Current and future roles of mucins in cholangiocarcinoma—recent evidences for a possible interplay with bile acids
Introduction
Cholangiocarcinoma (CCA) is rare but often highly fatal tumour arising from the biliary tract epithelium. It encompasses three distinct anatomic categories, namely intrahepatic (iCCA), perihilar (pCCA) and distal (dCCA) which exhibit different clinical and pathological features.
All three types of CCA present diagnostic challenges. In particular, a presumed diagnosis of iCCA can be made on radiological criteria only in the absence of extrahepatic primary solid tumours and liver cirrhosis. Radiological criteria, in fact, are insensitive for the differentiation between scirrhous hepatocarcinoma (HCC) and iCCA, or metastatic adenocarcinoma and iCCA. In the latest case, the histological examination is useless as well and the only tool available remains the immunochemistry profile (1). Even the diagnosing of pCCA is challenging despite the availability of different imaging tools. Obtaining cells from pCCA for cytologic examination is remarkably difficult owing to the strong desmoplastic nature of this tumour. Therefore, the sensitivity of cytology performed on brush biopsy specimens is only 15% (2). Similarly, to pCCA, cancers located in the distal bile duct (dCCA) display low cellularity and strong desmoplastic reaction, thus making cytologic diagnosis difficult. Moreover, dCCA is characterized by a clinical presentation very similar to that of pancreatic adenocarcinoma. Thus, the differential diagnosis is challenging and a significant number of patients are candidate to surgical exploration without definitive reparative diagnosis (3).
Although surgery is the only curative option for both iCCA and extrahepatic CCA (eCCA), the type of treatment varies among these subtypes in terms of extent of liver and biliary resection. Unfortunately, due to the poorly specific nature of the symptoms, as well as to failure of currently available diagnostic tests, 70% of patients are diagnosed in advanced stage of disease, when they are no longer surgical candidates (4).
The pathogenesis of CCA is a complex, multi-step process, characterized by deregulation of various signalling networks. Despite several efforts made in the last few years for understanding CCA biology, the intricate network of molecular mechanisms responsible for early and widespread dissemination of this malignancy remains elusive. An enhanced understanding of CCA carcinogenesis and key molecular pathways would herald targeted, individualized therapies, as well as new early diagnostic tool with improvement of patient survival.
Recently, a family of high-molecular weight epithelial glycoproteins named mucin proteins, have gained interest for their involvement in tumour growth and progression and possible use as diagnostic and prognostic cancer biomarkers. Alterations in epithelial mucin core protein and glycosylation have been observed in a number of cancers and have been reported to be associated with cancer cells differentiation, proliferation, altered cellular adhesion, invasion and metastasis.
Along with mucin proteins also bile acids (BAs) represent a class of new promising biomarkers. Several studies demonstrated an association between BAs and some forms of liver and gastrointestinal cancer, mostly esophageal, stomach and colon cancers (5-7). BAs have been shown to participate in tumour progression by inducing alterations in the expression of oncogenic mucins (8,9). In particular, BAs have proven to modulate the expression levels of mucins such as MUC1, MUC2, MUC4 and MUC5AC in esophageal, gastric and colon cancers (9-12). In this review we will summarize the most important findings regarding the role of mucin glycoproteins and BAs in CCA and their possible use as diagnostic or prognostic biomarkers. We will also discuss a recent finding suggesting a possible interplay between BAs and mucins.
Mucins classification, structure and patho-physiological functions
Mucins represent the major structural constituents of mucus, a complex adherent secretion mainly involved in lubrication and epithelium protection from stress-induced damage. They consist in high-molecular weight glycoproteins constituted by many oligosaccharide side chains linked to a protein backbone called apomucin. They are characterized by the presence of tandem repeat domains mainly constituted by proline, threonine and serine (PST domain), which are extensively O-glycosylated through N-acetylgalactosamine O-linkages (GalNAcO-linkages) at the threonine and serine residues. The carbohydrate component represents about 50–80% of the total glycoprotein weight. To date, more than 20 human mucins have been identified. Based on their structure and function mucins are generally divided in two classes: membrane-bound mucins (i.e., MUC1, MUC3, MUC4, MUC12, MUC17) that are primarily located at the cell surface and secreted mucins that can be further subdivided into gel-forming mucins (MUC2, MUC5AC, MUC5B, MUC6, and MUC19) and non-gel-forming mucins (MUC7, MUC8 and OVGP1 or MUC9) (13). In addition to the PST domain, transmembrane mucins, except MUC4, are composed by a very conserved SEA (sea urchin sperm protein, enterokinase, and agrin) domain having a role in protein glycosylation and involved in the proteolytic cleavage leading to the mucin separation into two subunits. In the case of MUC1, after cleavage, both subunits (MUC1-C and MUC1-N) are noncovalently linked to form a heterodimer that blocks cell-cell and cell-extracellular matrix interactions. Changes in MUC1 promoting the release of the MUC1-N component, leaves MUC1-C as a putative receptor able to engage diverse signaling pathways linked to transformation and tumor progression (14,15). Secreted mucins undergo a similar proteolytic cleavage, but at a GPDH sequence. In addition, they are characterized by the presence of von Willebrand factor (VWF) domain and a cysteine-knot domain, responsible for dimerization in the endoplasmic reticulum. Mucins and mucin-associated carbohydrate chains have distinct tissue-specific distributions that can be quantitatively and qualitatively modified under pathological conditions (16). In this context, the glycosylated PST domain may be highly polymorphic for length and sequence variability and repeated multiple times.
Mucins play a fundamental role in homeostasis maintenance, a function exerted by both glycoproteins classes with some specificities. Secreted mucins primarily form the protective barrier, while membrane-bound mucins, in addition to contributing the physical barrier, transmit growth and survival signaling into the cell (13,15).
Aberrant expression of transmembrane mucins MUC1, MUC4, MUC13 and MUC16 has been associated with different human malignancies (13). It is their overexpression that usually contributes to oncogenesis by promoting loss of epithelial cell polarity, receptor tyrosine kinase signaling and constitutive activation of survival pathways, as the Wnt-β-catenin and nuclear factor-κB pathways. As an example, overexpression of MUC1 alters the function of the E-cadherin in cancer cells adherens junctions (17). Loss of tight junction function is mediated by the tyrosine kinase ERBB2 and is important to the epithelial stress response. MUC1 can forms complexes with ERBB2 promoting heregulin-induced ERBB2 signaling (13). Disruption of tight junctions may also be promoted by MUC4 via a different mechanism, but leading as well to the activation of downstream effectors of ERBB2 signaling. Therefore, overexpression of transmembrane mucins may promote the malignant epithelial-mesenchymal transition (EMT) phenotype by disrupting polarity and cell-cell interactions.
A role played by mucins in inflammation and cancer has also been recently established. Among the secreted mucins, loss of MUC2 was shown to confer a microenvironment in which bacteria may activate an inflammatory response at the epithelial cell surface (13). Indeed, physiological expression of MUC2 suppresses inflammation in the intestinal tract thereby inhibiting tumor development. A protective role against inflammation was also shown for MUC1. Nevertheless, in chronic inflammation prolonged activation of MUC1 can lead to cancer development as shown in Helicobacter pylori infection, in which MUC1 expression plays a major role in gastric cancer (18). The overall knowledge on cancers associated with mucin overexpression prompted the research field to develop inhibitors against mucin functions. One of the major targets is represented by MUC1-C subunit, whose inhibition has been shown to block the survival of human breast and prostate cancers (13).
Diagnostic and prognostic role of mucins
The most specific tumour-associated mucins found in biliary tract cancers are MUC4 and MUC5.
An altered gene expression of MUC4 in CCA has been widely proven in literature. Compared with samples from patients with benign biliary disease, MUC4 mRNA expression was consistently found upregulated in tissue samples, biliary brush samples and bile samples from patients with CCA. By using different molecular biology methods, the mean fold changes (cancer versus benign) of mRNA isolated from biliary brushings were as follow: 3.2 by microarray, 29.4 and 15.5 by quantitative PCR (qPCR) (19). Similarity, qPCR analysis on bile samples showed a 1.9-fold increase (95% CI: 1.69–2.33) in MUC4 mRNA expression in samples from CCA patients compared to samples from benign biliary conditions (95% CI: 0.86–1.16) (20). By using RNA isolated from tissue samples, the mean fold change of MUC4 expression was equal to 4.1 (19).
MUC4 overexpression has recently been suggested as potential predictor of poor outcome in patients with different types of cancer including CCA. To date, six studies have investigated the relationship between MUC4 expression and the overall survival by immunohistochemical staining of tissue samples (20-25). The main findings are reported in Table 1. All studies were retrospective in design and all but one was performed in Asian area. The immunohistochemistry was performed by using the 8G7 clone antibody except for Yeh et al. who used the 1G8 antibody and for He who used a polyclonal antibody. Overall, the result indicated that a positive or high expression levels of MUC4 was significantly related to poor survival in patients with resected CCA. Only Matull and coauthors (20) did not find a difference in survival rate between cancer patients with MUC4-positive and MUC4-negative disease. Moreover, in four out of six studies, high MUC4 expression was found to be statistically significant independent poor prognostic factor after adjustment for other clinicopathological factors including mainly surgical margin involvement and tumour size. A recent meta-analysis that pooled five out of the six studies reported here found a HR (hazard ratio) for positive or high expression group of 3.04 (95% CI: 2.25–4.12) when compared with negative or low expression group with slight between-study heterogeneities (I2=3.10%, P=0.39) (26).
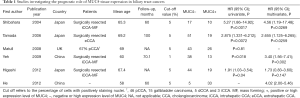
Full table
Analysis of MUC4 expression on bile samples has been also suggested as promising diagnostic biomarker for biliary tract cancers. Matull and coauthors reported that western blot analysis of biliary MUC4 has a sensitivity of 27% a specificity of 93% a positive and negative predictive value of 82% and 51% respectively in discriminating biliary tract cancers from other malignancies, primary sclerosing cholangitis and other benign biliary conditions (20).
MUC5AC, initially isolated as a tracheobronchial mucin (27) was demonstrated to be constitutively expressed not only in the bronchial epithelium but also in the gastric and endocervix epithelium, in bronchial submucosal glands and nasal mucosa, while it is not expressed in the normal intra-hepatic biliary tree. An aberrant expression of MUC5AC has been supposed to represent a new diagnostic and prognostic marker of CCA. To date, the association between serum MUC5AC status or concentration and overall survival has been assessed by three studies. Boonla and coauthors, by investigating the expression of MUC5AC in serum samples from 179 patients with histologically confirmed CCA by immunoblot analysis, showed that patients whit positive serum MUC5AC status (n=112) had a significantly poorer prognosis compared with patients with negative status (n=67) (median survival, 127 days; 95% CI: 107–180 vs. 329 days 95% CI: 199–458 days; P<0.001) (28). In multivariate analysis, after adjustment for predictive variables (including age, gender, histologic grade, tumor stage, and lymph node resection), a positive status for serum MUC5AC was associated with a 2.5-fold higher risk of death compared with a negative serum MUC5AC status (P<0.001). In the study of Matull et al., the median survival was 5.2 months (95% CI: 0.0–11.7 months) for biliary tract cancer (BTC) patients with MUC5AC positive status (n=17) compared with 16.9 months (95% CI: 9.8–23.9 months) for MUC5AC negative patients (n=22) (P=0.03) (20).
Finally, our group, who was the first to analyze the expression of MUC5AC in serum in a quantitative way demonstrated that patients with serum MUC5AC above the cut-off of 14 ng/mL had a survival rate at 3-year significantly lesser that patients with MUC5AC serum level behind the cut-off (21.5% vs. 59.3%, P=0.039) (29).
The performance of MUCAC5 as diagnostic biomarker has been assessed by a much higher number of studies. Two recent meta-analyses examined the potential of MUC5AC as biomarker in CCA. The first one, published in 2016 was made on six studies performed on serum samples including a total of 1,213 patients (20,29-33). The summary receiver’s operative characteristics (SROC) curve, used to summarize serum MUC5AC’s overall diagnostic performance showed an area under the curve (AUC) of 0.9138. The pooled sensitivity and specificity were 0.69 (95% CI: 0.65–0.73) and 0.93 (95% CI: 0.91–0.95) respectively with a heterogeneity index (I2) of 85.0% and 69.1% respectively. The pooled positive and negative likelihood ratio were 8.99 (95% CI: 5.65–14.30) and 0.33 (95% CI: 0.24–0.46) with I2 values of 53.0% and 83.6% (34). Subgroup analyses by laboratory method and ethnicity were performed to find out possible sources of heterogeneity although a significant decrease of I2 was not recorded. Therefore, the authors concluded that serum MUC5AC might be a useful tool for the diagnosis of CCA while, due to low negative likelihood ratio serum MUC5AC does not represent an ideal approach for CCA’s early detection.
The second meta-analysis, published in 2017, included a total of 17 studies six of which were performed on serum samples, ten on tissue samples and one on both matrices (35). With respect to previous mentioned meta-analysis, the latter included one more study in serum and used odds ratio (OR) estimates to assess the diagnostic performance of MUC5AC. Despite the different methodologies used, overall results were consistent among the two meta-analyses. The subgroup analysis on the seven studies conducted in serum samples showed an OR of 4.52 (95%CI:1.88–10.85, P=0.0007) thus confirming the potential of MUC5AC in the diagnosis of CCA. Major characteristics of these studies are reported in Table 2 (20,28,30-33). The high heterogeneity also found by these authors (I2=90%, P<0.0001) was mostly imputable to ethnicity as showed by the meta regression analysis performed in the whole population. Interesting, the sub-group analysis performed on tissue samples failed to find an association between MUC5AC and CCA (OR: 1.05, 95% CI: 0.43–2.59, P=0.92) thus highlighting the role of MUC5AC as circulating biomarker.
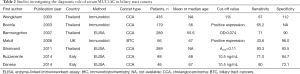
Full table
Emerging role of BAs in CCA
BAs are amphiphilic molecules and are the main component of bile along with cholesterol, phospholipids, and bilirubin (36). Primary BAs (cholic acid CA, chenodeoxycholic acid, CDCA) are synthesized in the liver using cholesterol as a precursor. After conjugation of carboxyl group with the amino acids glycine or taurine, they are secreted into the bile duct and in the duodenum. More than 95% of primary BAs are reabsorbed in the terminal ileum. In the colon, primary BAs are deconjugated and converted into “secondary BAs” (e.g., deoxycholic acid, DCA ursodeoxycholic acid UDCA, and lithocholic acid, LTA) by the bacterial flora. The secondary BAs DCA and UDCA are partly absorbed in the colon and enter the enterohepatic circulation where they also can be conjugated with glycine and taurine, to produce taurochenodeoxycholic acid (TCDCA) and glycochenodeoxycholic acid (GCDCA), whilst LTA is largely insoluble (37). Unfortunately, measuring BAs in biological fluids is not an easy enterprise and only few research groups have implemented reliable methods so far. To date, only six studies attempted to compare the composition of bile or serum BAs in CCA and BBD with often inconsistent results. Major characteristics and principal findings of these studies are summarized in Table 3 (38-43). Despite the differences in the methods used and matrices analysed, all studies but one found one or more BAs abnormally increased in CCA patients respect to healthy subjects or patients with BBD. Interesting, similar results came from studies on cancers different from CCA that arise in organs where a direct contact with bile (and thus BAs) can be established (i.e., liver and colon), as well as in those that enter in contact with in particular settings (i.e., oesophagus stomach and pancreas, as a consequence of reflux) (44).
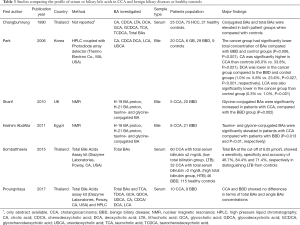
Full table
Recent studies have shown that conjugated BAs, but not free BAs, stimulate CCA cell growth, and that an imbalance in the ratio of free conjugated BAs may play an important role in the tumorigenesis of CCA. The mechanisms by which such BAs might exert their carcinogenic effects have not been elucidated, although evidence has recently emerged from the literature. First, the structure of BAs allows them to act as detergents, disrupting the lipid bi-layer of cells, thus potentially allowing carcinogenic substances to enter the cell. Other hypotheses include the ability of BAs to produce oxidative damage, epithelium proliferation, cell death, signaling activation and localized DNA instability. More recently, conjugated BAs have been shown to be able to stimulate CCA cell growth and invasion in vitro through the activation of the sphingosine 1-phosphate receptor 2 (S1PR2) and of the ERK1/2- and AKT-signaling pathways (45).
Interesting an increasing number of studies are showing that BAs may induce alterations in the expression of oncogenic mucins thus representing a new mechanism of tumour progression. Studies evaluating the possible interaction between BAs and mucin proteins in cancer development are presented in the follow paragraph (8,9). In particular, BAs are able to modulate the expression levels of mucins such as MUC1, MUC2, MUC4 and MUC5AC in esophageal, gastric and colon cancers (9-12).
Possible interplay between BAs and mucins
The earliest demonstration that BAs are able to induce alteration of mucin production dates back to 1996. In this pioneer study the authors observed that cytotoxic concentrations of BAs induce mucin release in human colonic epithelial cells, while nontoxic concentrations of DCA reduce mucin levels in differentiated enterocyte-like cells (46). These preliminary findings have prompted the design of further studies evaluating the possible cytoprotection or cytotoxicity of BAs-induced mucin alteration especially in the field of gastrointestinal and pancreatic cancer research. In 2004, Mariette and colleagues demonstrated that TCA, TDCA, TCDCA and GCA could induce MUC4 expression via activation of phosphatidylinositol 3-kinase in oesophageal cancer cell line (11). Through the same pathway, BAs were shown to induce MUC1 overexpression in human esophageal adenocarcinoma cells (8). In 2016 Joshi and coauthors demonstrated that the overexpression of MUC4 in pancreatic cancer, which is known to be associated with progression and metastasis, is induced by BAs via activation of the FXR/FAK/c-Jun axis (47). Conversely, in oesophageal cancer the MUC4 regulation is mediated by the activation of hepatocyte nuclear factor 1alpha by TDCA and TCDCA (9). Indeed, DCA was found to be able to modulate the expression of Cdx2 and the downstream MUC2 via the nuclear receptor FXR-NF-κB activity in normal gastric epithelial cells thus suggesting a possible implication of this pathway in the intestinal metaplasia of human gastric mucosa (48).
Taken together these findings suggest an emerging role of BAs-mucin proteins interaction in the pathogenesis of different type of cancers. To date, no studies have explored the possible interplay between BAs and MUC4 or MUC5AC in CCA although some promising data exist. El-Sayed Ali and coauthors recently performed a study aimed to analyse the possible effect of BAs on MUC5AC production by human colon carcinoma cells used as model for airway goblet cells. After incubation of the cell lines with different concentrations of physiological concentrations of CA, CDCA DCA and LCA, the authors observed a dose-dependent increase in MUC5AC production which occurred within the first 24 hours after challenge (49). Future studies aimed to investigate the association between CCA-linked aberrant signaling pathways and MUC5AC expression are needed in order to verify the possible involvement of BAs.
Conclusions
CCA incidence rates are increasing worldwide. Current diagnostic techniques to distinguish benign from malignant biliary tract disease are unsatisfactory, but evidence exist about the role of MUC4 and MUC5AC as reliable biomarkers of cancer progression. However, at this time, less is known about the molecules and the downstream intracellular signaling cascades that are responsible for the overexpression of MUC4 and MUC5AC gene observed in CCA. An increasing amount of data, however, suggests a possible involvement of BAs. Due to the increased interest in BAs chemistry, biology, metabolism and pathophysiology, the assessment of a comprehensive BAs profile in bile of patients with CCA and benign biliary diseases is hence advisable for better defining which BAs exert the higher carcinogenic potency, but also for identifying new potential disease markers whose measurement in plasma may help improving diagnosis and prognostication of CCA. Moreover, a more detailed understanding of the precise mechanisms by which BAs might induce MUC5AC or MUC4 expression could also facilitate the development of chemopreventive strategies to lower the risk of carcinogenesis and metastasis in mucinous carcinomas.
Acknowledgements
None.
Footnote
Conflicts of Interest: The authors have no conflicts of interest to declare.
References
- Bridgewater J, Galle PR, Khan SA, et al. Guidelines for the diagnosis and management of intrahepatic cholangiocarcinoma. J Hepatol 2014;60:1268-89. [Crossref] [PubMed]
- Razumilava N, Gores GJ. Classification, diagnosis, and management of cholangiocarcinoma. Clin Gastroenterol Hepatol 2013;11:13-21.e1; quiz e3-4.
- Dickson PV, Behrman SW. Distal cholangiocarcinoma. Surg Clin North Am 2014;94:325-42. [Crossref] [PubMed]
- Squadroni M, Tondulli L, Gatta G, et al. Cholangiocarcinoma. Crit Rev Oncol Hematol 2017;116:11-31. [Crossref] [PubMed]
- Bernstein H, Bernstein C, Payne CM, et al. Bile acids as carcinogens in human gastrointestinal cancers. Mutat Res 2005;589:47-65. [Crossref] [PubMed]
- Schwabe RF, Jobin C. The microbiome and cancer. Nat Rev Cancer 2013;13:800-12. [Crossref] [PubMed]
- Bernstein C, Holubec H, Bhattacharyya AK, et al. Carcinogenicity of deoxycholate, a secondary bile acid. Arch Toxicol 2011;85:863-71. [Crossref] [PubMed]
- Mariette C, Piessen G, Leteurtre E, et al. Activation of MUC1 mucin expression by bile acids in human esophageal adenocarcinomatous cells and tissues is mediated by the phosphatidylinositol 3-kinase. Surgery 2008;143:58-71. [Crossref] [PubMed]
- Piessen G, Jonckheere N, Vincent A, et al. Regulation of the human mucin MUC4 by taurodeoxycholic and taurochenodeoxycholic bile acids in oesophageal cancer cells is mediated by hepatocyte nuclear factor 1alpha. Biochem J 2007;402:81-91. [Crossref] [PubMed]
- Lee JY, Lee KT, Lee JK, et al. Farnesoid X receptor, overexpressed in pancreatic cancer with lymph node metastasis promotes cell migration and invasion. Br J Cancer 2011;104:1027-37. [Crossref] [PubMed]
- Mariette C, Perrais M, Leteurtre E, et al. Transcriptional regulation of human mucin MUC4 by bile acids in oesophageal cancer cells is promoter-dependent and involves activation of the phosphatidylinositol 3-kinase signalling pathway. Biochem J 2004;377:701-8. [Crossref] [PubMed]
- Martin NA, Mount Patrick SK, Estrada TE, et al. Active transport of bile acids decreases mucin 2 in neonatal ileum: implications for development of necrotizing enterocolitis. PLoS One 2011;6. [Crossref] [PubMed]
- Kufe DW. Mucins in cancer: function, prognosis and therapy. Cancer 2009;9:874-85. [PubMed]
- Kufe DW. Targeting the human MUC1 oncoprotein: a tale of two proteins. Cancer Biol Ther 2008;7:81-4. [Crossref] [PubMed]
- Dhanisha SS, Guruvayoorappan C, Drishya S, et al. Mucins: Structural diversity, biosynthesis, its role in pathogenesis and as possible therapeutic targets. Crit Rev Oncol Hematol 2018;122:98-122. [Crossref] [PubMed]
- Silva E, Teixeira A, David L. Mucins as key molecules for the classification of intestinal metaplasia of the stomach. Virchows Arch. 2002;440:311-7. [Crossref] [PubMed]
- Vajaria BN, Patel PS. Glycosylation: a hallmark of cancer? Glycoconj J 2017;34:147-56. [Crossref] [PubMed]
- Vinall LE, King M, Novelli M, et al. Altered expression and allelic association of the hypervariable membrane mucin MUC1 in Helicobacter pylori gastritis. Gastroenterology. 2002;123:41-9. [Crossref] [PubMed]
- Chapman MH, Tidswell R, Dooley JS, et al. Whole genome RNA expression profiling of endoscopic biliary brushings provides data suitable for biomarker discovery in cholangiocarcinoma. J Hepatol 2012;56:877-85. [Crossref] [PubMed]
- Matull WR, Andreola F, Loh A, et al. MUC4 and MUC5AC are highly specific tumour-associated mucins in biliary tract cancer. Br J Cancer 2008;98:1675-81. [Crossref] [PubMed]
- Tamada S, Shibahara H, Higashi M, et al. MUC4 is a novel prognostic factor of extrahepatic bile duct carcinoma. Clin Cancer Res 2006;12:4257-64. [Crossref] [PubMed]
- Yeh CN, Pang ST, Wu RC, et al. Prognostic value of MUC4 for mass-forming intrahepatic cholangiocarcinoma after hepatectomy. Oncol Rep 2009;21:49-56. [PubMed]
- Higashi M, Yamada N, Yokoyama S, et al. Pathobiological implications of MUC16/CA125 expression in intrahepatic cholangiocarcinoma-mass forming type. Pathobiology 2012;79:101-6. [Crossref] [PubMed]
- He Y. Expression and signification of MUC3 and MUC4 in hepatolithiasis associated intrahepatic cholangiocarcinoma. Hengyang: The University of South China, 2012.
- Shibahara H, Tamada S, Higashi M, et al. MUC4 is a novel prognostic factor of intrahepatic cholangiocarcinoma-mass forming type. Hepatology 2004;39:220-9. [Crossref] [PubMed]
- Li B, Tang H, Zhang A, Dong J. Prognostic Role of Mucin Antigen MUC4 for Cholangiocarcinoma: A Meta-Analysis. PLoS One 2016;11. [Crossref] [PubMed]
- Guyonnet Duperat V, Audie JP, Debailleul V, et al. Characterization of the human mucin gene MUC5AC: a consensus cysteine-rich domain for 11p15 mucin genes? Biochem J 1995;305:211-9. [Crossref] [PubMed]
- Boonla C, Wongkham S, Sheehan JK, et al. Prognostic value of serum MUC5AC mucin in patients with cholangiocarcinoma. Cancer 2003;98:1438-43. [Crossref] [PubMed]
- Ruzzenente A, Iacono C, Conci S, et al. A novel serum marker for biliary tract cancer: diagnostic and prognostic values of quantitative evaluation of serum mucin 5AC (MUC5AC). Surgery 2014;155:633-9. [Crossref] [PubMed]
- Silsirivanit A, Araki N, Wongkham C, et al. A novel serum carbohydrate marker on mucin 5AC: values for diagnostic and prognostic indicators for cholangiocarcinoma. Cancer 2011;117:3393-403. [Crossref] [PubMed]
- Wongkham S, Sheehan JK, Boonla C, et al. Serum MUC5AC mucin as a potential marker for cholangiocarcinoma. Cancer Lett 2003;195:93. [Crossref] [PubMed]
- Bamrungphon W, Prempracha N, Bunchu N, et al. A new mucin antibody/enzyme-linked lectin-sandwich assay of serum MUC5AC mucin for the diagnosis of cholangiocarcinoma. Cancer Lett 2007;247:301-8. [Crossref] [PubMed]
- Danese E, Ruzzenente O, Ruzzenente A, et al. Assessment of bile and serum mucin5AC in cholangiocarcinoma: diagnostic performance and biologic significance. Surgery 2014;156:1218-24. [Crossref] [PubMed]
- Xuan J, Li J, Zhou Z, et al. The diagnostic performance of serum MUC5AC for cholangiocarcinoma: A systematic review and meta-analysis. Medicine (Baltimore) 2016;95. [Crossref] [PubMed]
- Pabalan N, Sukcharoensin S, Butthongkomvong K, et al. Expression and Serum Levels of Mucin 5AC (MUC5AC) as a Biomarker for Cholangiocarcinoma: a Meta-analysis. J Gastrointest Cancer 2017. [Epub ahead of print]. [Crossref] [PubMed]
- Baptissart M, Vega A, Maqdasy S, et al. Bile acids: from digestion to cancers. Biochimie 2013;95:504-17. [Crossref] [PubMed]
- Griffiths WJ, Sjövall J. Bile acids: analysis in biological fluids and tissues. J Lipid Res 2010;51:23-41. [Crossref] [PubMed]
- Sharif AW, Williams HR, Lampejo T, et al. Metabolic profiling of bile in cholangiocarcinoma using in vitro magnetic resonance spectroscopy. HPB (Oxford) 2010;12:396-402. [Crossref] [PubMed]
- Hashim Abdalla MS, Taylor-Robinson SD, Sharif AW, et al. Differences in phosphatidylcholine and bile acids in bile from Egyptian and UK patients with and without cholangiocarcinoma. HPB (Oxford) 2011;13:385-90. [Crossref] [PubMed]
- Proungvitaya S, Sombattheera S, Boonsiri P, et al. Diagnostic value of serum bile acid composition patterns and serum glycocholic acid levels in cholangiocarcinoma. Oncol Lett 2017;14:4943-8. [Crossref] [PubMed]
- Changbumrung S, Tungtrongchitr R, Migasena P, et al. Serum unconjugated primary and secondary bile acids in patients with cholangiocarcinoma and hepatocellular carcinoma. J Med Assoc Thai 1990;73:81-90. [PubMed]
- Sombattheera S, Proungvitaya T, Limpaiboon T, et al. Total serum bile acid as a potential marker for the diagnosis of cholangiocarcinoma without jaundice. Asian Pac J Cancer Prev 2015;16:1367-70. [Crossref] [PubMed]
- Park JY, Park BK, Ko JS, et al. Bile acid analysis in biliary tract cancer. Yonsei Med J 2006;47:817-25. [Crossref] [PubMed]
- Di Ciaula A, Wang DQ, Molina-Molina E, et al. Bile Acids and Cancer: Direct and Environmental-Dependent Effects. Ann Hepatol 2017;16:s87-s105. [Crossref] [PubMed]
- Liu R, Zhao R, Zhou X, et al. Conjugated bile acids promote cholangiocarcinoma cell invasive growth through activation of sphingosine 1-phosphate receptor 2. Hepatology 2014;60:908-18. [Crossref] [PubMed]
- Shekels LL, Lyftogt CT, Ho SB. Bile acid-induced alterations of mucin production in differentiated human colon cancer cell lines. Int J Biochem Cell Biol 1996;28:193-201. [Crossref] [PubMed]
- Joshi S, Cruz E, Rachagani S, et al. Bile acids-mediated overexpression of MUC4 via FAK-dependent c-Jun activation in pancreatic cancer. Mol Oncol 2016;10:1063-77. [Crossref] [PubMed]
- Li S, Chen X, Zhou L, et al. Farnesoid X receptor signal is involved in deoxycholic acid-induced intestinal metaplasia of normal human gastric epithelial cells. Oncol Rep 2015;34:2674-82. [Crossref] [PubMed]
- Ali MS, Parikh S, Pearson JP. Bile Salts Up-Regulate MUC5AC, Not MUC5B, Mucin Expression in Cultured Airway Goblet Cell 006 Model HT29-MTX Cell Line. Glob J Oto 2017;7.