risk patients
CCE is performed and interpreted at bedside by intensivists specifically trained to establish diagnoses and guide therapeutic management, especially in patients with cardiopulmonary compromise (44). Owing to its ease of use, portability, non-invasiveness, anatomical and functional real-time assessment, CCE is currently recommended as the first-line modality to assess patients with shock or with acute respiratory failure, and is more used as a monitoring rather than a diagnostic tool to better guide tailored management of unstable patients (45). According to image quality and indication, transesophageal echocardiography may represent a valuable adjunct in the diagnostic work-up of a ventilated patient under sedation after a weaning failure, but it requires a specific training (46). This explains the pivotal role of CCE in the setting of patients at high risk of weaning failure of cardiac origin (Table 1).
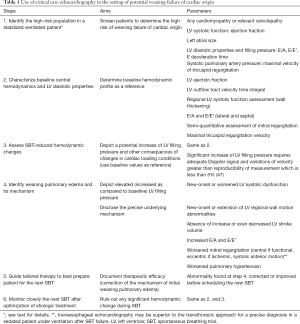
Full table
Practically, CCE should be used systematically in cardiac patients before initiating the weaning process since this population is intrinsically at high risk of WiPO (5,48). In patients without known cardiac disease but presenting with a past medical history or comorbidities potentially consistent with borderline LV systolic function, abnormal LV diastolic properties, (severe) pulmonary hypertension, and/or positive cumulative fluid balance, CCE should be used as a screening tool to objectively identify those who are at high risk of weaning failure of cardiac origin (49,50). Before SBT, patients with a high E/E’ value, significant left-sided valvulopathy (especially mitral regurgitation), LV outflow tract obstruction, pulmonary hypertension, especially in the presence of a depressed LV systolic function or an underlying cardiomyopathy known to be associated with LV diastolic dysfunction should be closely monitored using CCE during the weaning process (Table 1). As for difficult-to-wean patients (13), the T-piece trial should then be chosen to increase the sensitivity of SBT in order to unmask a compensated heart failure under positive-pressure ventilation. In all these patients, CCE should be performed immediately prior to (i.e., typically under pressure support with ongoing ventilator settings) and during the SBT (typically a T-piece trial) to depict any significant increase of LV filling pressure, since there is no reliable threshold value of E/E’ that can be uniformly used to predict weaning failure (18). Practically, CCE assessment is performed using transthoracic echocardiography immediately before ventilator disconnection and after 15 to 30 minutes of T-piece trial, or earlier in the presence of clinical signs of weaning failure (51). This hemodynamic assessment should be goal-oriented to be rapidly performed (51), focused on the semi-quantitative evaluation of LV filling pressure and systolic pulmonary pressure, and seek for any transient but relevant mitral regurgitation (Table 1). In an asymptomatic patient, the absence of (I) significant increase of E/E’, (II) substantial augmentation of systolic pulmonary artery pressure, (III) SBT-induced mitral regurgitation or worsening of its severity compared to baseline, and (IV) relevant regional wall motion abnormality or new-onset LV systolic dysfunction allows considering extubation with a low risk of failure. A significant increase of E’ maximal velocity reflecting improved LV relaxation during SBT could constitute an additional factor predicting a successful weaning (21). In contrast, early clinical failure (typically within a few minutes) of SBT should trigger a prompt CCE assessment to confirm the WiPO (elevated LV filling pressure) and to depict its precise mechanism (17). In this case, additional transesophageal echocardiographic assessment may be performed once the patient has been sedated and reconnected to the ventilator in the presence of suboptimal surface image quality (10,46). This new CCE evaluation allows tailoring medical therapy to optimally prepare the difficult-to-wean patient to a new SBT (52). It is then recommended to consider a new T-piece trial after 24 hours of assist-control ventilation and adapted therapy, and subsequently on a daily basis (13).
Once the patient has been reconnected to the ventilator and switched to assist-control ventilation, the treatment of WiPO relies on the identification of its mechanism (Table 1). Volume overload (excessive preload) responsible for increased LV filling pressure should trigger diuretic therapy. Liu et al. (8) recently showed that patients with initial WiPO could subsequently be extubated successfully after fluid removal by diuretics and vasodilator therapy when passive leg rising became positive. Accordingly, authors suggest that correction of fluid overload should aim at reaching a state of preload dependency (8). Excessive afterload is suspected on the abrupt increase of systolic blood pressure contemporary to SBT-induced rise of LV filling pressure. Additional echocardiographic findings consistent with afterloaded left heart are the acute reduction of LV ejection fraction and the worsening of a pre-existing mitral regurgitation. In this case, intravenous administration of vasodilators should be initiated, including using initial intravenous boluses to rapidly decrease systolic blood pressure, hence LV afterload. Nitrates appear as a reasonable choice if excessive afterload is associated with volume overload and/or new-onset regional wall motion abnormalities consistent with SBT-induced myocardial ischemia. When SBT-induced adrenergic release results in a systolic anterior motion of the mitral valve with associated acute and severe mitral regurgitation at the origin of a WiPO, beta-blockers are the treatment of choice (32). In contrast, Dobutamine could be deleterious in this clinical setting since it would amplify LV outflow tract dynamic obstruction and associated rise of filling pressure. Dobutamine is usually not indicated in weaning failure of cardiac origin because contemporary increase of PAOP is usually not associated with a decreased cardiac output (4,20). In addition, further adrenergic stimulation by a β1-agonist agent could be deleterious in increasing myocardial oxygen consumption with its inherent risk of myocardial ischemia in predisposed patients (3,15). Levosimendan has been successfully used in patients with a dilated cardiomyopathy who failed weaning (53), but cannot be currently recommended. Once the mechanism of WiPO corrected or improved, the patients should be closely monitored using CCE during the next SBT (Table 1).
After extubation, careful optimization of loading conditions and liberal use of non-invasive positive-pressure ventilation are crucial to best prevent re-intubation (5). In ICU patients who were extubated after a successful SBT, Silva et al. (54) elegantly demonstrated that a combined thoracic ultrasound approach encompassing transthoracic echocardiography, lung and diaphragm ultrasound allowed predicting subsequent respiratory distress and accurately identified its mechanism (i.e., cardiac or respiratory origin). The identification of lung interstitial edema and elevated LV filling pressure were the strongest predictors of post-extubation respiratory failure (54). Overall, a combined approach based on ultrasonography evaluation of the heart, the diaphragm, the pleura and the lung appear as a sound strategy to best guide tailored therapeutic management of patients at high risk of weaning failure (16).
Conclusions
Cardiac patients are at high-risk of weaning failure due to WiPO since the transition from positive-pressure invasive ventilation to spontaneous breathing may elicit an excessive burden on both the respiratory and cardiovascular systems. Numerous patients without known cardiac disease are also prone to weaning failure of cardiac origin due to marginal systolic function or LV diastolic dysfunction, especially when associated with a marked positive fluid balance. Although various approaches based on cardiac biomarkers, biological signs of hemoconcentration, or measurement of extravascular lung water have been proposed for the diagnosis of WiPO, CCE is the only non-invasive method which allows real-time identification of WiPO and of its underlying mechanism. Accordingly, CCE is ideally suited for detecting high-risk patients of weaning failure who should be monitored during and after the SBT, and to guide tailored therapy in those who failed. The impact on patient-centered outcomes of such integrated management strategy deserves to be prospectively tested in a large population of patients at high risk of weaning failure of cardiac origin.
Acknowledgements
None.
Footnote
Conflicts of Interest: The author has no conflicts of interest to declare.
References
- Boles JM, Bion J, Connors A, et al. Weaning from mechanical ventilation. Eur Respir J 2007;29:1033-56. [Crossref] [PubMed]
- Pinsky MR. Breathing as exercise: the cardiovascular response to weaning from mechanical ventilation. Intensive Care Med 2000;26:1164-6. [Crossref] [PubMed]
- Teboul JL, Monnet X, Richard C. Weaning failure of cardiac origin: recent advances. Crit Care 2010;14:211. [Crossref] [PubMed]
- Lemaire F, Teboul JL, Cinotti L, et al. Acute left ventricular dysfunction during unsuccessful weaning from mechanical ventilation. Anesthesiology 1988;69:171-9. [Crossref] [PubMed]
- Kuhn BT, Bradley LA, Dempsey TM, et al. Management of mechanical ventilation in decompensated heart failure. J Cardiovasc Dev Dis 2016;3:33. [Crossref] [PubMed]
- Perren A, Brochard L. Managing the apparent and hidden difficulties of weaning from mechanical ventilation. Intensive Care Med 2013;39:1885-95. [Crossref] [PubMed]
- Cabello B, Thille AW, Roche-Campo F, et al. Physiological comparison of three spontaneous breathing trials in difficult-to-wean patients. Intensive Care Med 2010;36:1171-9. [Crossref] [PubMed]
- Liu J, Shen F, Teboul JL, et al. Cardiac dysfunction induced by weaning from mechanical ventilation: incidence, risk factors, and effects of fluid removal. Crit Care 2016;20:369. [Crossref] [PubMed]
- François B, Bellissant E, Gissot V, et al. 12-h pretreatment with methylprednisolone versus placebo for prevention of postextubation laryngeal edema: a randomised double-blind trial. Lancet 2007;369:1083-9. [Crossref] [PubMed]
- Vignon P, Repessé X, Vieillard-Baron A, et al. Critical ultrasonography in acute respiratory failure. Crit Care 2016;20:228. [Crossref] [PubMed]
- Dres M, Teboul JL, Anguel N, et al. Passive leg raising performed before a spontaneous breathing trial predicts weaning-induced cardiac dysfunction. Intensive Care Med 2015;41:487-94. [Crossref] [PubMed]
- Girard TD, Kress JP, Fuchs BD, et al. Efficacy and safety of a paired sedation and ventilator weaning protocol for mechanically ventilated patients in intensive care (Awakening and Breathing Controlled trial): a randomised controlled trial. Lancet 2008;371:126-34. [Crossref] [PubMed]
- Frutos-Vivar F, Esteban A. Our paper 20 years later: how has withdrawal from mechanical ventilation changed? Intensive Care Med 2014;40:1449-59. [Crossref] [PubMed]
- Raper R, Sibbald WJ. Misled by the wedge? The Swan-Ganz catheter and left ventricular preload. Chest 1986;89:427-34. [Crossref] [PubMed]
- Teboul JL. Weaning-induced cardiac dysfunction: where are we today? Intensive Care Med 2014;40:1069-79. [Crossref] [PubMed]
- Mayo P, Volpicelli G, Lerolle N, et al. Ultrasonography evaluation during the weaning process: the heart, the diaphragm, the pleura and the lung. Intensive Care Med 2016;42:1107-17. [Crossref] [PubMed]
- Vignon P, Colreavy F, Slama M. Pulmonary edema: which role for echocardiography in the diagnostic work-up? In: De Backer D, Cholley BP, Slama M, et al. editors. Hemodynamic monitoring using echocardiography in the critically ill. Springer, 2011:177-94.
- de Meirelles Almeida CA, Nedel WL, Morais VD, et al. Diastolic dysfunction as a predictor of weaning failure: A systematic review and meta-analysis. J Crit Care 2016;34:135-41. [Crossref] [PubMed]
- Vignon P. Echocardiographic assessment of pulmonary artery occlusion pressure in ventilated patients: a transesophageal study. Crit Care 2008;12:R18. [Crossref] [PubMed]
- Lamia B, Maizel J, Ochagavia A, et al. Echocardiographic diagnosis of pulmonary artery occlusion pressure elevation during weaning from mechanical ventilation. Crit Care Med 2009;37:1696-701. [Crossref] [PubMed]
- Moschietto S, Doyen D, Grech L, et al. Transthoracic Echocardiography with Doppler Tissue Imaging predicts weaning failure from mechanical ventilation: evolution of the left ventricle relaxation rate during a spontaneous breathing trial is the key factor in weaning outcome. Crit Care 2012;16:R81. [Crossref] [PubMed]
- Papanikolaou J, Makris D, Saranteas T, et al. New insights into weaning from mechanical ventilation: left ventricular diastolic dysfunction is a key player. Intensive Care Med 2011;37:1976-85. [Crossref] [PubMed]
- Caille V, Amiel JB, Charron C, et al. Echocardiography: a help in the weaning process. Crit Care 2010;14:R120. [Crossref] [PubMed]
- Papaioannou VE, Stakos DA, Dragoumanis CK, et al. Relation of tricuspid annular displacement and tissue Doppler imaging velocities with duration of weaning in mechanically ventilated patients with acute pulmonary edema. BMC Cardiovasc Disord 2010;10:20. [Crossref] [PubMed]
- Schifelbain LM, Vieira SR, Brauner JS, et al. Echocardiographic evaluation during weaning from mechanical ventilation. Clinics 2011;66:107-11. [Crossref] [PubMed]
- Gerbaud E, Erickson M, Grenouillet-Delacre M, et al. Echocardiographic evaluation and N-terminal pro-brain natriuretic peptide measurement of patients hospitalized for heart failure during weaning from mechanical ventilation. Minerva Anestesiol 2012;78:415-25. [PubMed]
- Abdelbary A, Nassar Y, Hussein K, et al. Can We Predict Left Ventricular DysfunctionInduced Weaning Failure? Invasive & Echocardiographic Evaluation. J Am Sci 2011;7:312-9.
- Ruiz-Bailén M, Cobo-Molinos J, Castillo-Rivera A, et al. Stress echocardiography in patients who experienced mechanical ventilation weaning failure. J Crit Care 2017;39:66-71. [Crossref] [PubMed]
- Gandhi SK, Powers JC, Nomeir AM, et al. The pathogenesis of acute pulmonary edema associated with hypertension. N Engl J Med 2001;344:17-22. [Crossref] [PubMed]
- Piérard LA, Lancellotti P. The role of ischemic mitral regurgitation in the pathogenesis of acute pulmonary edema. N Engl J Med 2004;351:1627-34. [Crossref] [PubMed]
- Stone GW, Griffin B, Shah PK, et al. Prevalence of unsuspected mitral regurgitation and left ventricular diastolic dysfunction in patients with coronary artery disease and acute pulmonary edema associated with normal or depressed left ventricular systolic function. Am J Cardiol 1991;67:37-41. [Crossref] [PubMed]
- Adamopoulos C, Tsagourias M, Arvaniti K, et al. Weaning failure from mechanical ventilation due to hypertrophic obstructive cardiomyopathy. Intensive Care Med 2005;31:734-7. [Crossref] [PubMed]
- Mekontso-Dessap A, de Prost N, Girou E, et al. B-type natriuretic peptide and weaning from mechanical ventilation. Intensive Care Med 2006;32:1529-36. [Crossref] [PubMed]
- Chien JY, Lin MS, Huang YC, et al. Changes in B-type natriuretic peptide improve weaning outcome predicted by spontaneous breathing trial. Crit Care Med 2008;36:1421-6. [Crossref] [PubMed]
- Zapata L, Vera P, Roglan A, et al. B-type natriuretic peptides for prediction and diagnosis of weaning failure from cardiac origin. Intensive Care Med 2011;37:477-85. [Crossref] [PubMed]
- Dres M, Teboul JL, Anguel N, et al. Extravascular lung water, B-type natriuretic peptide, and blood volume contraction enable diagnosis of weaning-induced pulmonary edema. Crit Care Med 2014;42:1882-9. [Crossref] [PubMed]
- Ait-Oufella H, Tharaux PL, Baudel JL, et al. Variation in natriuretic peptides and mitral flow indexes during successful ventilatory weaning: a preliminary study. Intensive Care Med 2007;33:1183-6. [Crossref] [PubMed]
- Mekontso Dessap A, Roche-Campo F, Kouatchet A, et al. Natriuretic peptide-driven fluid management during ventilator weaning: a randomized controlled trial. Am J Respir Crit Care Med 2012;186:1256-63. [Crossref] [PubMed]
- Anguel N, Monnet X, Osman D, et al. Increase in plasma protein concentration for diagnosing weaning-induced pulmonary oedema. Intensive Care Med 2008;34:1231-8. [Crossref] [PubMed]
- Pottier V, Valette X, Seguin A, et al. Diagnostic accuracy of hemoconcentration for pulmonary edema as the cause of weaning failure. Minerva Anestesiol 2016;82:419-28. [PubMed]
- Volpicelli G, Skurzak S, Boero E, et al. Lung ultrasound predicts well extravascular lung water but is of limited usefulness in the prediction of wedge pressure. Anesthesiology 2014;121:320-7. [Crossref] [PubMed]
- Via G, Lichtenstein D, Mojoli F, et al. Whole lung lavage: a unique model for ultrasound assessment of lung aeration changes. Intensive Care Med 2010;36:999-1007. [Crossref] [PubMed]
- Bataille B, Riu B, Ferre F, et al. Integrated use of bedside lung ultrasound and echocardiography in acute respiratory failure: a prospective observational study in ICU. Chest 2014;146:1586-93. [Crossref] [PubMed]
- Mayo PH, Beaulieu Y, Doelken P, et al. American College of Chest Physicians/La Société de Réanimation de Langue Française statement on competence in critical care ultrasonography. Chest 2009;135:1050-60. [Crossref] [PubMed]
- Vignon P. What is new in critical care echocardiography? Crit Care 2018;22:40. [Crossref] [PubMed]
- Vignon P, Merz TM, Vieillard-Baron A. Ten reasons for performing hemodynamic monitoring using transesophageal echocardiography. Intensive Care Med 2017;43:1048-51. [Crossref] [PubMed]
- Vignon P, Allot V, Lesage J, et al. Diagnosis of left ventricular diastolic dysfunction in the setting of acute changes in loading conditions. Crit Care 2007;11:R43. [Crossref] [PubMed]
- McMurray JJ, Adamopoulos S, Anker SD, et al. ESC Guidelines for the diagnosis and treatment of acute and chronic heart failure 2012: The Task Force for the Diagnosis and Treatment of Acute and Chronic Heart Failure 2012 of the European Society of Cardiology. Developed in collaboration with the Heart Failure Association (HFA) of the ESC. Eur Heart J 2012;33:1787-847. [Crossref] [PubMed]
- Paulus WJ, Tschöpe C, Sanderson JE, et al. How to diagnose diastolic heart failure: a consensus statement on the diagnosis of heart failure with normal left ventricular ejection fraction by the Heart Failure and Echocardiography Associations of the European Society of Cardiology. Eur Heart J 2007;28:2539-50. [Crossref] [PubMed]
- Ritzema JL, Richards AM, Crozier IG, et al. Serial Doppler echocardiography and tissue Doppler imaging in the detection of elevated directly measured left atrial pressure in ambulant subjects with chronic heart failure. JACC Cardiovasc Imaging 2011;4:927-34. [Crossref] [PubMed]
- Voga G. Early and simple detection of diastolic dysfunction during weaning from mechanical ventilation. Crit Care 2012;16:137. [Crossref] [PubMed]
- Vignon P. Ventricular diastolic abnormalities in the critically ill. Curr Opin Crit Care 2013;19:242-9. [Crossref] [PubMed]
- Meaudre E, Jego C, Goutorbe P, et al. Weaning failure from mechanical ventilation due to dilated cardiomyopathy: successful use of levosimendan. Acta Anaesthesiol Scand 2009;53:416-7. [Crossref] [PubMed]
- Silva S, Ait Aissa D, Cocquet P, et al. Combined Thoracic Ultrasound Assessment during a Successful Weaning Trial Predicts Postextubation Distress. Anesthesiology 2017;127:666-74. [Crossref] [PubMed]