Protecting functional β cells with a therapeutic peptide
Type 1 diabetes (T1D) is an autoimmune disease in which insulin-producing pancreatic islet β cells are targeted and destroyed by autoreactive immune cells. This loss of β cell mass leads to decreased capacity for insulin production and an eventual decline of insulin-mediated regulation of glucose levels in the blood. As functional β cell mass is depleted, the remaining β cells are required to produce higher levels of insulin per β cell, leading to β cell exhaustion or apoptosis, both of which lead to further decreases in net β cell function (1). Eventually, too few functional β cells remain to secrete adequate levels of insulin to maintain normal blood glucose levels. Insufficient insulin secretion then leads to chronic hyperglycemia and T1D. T1D can be managed through injection of exogenous insulin to normalize blood glucose. However, no cure exists and insulin therapy leaves patients susceptible to hypoglycemic episodes and to the deleterious complications of periodic hyperglycemia including cardiovascular disease, blindness, and kidney failure (2).
Destruction of β cell mass has long been viewed as the predominant cause of T1D. This destruction is believed to occur gradually and as a result of chronic and prolonged autoimmune activity in the islet (3,4). The characteristic hyperglycemia and related symptoms associated with T1D are not thought to occur until approximately 80% of functional β cell mass is lost (5). However, several studies provide evidence that a significant proportion of subjects diagnosed with T1D have measurable levels of c-peptide, indicating a residual mass of functional β cells (5). Notably, some subjects retain measurable β cell functional for years after diagnosis. Furthermore, an even larger proportion of subjects with T1D (including subjects with no detectable c-peptide) secrete measurable levels of proinsulin, indicating the presence of significant β cell mass that is dysfunctional but not destroyed (6). As such, there is an increasing interest in more clearly defining the role that β cell dysfunction plays in the progression of T1D and, correspondingly, the importance of developing therapeutic strategies to restore β cell health and function. Indeed, there is an apparent window of opportunity between the initiation of autoimmune activity and the clinical onset of T1D for novel therapeutic interventions to rejuvenate and protect remaining β cell mass, thereby delaying T1D onset (7).
The primary role of β cells is secretion of insulin in response to increased blood glucose. Insulin is stored in secretory granules and is released by exocytosis. This release, then, requires the fusion of insulin-containing granules with the plasma membrane. The fusion of these membranes is accomplished by assembly of souble N-ethylmaleimide-sensitive factor-attachment protein receptor (SNARE) complexes. These membrane-bridging complexes are assembled from individual SNARE proteins anchored in the two membranes. In response to glucose sensing, two target membrane SNARE (t-SNARE) proteins on the plasma membrane, syntaxin and synaptosomal-associated protein 25 (SNAP25), bind with one vesicle-associated (v-SNARE) protein, vesicle-associated membrane protein (VAMP) (8). These interactions form a complete SNARE complex, facilitate fusion of the two membranes, and permit insulin secretion from the β cell (Figure 1). Proper assembly of these SNARE complexes requires accessory proteins, known as Munc18 proteins. Munc18 proteins bind the t-SNARE and v-SNARE proteins with high affinity (8), but must release their SNARE partners to allow for SNARE assembly (Figure 1). To accomplish this, double C2-domain protein β (Doc2b) competes with SNARE proteins (9,10) and binds to Munc18 proteins (11,12) (Figure 1). Therefore, Doc2b serves as a temporary docking platform for Munc18 proteins, releasing the t-SNARE and v-SNARE proteins for complex formation and facilitating the fusion of the plasma and granule membranes. Cumulatively, these interactions regulate first and second phase insulin secretion. Therefore, Doc2b would seem to be crucial for proper β cell function and insulin secretion. In a study published in April 2018 in Diabetes, Aslamy et al. explore the role that Doc2b plays in β cell dysfunction in T1D. Their work also demonstrates that modulating this pathway through expression of the C-terminal tandem C2 domains (C2A and C2B) of Doc2b is a therapeutic option for promoting insulin secretion and maintaining residual β cell mass in those at risk for T1D (13).
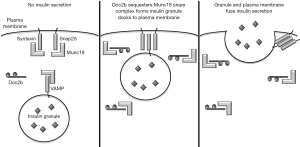
Previous work by these authors established a correlation between Doc2b expression and β cell function. Aslamy et al. showed that the remaining β cells in the islets of new-onset T1D patients exhibit reduced Doc2b expression (14). Furthermore, incubation of non-diabetic human islets with pro-inflammatory cytokines ex vivo, a condition intended to mimic the inflammatory milieu that destroys islets in vivo, reduced Doc2b expression (14). Conversely, overexpression of Doc2b in MIN6 insulinoma cells increased insulin secretion in vitro (10,15), and overexpression of Doc2b in the pancreas, skeletal muscle, and adipose tissue in vivo increased glucose-stimulated insulin secretion and improved whole-body glucose homeostasis and insulin sensitivity (16). Together, these studies suggest that modulation through Doc2b could be beneficial in maintaining β cell mass in the autoimmune prone individuals, but the mechanisms by which Doc2b promotes β cells health remained unknown.
In their new manuscript (13), Aslamy et al. confirm previous findings and explore more precisely the mechanisms by which Doc2b promotes β cell health and function. In this study, the authors first generated a doxycycline-induced β cell-specific double transgenic mouse model (βDoc2b-dTg). When these mice were exposed to doxycycline in their drinking water, Doc2b was overexpressed specifically in β cells by 4–5-fold compared to mice not given doxycycline (13). These doxycycline-treated mice exhibited higher insulin secretion and improved glucose tolerance than untreated mice during intraperitoneal glucose tolerance tests (IPGTT). Furthermore, when exposed to multiple-low-dose streptozotocin (MLD-STZ), Doc2b-overexpressing mice showed reduced β cell apoptosis, increased β cell mass, and increased serum insulin compared to control mice (13). Together, data confirmed prior observations in mice, establishing that Doc2b overexpression can be driven in multiple tissues (16). More importantly, these experiments demonstrated that overexpression of Doc2b exclusively in β cells increases insulin secretion and improves whole body glucose homeostasis (13).
To explore the anti-apoptotic effects of Doc2b in β cells, the authors transduced INS-1 insulinoma cells with adenovirus particles encoding Doc2b. When incubated with inflammatory cytokines, INS-1 cells overexpressing Doc2b showed reduced endoplasmic reticulum (ER) stress and apoptosis compared to control cells (13). These data confirm the observations made with the βDoc2b-dTg mice and demonstrate that overexpression of Doc2b improves β cell health and viability, even in the face of inflammatory insult (13). The authors then examined Doc2b proteins mutated by truncation of the Munc18-interacting domain (MID) domain or by single amino acid substitutions that obliterate calcium binding ability of C2A and C2B to determine the domain(s) most important for the protective effects of Doc2b. INS-1 cells were transfected with plasmids encoding these mutated proteins and first exposed to ER stress trigger Thapsigargin to test the efficacy of these mutated proteins in preventing apoptosis. Interestingly, the calcium-binding C2A and C2B were sufficient to protect INS-1 cells from apoptosis, since overexpression of a truncated protein containing only both C2 domains together (C2AB) reduced apoptosis to the same levels as the whole Doc2b protein. Importantly, this anti-apoptotic role was lost when the calcium-binding sites were disrupted by amino acid substitutions, demonstrating the importance of calcium for the protective effect (13).
Finally, the authors demonstrated that the C2AB is sufficient to improve β cell function and insulin secretion. Expression of C2AB, but not C2A or C2B separately, improved glucose-stimulated insulin secretion to similar levels as whole Doc2b in both MIN6 and INS-1 insulinoma cells. This supports previous work that the C2AB, but not C2A or C2B separately, create a ring-shaped oligomer that regulates membrane fusion (17,18). The authors also confirmed that C2AB was sufficient to activate the t-SNARE protein syntaxin 4, demonstrating that C2AB can facilitate the formation of complete SNARE complexes, and providing a mechanism to explain how these domains improve β cell insulin secretion (13).
The findings presented in this publication are exciting, since they reveal a promising new therapeutic avenue for promoting β cell function and preventing β cell apoptosis in those at risk for T1D. However, a few questions remain to be answered before these findings can be translated to the clinic. Given that calcium binding was essential for the anti-apoptotic activity of Doc2b, it would be of interest to further explore whether calcium binding to Doc2b directly modulates its activity or whether binding to C2AB depletes calcium levels, leading to reduced calcium-associated stress and enzymatic activity. C2AB facilitated SNARE complex formation and recapitulated many of the beneficial effects of Doc2b, but lacks the MID domain. Therefore, the functional role of that domain remains unclear. In neuronal studies, the C2A domain of Doc2b was suggested to interact directly with the plasma membrane in a Ca2+-dependent manner, promoting exocytosis by improving the kinetics of vesicle fusion (19). Consequently, there is some risk that Doc2b overexpression could lead to excessive insulin secretion in some scenarios. The authors propose that Doc2b sequesters Ca2+, preventing ER stress and down modulating the unfolded protein response (UPR). Indeed, several lines of evidence support a role for ER stress and intersection with the UPR to promote β cell dysfunction and apoptosis (20). However, the authors don’t show any data to directly support modulation of the UPR by Doc2b. Furthermore, experiments showing reduced ER stress and reduced apoptosis did not explore precisely how Doc2b reduces these processes or how its activity may influence enzymatic processes that were recently shown to directly modulate the immunogenicity of self-proteins (21). More mechanistic studies could shed additional light on how this protein interacts with these pathways (directly or indirectly) to protect β cells. Indeed, since overexpression or administration of Doc2b reduces apoptosis after inflammatory cytokine exposure, and given that β cells exhibit increased immunogenicity as a consequence of ER stress (21,22), Doc2b therapy may have the added benefit of reducing autoimmune targeting of β cells by autoreactive CD4+ and CD8+ T cells. Consequently, studies aimed at the effects of Doc2b overexpression on β cell immunogenicity could provide important insights.
One crucial consideration would be how best to apply Doc2b therapy as a clinical intervention. Systemic administration of C2AB as a peptide/protein therapeutic would likely be problematic, as off-target neurologic effects could be anticipated. Based on the work of Xiao et al., adenovirus gene therapy can be effectively targeted to the pancreas (23). However, barriers (most notably safety concerns) exist for clinical application of adenovirus based approaches. A more plausible option might be to utilize a DNA vaccine, as effectively implemented by Roep et al. for plasmid encoded proinsulin (24). In any case, it seems likely that driving expression of C2AB within the islets of new onset patients or subjects at risk for T1D could provide protection from apoptosis and enhance β cell function.
In summary, this interesting article highlights a role for Doc2b interactions with SNARE complexes to block apoptosis and rejuvenate insulin secretion by pancreatic β cells. Although further questions remain about the mechanisms of these effects, refinement of therapeutic strategies to harness these protective effects could be a major step forward toward effective treatment and prevention of T1D.
Acknowledgements
Funding: This work was supported in part by Children’s Hospital of Pittsburgh of the UPMC Health System [Cochrane-Weber Endowed Fund to ML Marré], National Institutes of Health [grant number 5R01DK099257-03 to JD Piganelli]; Juvenile Diabetes Research Foundation [grant number 1-SRA-2017-344-S-B and 2-SRA-2018-551-S-B to EA James]; and American Diabetes Association [grant number 1-17-IBS-129 to JD Piganelli].
Footnote
Conflicts of Interest: The authors have no conflicts of interest to declare.
References
- Akirav E, Kushner JA, Herold KC. Beta-cell mass and type 1 diabetes: going, going, gone? Diabetes 2008;57:2883-8. [Crossref] [PubMed]
- Burrack AL, Martinov T, Fife BT. T. Cell-Mediated Beta Cell Destruction: Autoimmunity and Alloimmunity in the Context of Type 1 Diabetes. Front Endocrinol (Lausanne) 2017;8:343. [Crossref] [PubMed]
- Eizirik DL, Mandrup-Poulsen T. A choice of death--the signal-transduction of immune-mediated beta-cell apoptosis. Diabetologia 2001;44:2115-33. [Crossref] [PubMed]
- Cnop M, Welsh N, Jonas JC, et al. Mechanisms of pancreatic beta-cell death in type 1 and type 2 diabetes: many differences, few similarities. Diabetes 2005;54 Suppl 2:S97-107. [Crossref] [PubMed]
- Chen C, Cohrs CM, Stertmann J, et al. Human beta cell mass and function in diabetes: Recent advances in knowledge and technologies to understand disease pathogenesis. Mol Metab 2017;6:943-57. [Crossref] [PubMed]
- Steenkamp DW, Cacicedo JM, Sahin-Efe A, et al. Preserved Proinsulin Secretion in Long-Standing Type 1 Diabetes. Endocr Pract 2017;23:1387-93. [Crossref] [PubMed]
- Battaglia M, Anderson MS, Buckner JH, et al. Understanding and preventing type 1 diabetes through the unique working model of TrialNet. Diabetologia 2017;60:2139-47. [Crossref] [PubMed]
- Rizo J, Sudhof TC. The membrane fusion enigma: SNAREs, Sec1/Munc18 proteins, and their accomplices--guilty as charged? Annu Rev Cell Dev Biol 2012;28:279-308. [Crossref] [PubMed]
- Verhage M, de Vries KJ, Roshol H, et al. DOC2 proteins in rat brain: complementary distribution and proposed function as vesicular adapter proteins in early stages of secretion. Neuron 1997;18:453-61. [Crossref] [PubMed]
- Ke B, Oh E, Thurmond DC. Doc2beta is a novel Munc18c-interacting partner and positive effector of syntaxin 4-mediated exocytosis. J Biol Chem 2007;282:21786-97. [Crossref] [PubMed]
- Oh E, Kalwat MA, Kim MJ, et al. Munc18-1 regulates first-phase insulin release by promoting granule docking to multiple syntaxin isoforms. J Biol Chem 2012;287:25821-33. [Crossref] [PubMed]
- Jewell JL, Oh E, Bennett SM, et al. The tyrosine phosphorylation of Munc18c induces a switch in binding specificity from syntaxin 4 to Doc2beta. J Biol Chem 2008;283:21734-46. [Crossref] [PubMed]
- Aslamy A, Oh E, Olson EM, et al. Doc2b Protects β-Cells Against Inflammatory Damage and Enhances Function. Diabetes 2018;67:1332-44. [Crossref] [PubMed]
- Aslamy A, Oh E, Ahn M, et al. Exocytosis Protein DOC2B as a Biomarker of Type 1 Diabetes. J Clin Endocrinol Metab 2018;103:1966-76. [Crossref] [PubMed]
- Miyazaki M, Emoto M, Fukuda N, et al. DOC2b is a SNARE regulator of glucose-stimulated delayed insulin secretion. Biochem Biophys Res Commun 2009;384:461-5. [Crossref] [PubMed]
- Ramalingam L, Oh E, Thurmond DC. Doc2b enrichment enhances glucose homeostasis in mice via potentiation of insulin secretion and peripheral insulin sensitivity. Diabetologia 2014;57:1476-84. [Crossref] [PubMed]
- Zanetti MN, Bello OD, Wang J, et al. Ring-like oligomers of Synaptotagmins and related C2 domain proteins. Elife 2016.5. [PubMed]
- Brouwer I, Giniatullina A, Laurens N, et al. Direct quantitative detection of Doc2b-induced hemifusion in optically trapped membranes. Nat Commun 2015;6:8387. [Crossref] [PubMed]
- Friedrich R, Groffen AJ, Connell E, et al. DOC2B acts as a calcium switch and enhances vesicle fusion. J Neurosci 2008;28:6794-806. [Crossref] [PubMed]
- Brozzi F, Eizirik DL. ER stress and the decline and fall of pancreatic beta cells in type 1 diabetes. Ups J Med Sci 2016;121:133-9. [Crossref] [PubMed]
- Marré ML, McGinty JW, Chow I, et al. Modifying enzymes are elicited by ER stress, generating epitopes that are selectively recognized by CD4+ T cells in patients with type 1 diabetes. Diabetes 2018;67:1356-68. [Crossref] [PubMed]
- Marré ML, Profozich JL, Coneybeer JT, et al. Inherent ER stress in pancreatic islet β cells causes self-recognition by autoreactive T cells in type 1 diabetes. J Autoimmun 2016;72:33-46. [Crossref] [PubMed]
- Xiao X, Guo P, Shiota C, et al. Endogenous Reprogramming of Alpha Cells into Beta Cells, Induced by Viral Gene Therapy, Reverses Autoimmune Diabetes. Cell Stem Cell 2018;22:78-90.e4. [Crossref] [PubMed]
- Roep BO, Solvason N, Gottlieb PA, et al. Plasmid-encoded proinsulin preserves C-peptide while specifically reducing proinsulin-specific CD8(+) T cells in type 1 diabetes. Sci Transl Med 2013;5. [Crossref] [PubMed]