Interactive translational research model and cadaveric simulation: where minimally invasive cardiac surgery and industry meet
Techniques in minimally invasive cardiac surgery (MICS) have developed over the past decade (1-6). As the limits of what is possible continue to be challenged, minimally invasive approaches rival the open, maximally invasive surgical techniques which have been established as the “gold standard”. Indeed, as minimally invasive techniques continue to evolve, MICS may supplant traditional approaches as the therapeutic modality of choice.
The impetus for more rapid development in MICS is multifactorial. The need to avoid the morbidity of a conventional sternotomy has been one driving factor (3,5). Complications associated with sternotomies have been well documented in the literature, including increased blood loss, prolonged postoperative recovery, sternal dehiscence, soft tissue trauma, deep sternal wound infections, mediastinitis, osteomyelitis, prolonged ventilator dependence, and suboptimal cosmesis (5-8). Other factors driving the expedited development of MICS may be categorized as patient related, surgeon related, and cost related. In terms of patient related factors, cardiac surgery has not been immune to the modern wave of patient driven demand for less invasive procedures and their potential benefits of improved cosmesis, decreased pain, less recovery time, and faster return to work or daily activities (3). Similarly, surgical providers seek to advance surgical procedures and technologies not only to provide the best possible options for their patients in terms of treating disease in the least harmful way possible, but also to stay relevant in a field that is ever-changing (1,2,6). Finally, the cost burden of healthcare in the United States continues to grow at an alarming rate. Longer ICU and hospital stays, higher surgical complication rates, and lower patient satisfaction scores due to suboptimal pain management, resulting in lower hospital reimbursements may be positively correlated to a higher degree of invasiveness of the surgical procedure. In addition, longer recovery times may result in increased personal and societal costs due to lost workplace productivity.
With MICS initially explored starting in the mid-1990’s, the development of minimally invasive procedures in cardiac surgery has lagged behind general surgery and other surgical specialties (9,10). This delay was largely due to challenges that are unique to cardiac surgery, specifically questions regarding cannulation strategies and the feasibility and efficacy of performing procedures that are of a much higher order of complexity with limited exposure and visualization (10).
Partnership between surgery and industry: the interactive model
As innovations in perfusion techniques, cannulation strategies, echocardiography, surgical instrumentation and surgical technologies, including innovations in videoscopic imaging developed, the notion of MICS became reality. The continued evolution of MICS depends on the symbiotic relationship between cardiac surgery and industry, their parallel growth, and their ongoing collaboration.
In an editorial by Charles et al. on the role of surgeons in translational research, they described how surgeons are uniquely qualified for translational research due to the nature of their intimate surgeon-patient relationship, their immersion in clinical and surgical practice, and their ability to recognize the limitations of current therapies (11). Armed with these advantages, they argued that surgeons and surgically trained innovators are best able to identify clinical problems, study them in the lab, and then return to the bedside with improvements in care, thus eliminating the disconnect between research and the application of that research in a clinical setting (11). They concluded that this model is the most efficient way to ensure that scholarly research efforts yield maximal tangible improved clinical outcomes for patients (11).
While these points are valid, this argument may be taken a step further by saying that surgeons and industry make each other better and it is the collaborative effort between these groups that is the most efficient way to translate research efforts into improved patient care. The real time observation of obtaining surgical access, the conduct of the operation, and application of devices and technology in a surgical setting, reduces the clinical disconnect between non-surgically trained inventors, designers, and engineers. Conversely, real time proctoring, two-way feedback, and the freedom to explore operative strategies using the cadaver model removes the intangible barriers that surgeons face, which often hinder the creation and development of a MICS program.
In this interactive translational research model, the surgeon-innovator who is adept at delineating the clinical problem, articulates the problem and guides industry to further develop device prototypes to address the clinical problem. Surgeon feedback on device and technology performance can direct device modification. Similarly, representative professionals from industry may observe challenges uncovered intraoperatively and provide insight based on their expertise on how best to trouble shoot. Taking these lessons into account, modifications in surgical exposure, device design, and application of existing technologies are made, reassessed in the simulated surgical setting, and revised until the optimal approach, surgical instrumentation, and incorporated technologies are developed. In addition to these modifications, innovative devices may be designed in response to a technical challenge that was uncovered during the simulation lab. In this manner, each group may challenge the other to become comfortable being uncomfortable as they challenge the “gold standard” and pursue uncharted surgical territory, with the ultimate, shared goal of improving patient care.
Advantages of the cadaveric simulation model
The cadaver is well suited for surgical simulation, particularly in defined minimally invasive cardiac surgical techniques. The cadaver model most closely resembles realistic operating conditions and clinical operative experience, which cannot be fully replicated by inanimate simulators or animal models (12,13). Cadaveric simulation is equally beneficial to inventors, developers, and engineers in the medical industry, as well as surgeons and trainees. Benefits common to both surgeons and industry include the ability to evaluate optimal device placement, the quality of tissue handling by the device, the ease of achieving adequate tissue-device interface, and optimal incorporation of supporting technologies. Industry-specific benefits include the ability to perform device testing and accurately evaluate device performance in a technically realistic simulated operative environment. Also, cadaveric simulation allows devices and incorporated technology to be evaluated during demonstration of actual surgical procedures for which the device and technology was intended, within an operating room setting. Similarly, cadaveric simulation is beneficial for surgeons as well. Just as the operating room is not the ideal place for early surgical training, the operating room is also not the ideal setting for a surgeon to develop and master minimally invasive approaches to complex cardiac surgical procedures. Surgical simulation allows for the safe acquisition of skills and incorporation of technology, such as video assistance, which facilitates smaller access incisions. It also allows for deliberate practice, in which training tasks may be performed with focused concentration toward a specific training goal, with immediate feedback and ample opportunities to repeat the training task with gradual improvement, until superior performance and expertise skill level is achieved (14). In this manner, prerequisite psychomotor skills, spatial judgement, and device knowledge are attained to the point that these skills become automated (15). Anatomy through a minimally invasive vantage point becomes sufficiently familiar, and the learning curve of using videoscopic assistance is overcome. Hence, surgeons develop the skill sets needed to perform these complex procedures with equivalent or better efficacy through an incision that focuses visualization and exposure on the operative pathology (15). According to Dearani et al., orchestration of an operation requires both structured steps and improvised steps (16). As skills required to perform minimally invasive cardiac surgical procedures become optimized and automated through deliberate practice in the cadaver lab, ability to perform these skills is transferable to the actual clinical operative setting. This transference of skills will allow surgeons to focus on learning operative strategies and management of intraoperative complications during valuable operating room time, dramatically reduce their initial learning curve, and have a greater sense of comfort and confidence in their minimally invasive operative skills and use of new devices and technologies which make the minimally invasive approach possible (14-16).
The culmination of the interactive translational research model is a well-executed, effortless operation that results in improved patient care, less surgical trauma, improved cosmesis, and faster postoperative recovery. The final product that unfolds in the operating room reflects many hours of research, development, and focused trial and error in the simulation lab learning what works and equally important what does not.
The University of Rochester—LSI SOLUTIONS® (UR-LSI) Partnership
There are only a few models of scholarly, clinical exchange between academic medicine and industry in the United States (17-19) published in the literature (Table 1). The cardiac surgery research partnership between the University of Rochester and LSI SOLUTIONS® began in 2010, with the establishment of the Peter A. Knight M.D. Cardiac Surgery Research Fellowship Program. The overall goal was to create enabling technology and surgical techniques to develop a minimally invasive cardiac surgical program, and ultimately improve patient care. Since that time, a sophisticated cadaveric cardiac simulation lab has developed to test and evaluate devices, technologies, and exposures. Procedure specific course objectives of these labs have included minimally invasive aortic valve sparing root replacement, mitral valve repair, and left ventricular assist device insertion, among others. These cadaveric cardiac surgery simulation labs have been attended by cardiac surgeons and surgical trainees from across the country and internationally. In total, 37 cadaver labs, including 3 surgeon training events, using a total of 68 cadavers have occurred, with over 25 devices tested (Table 2). Ten of these devices have become available for clinical use in cardiac surgery. To date, 8 PAK fellows have matriculated, with the sum of their research resulting in 16 poster presentations, 23 podium presentations, and 14 published peer-reviewed manuscripts.
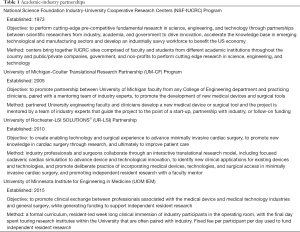
Full table
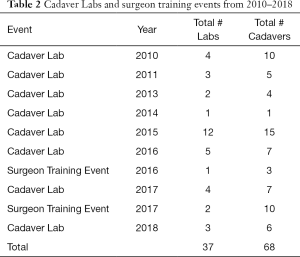
Full table
In conclusion, partnerships between surgery and industry play a vital role in advancing translational science in a more efficient manner, resulting in the expeditious conversion of medical device design concepts, new technologies, and new applications of existing technologies into tangible patient benefits with the goal of improved clinical outcomes. In addition, cadaveric simulation within this interactive translational research model is essential in optimizing the cooperative use of medical devices, technologies, and unfamiliar minimally invasive cardiac surgical approaches to promote the development of MICS. The University of Rochester and LSI SOLUTIONS® partnership provides a successful model of how interactive translational research and cadaveric simulation may be utilized to successfully create and develop a MICS program.
Acknowledgements
The authors would like to thank all those who so selflessly and most honorably choose whole body donation through the Anatomical Gift Program at the University of Rochester School of Medicine. Their gift makes the development of minimally invasive cardiac surgery possible.
Footnote
Conflicts of Interest: DA Robinson and CA Johnson Jr participate in a research fellowship that is supported in part by LSI SOLUTIONS®. The other authors have no conflicts of interest to declare.
References
- Reichenspurner H. The Evolution of Modern Cardiac Surgery and the Necessity to Change. Innovations (Phila) 2016;11:79-83. [Crossref] [PubMed]
- Gulbins H, Pritisanac A, Hannekum A. Minimally invasive heart valve surgery: already established in clinical routine? Expert Rev Cardiovasc Ther 2004;2:837-43. [Crossref] [PubMed]
- Piciche M, Dato GA, Lorusso R, et al. A Review of Evolutionary and Cyclical Changes in the Surgical Approach to Aortic Valve Disease. Rev Recent Clin Trials 2018;13:45-51. [Crossref] [PubMed]
- Easterwood RM, Bostock IC, Nammalwar S, et al. The evolution of minimally invasive cardiac surgery: from minimal access to transcatheter approaches. Future Cardiol 2018;14:75-87. [Crossref] [PubMed]
- Grossi EA, Galloway AC, Ribakove GH, et al. Impact of minimally invasive valvular heart surgery: a case-control study. Ann Thorac Surg 2001;71:807-10. [Crossref] [PubMed]
- Schmitto JD, Mokashi SA, Cohn LH. Minimally-invasive valve surgery. J Am Coll Cardiol 2010;56:455-62. [Crossref] [PubMed]
- Ott DA, Cooley DA, Solis RT, et al. Wound complications after median sternotomy: A study of 61 patients from a consecutive series of 9,279. Cardiovasc Dis 1980;7:104-11. [PubMed]
- Li AE, Fishman EK. Evaluation of complications after sternotomy using single- and multidetector CT with three-dimensional volume rendering. AJR Am J Roentgenol 2003;181:1065-70. [Crossref] [PubMed]
- Cosgrove DM 3rd, Sabik JF, Navia JL. Minimally invasive valve operations. Ann Thorac Surg 1998;65:1535-8; discussion 1538-9. [Crossref] [PubMed]
- Iribarne A, Easterwood R, Chan EY, et al. The golden age of minimally invasive cardiothoracic surgery: current and future perspectives. Future Cardiol 2011;7:333-46. [Crossref] [PubMed]
- Charles EJ, Kron IL. Bedside-to-Bench and Back Again: Surgeon-Initiated Translational Research. Ann Thorac Surg 2018;105:10-1. [Crossref] [PubMed]
- Baker CJ, Sinha R, Sullivan ME. Development of a cardiac surgery simulation curriculum: from needs assessment results to practical implementation. J Thorac Cardiovasc Surg 2012;144:7-16. [Crossref] [PubMed]
- Fann JI, Feins RH, Hicks GL Jr, et al. Evaluation of simulation training in cardiothoracic surgery: the Senior Tour perspective. J Thorac Cardiovasc Surg 2012;143:264-72. [Crossref] [PubMed]
- Ericsson KA. Acquisition and maintenance of medical expertise: a perspective from the expert-performance approach with deliberate practice. Acad Med 2015;90:1471-86. [Crossref] [PubMed]
- Danzer E, Dumon K, Kolb G, et al. What is the cost associated with the implementation and maintenance of an ACS/APDS-based surgical skills curriculum? J Surg Educ 2011;68:519-25. [Crossref] [PubMed]
- Dearani JA, Gold M, Leibovich BC, et al. The role of imaging, deliberate practice, structure, and improvisation in approaching surgical perfection. J Thorac Cardiovasc Surg 2017;154:1329-36. [Crossref] [PubMed]
- Skube SJ, Arsoniadis EG, Jahansouz C, et al. Supplementing Resident Research Funding Through a Partnership With Local Industry. J Surg Educ 2018;75:907-10. [Crossref] [PubMed]
- Pienta KJ. Successfully accelerating translational research at an academic medical center: the University of Michigan-Coulter translational research partnership program. Clin Transl Sci 2010;3:316-8. [Crossref] [PubMed]
- Industry-University cooperative research centers program (IUCRC). Available online: https://www.nsf.gov/funding/pgm_summ.jsp?pims_id=5501.