Should we titrate ventilation based on driving pressure? Maybe not in the way we would expect
Introduction
Optimization of invasive mechanical ventilation is mandatory to maintain adequate respiratory function in patients undergoing general anaesthesia (1), as well as in critically ill patients without (2,3) and with acute respiratory distress syndrome (ARDS) (4). Further, different types of mechanical ventilation have been proposed, from fully controlled to partially assisted and spontaneous breathing (5,6). Additionally, non-invasive mechanical ventilation has been also proposed in the early or later phases of respiratory support (7). However, mechanical ventilation may induce lung injury even when used for a limited period of time (1). Several parameters have been considered to guide mechanical ventilation strategies, taking into consideration the mechanical properties of the respiratory system, lung and chest wall, as well as gas-exchange and haemodynamic. During controlled mechanical ventilation, some ventilator parameters are set by the physician, such as tidal volume (VT), positive end-expiratory pressure (PEEP), respiratory rate (RR), inspiratory airflow (V’) and inspiratory oxygen fraction (8). Other ventilator parameters are derived by the interaction between those set by the physician and the mechanical characteristics of the respiratory, lung and chest wall, like the inspiratory peak (Ppeak) and plateau (Pplat) pressure. The inspiratory effort, like inspiratory drive, inspiratory pressure time product or work of breathing, as well as electrical activity of the diaphragm have been additionally considered during assisted or non-invasive ventilation modes of ventilation (9). More recently, driving pressure (∆P), which is the difference between the static airway or transpulmonary pressure at the end-inspiration and at the end-expiration, both during controlled or assisted ventilation, has been proposed as an additional and more useful parameter to set mechanical ventilation. In this review, we will discuss the role of ∆P to optimize respiratory support both in patients during surgery as well as in critically ill patients with and without ARDS, during controlled and assisted ventilation.
Measurement of driving pressure
The following paragraphs will describe practical methods to measure the ∆P in different clinical settings.
Controlled mechanical ventilation
During controlled mechanical, the driving pressure of the respiratory system (ΔPrs) is defined as the difference between the plateau pressure of the airways at end-inspiration (PPLAT,rs) and PEEP. If the transpulmonary pressure is considered, the transpulmonary driving pressure (ΔPL) is calculated as ΔPL = (PPLAT,rs – POES,end-insp) – (PEEP − POES,end-exp) where POES,end-insp is the oesophageal pressure at end inspiration and POES,end-exp is the oesophageal pressure at end-expiration. The ΔPrs and ΔPL represent the distending pressure received by the respiratory system or the lung, respectively, when VT is delivered. In other words, ΔPrs = VT/compliance of the respiratory system (Cst,rs) or ΔPL = VT/compliance of the lung (Cst,L). From a theoretical point of view, if the Cst,rs or Cst,L, are linked to the aeration of the lungs at end-expiration, ΔP is a simple parameter at the bedside evaluating the distension of the respiratory system or the lungs induced by VT standardized by the pulmonary aeration, namely a surrogate of the dynamic lung strain. As an example (see Figure 1), in a patient ventilated with a VT of 500 mL, with an aerated lung volume at end-expiration of 2,000 mL and the Cst,rs of 100 mL/cmH2O, the relative ΔPrs will be 5 cmH2O. On the other hand, in a patient ventilated with a VT of 500 mL, with an aerated lung volume at end-expiration of 500 mL and the Cst,rs of 25 mL/cmH2O, the relative ΔPrs will be 20 cmH2O. This means that the VT delivered to lungs with a relevant loss of aeration induces greater distending pressure to the respiratory system and the lungs compared to the same VT applied to well aerated lungs. The consequence is that, to apply a low distending pressure in presence of loss of aeration, a reduction in VT will be necessary.
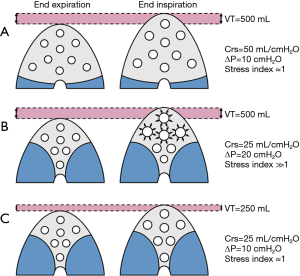
As shown in Figure 2, ΔP may be affected by: (I) VT size, i.e., the volume difference between end-inspiration and end-expiration; (II) PEEP, increasing the aerated lung size at end-expiration, thus improving Cst,rs and/or Cst,L. If PEEP is too high, yielding overdistension, or too low, yielding collapse, ΔP will further increase. Thus, the application of PEEP influences ΔP, depending on delivered VT. In experimental (10) and clinical (11) studies, it has been shown that different VT may deeply affect the aerated lung at end-expiration. In other words, higher VT, and consequently, higher Pplat, yield higher lung volume at end-expiration, in a dynamic recruitment process. Thus, ∆P should be cautiously interpreted if, during inspiration, VT progressively recruits or over-distends aerated or previously partially collapsed lung regions (12). Thus, VT may differently affect ΔP in presence of intra-tidal collapse and de-collapse and/or excessive overdistension. The combined measurement with stress index might improve the correct interpretation of ΔP. If at higher VT or PEEP, ΔP increases as well as stress index, this suggests overinflation of the lung; on the other side if ΔP increases but stress index decreases this likely mean that overinflation is associated with progressive recruitment and de-recruitment of alveolar units. Other factors may affect the correct measurement and interpretation of ΔP, such as the duration of the inspiratory pause/hold used to measure Pplat and the correct evaluation of PEEP. In most of the current studies, Pplat was measured 0.5 s after onset of the end-inspiratory pause and the PEEP used was the ventilator setting instead of the measured total PEEP. A recent study (13) showed that ΔP values are significantly altered by the manner in which Pplat and PEEP are measured: the measurement of ΔP is feasible during controlled mechanical ventilation, but its correct evaluation is not always easy to perform at the bedside with commonly used monitoring systems.
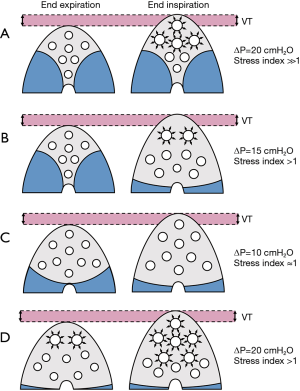
Assisted or non-invasive mechanical ventilation
It is commonly believed that the estimation of Pplat (and hence ΔP) is unreliable during assisted ventilation or spontaneous effort, since a sufficiently stable inspiratory hold cannot be achieved (14). Further, one must consider that the contribution of spontaneous effort cannot be estimated without the additional use of oesophageal pressure measurement. For these reasons, Pplat and ΔP are often thought to be accurately measurable only during controlled mechanical ventilation, and even paralysis, but not in presence of spontaneous effort. However, it has been proposed the possibility to estimate the ΔP even during assisted and spontaneous breathing (15). This might be performed during pressure support ventilation, or other modes of pressure limited ventilation, if the ventilator allows to perform an inspiratory hold in assisted modes, which is not always the case. A brief inspiratory hold is maintained at end of inspiration and may result in the measurement of a reasonably accurate Pplat. The airway pressure waveform does not reflect the inspiratory effort (negative Pes deflection), except when the inspiration is interrupted. An inspiratory hold during a positive pressure breath without spontaneous effort results in a slight decrease in the inspiratory pressure as the flow reduces to zero yielding the Pplat; this is the distending inspiratory pressure generated by the application of VT. On the other hand, an inspiratory hold during a positive pressure breath with spontaneous effort results in an increase in the airway pressure, which is again the Pplat. In this case the difference between the level of inspiratory support at end-inspiration and Pplat reflect both the size of the VT and the additional (hidden) contribution of the spontaneous effort at the end of inspiration (16). However, in case of active inspiratory effort, the ΔP of the respiratory system will underestimate the real ΔP of the lung, which includes the maximal inspiratory negative swing of the oesophageal pressure. In conclusion, an inspiratory hold during assisted ventilation allows measurement of the total distending pressure, i.e., Pplat and derivation of compliance as well as ΔP, which might allow a better setting of protective ventilation during spontaneous breathing. However, this measurement appears to be valid only in presence of low to moderate inspiratory effort.
Driving pressure during anaesthesia
Protective mechanical ventilation strategies using low VT with low to moderate levels of PEEP have been shown to improve outcomes and reduce post-operative pulmonary complications for patients who have had surgery (8,17,18), and efforts have been made to understand whether the use of individualized ventilator settings can improve outcome (19). However, in this clinical setting, the role of ΔP is debated. More recently, the optimization of ΔP has been considered as a tool to optimize mechanical ventilation during surgery in an attempt to minimize post-operative pulmonary complications and improve outcome. In a retrospective hospital registry based study in 69,265 consecutively enrolled patients who underwent a non-cardiac surgical procedures, the use of intraoperative protective ventilation, with VT less than 10 mL/kg predicted body weight and PEEP equal or higher than 5 cmH2O was associated with a decreased risk of post-operative respiratory complications (20). In this study, a PEEP of 5 cmH2O and a plateau pressure of 16 cmH2O or less were associated with the lowest risk of postoperative pulmonary complications. Interestingly, ΔP higher than 16 cmH2O was associated with an increased risk to develop post-operative pulmonary complications. In another retrospective study, including 109,360 adults undergoing mechanical ventilation during surgery, post-operative pulmonary complications were found to be more common after pressure-controlled ventilation compared to volume-controlled ventilation. VT and ΔP were varied more frequently with pressure-controlled ventilation compared with volume-controlled ventilation independently from the level of ΔP (i.e., higher or lower than 19 cmH2O) or PEEP (higher or lower than 5 cmH2O). These data seem to support the use of volume-controlled ventilation during surgery, particularly for patients at higher risk to develop post-operative pulmonary complications (21). These studies were based on the analysis of administrative data of large cohorts of patients. These findings were confirmed from individual data meta-analysis from randomized controlled trials in 2,250 patients undergoing non-cardiac surgery (22). Multivariate analysis suggested that ΔP was proportionally associated (i.e., without any specific threshold) with the development of post-operative pulmonary complications. Further, in a mediation analysis, ΔP was the only significant mediator of the effects of protective ventilation on development of pulmonary complications after surgery. Thus, findings from a retrospective, prospective observational and individual data meta-analysis from randomized controlled trials suggest an association between ΔP during surgery and the risk to develop post-operative pulmonary complications. The main drawback of these association analyses is that they are unable to define a causative link between ΔP and the occurrence of respiratory complications: the main clinical question remains whether the manipulation of ΔP during mechanical ventilation in patients undergoing surgery can affect post-operative outcome. Two mechanical ventilation strategies may be used to reduce ΔP: (I) use of lower VT; (II) titrating PEEP in order to minimize ΔP, at a constant VT. The reduction in VT and consequently ΔP has been found the main strategy to reduce the risk of developing post-operative pulmonary and/or non-pulmonary complications after surgery (8,17). Individual randomized controlled trials adequately powered have not been performed to directly test the hypothesis that lower VT alone is directly influencing post-operative outcome. However, studies published so far do not suggest any potential benefit of higher VT during surgery on post-operative outcome. Two randomized controlled trials investigated the effect of PEEP, at constant protective VT, during surgery on post-operative outcome (pulmonary and/or extrapulmonary complications) (23,24). In 900 patients at risk for postoperative pulmonary complications who were planned for open abdominal surgery under general anaesthesia ventilated at VT of 8 mL/kg, the application of PEEP 12 cmH2O with recruitment manoeuvres compared to PEEP 2 cmH2O without recruitment manoeuvres significantly improved the compliance of the respiratory system, thus reducing ΔP, without affecting post-operative pulmonary complications (23). Further, PEEP 12 cmH2O was associated with an increased post-operative plasma levels of Clara cell protein 16 (CC-16), a marker of epithelial lung injury (25) suggesting pulmonary overdistension (26). Another randomized controlled trial investigated 1,012 patients scheduled to have abdominal surgery with an expected duration above 2 h, ventilated with low VT and a PEEP either set to a fixed value of 5 cmH2O or individually set to achieve the lowest driving pressure (24). Individually tailored PEEP resulted in lower driving pressure (10.4 versus 13.2 cmH2O), without determining changes in post-operative pulmonary and systemic complications during the first 7 days after surgery. Overall, these data suggest that the reduction in ΔP determined by PEEP-induced recruitment, even when individually titrated, was not effective to reduce post-operative complications and improve outcome after surgery during ventilation with protective VT. These findings are in line with those reported previously, showing no beneficial effect of reduction in ΔP on the incidence of post-operative pulmonary complications (22). On the other side, levels of PEEP associated with an increase in ΔP, during protective VT, might be associated with an increased risk of post-operative pulmonary complications (22). However, several authors have started to challenge the concept that the lung should be kept open to minimise lung injury (27,28). Moreover, physiologic studies question the reliability of ΔP to assess the dynamic strain when relevant PEEP-induced lung aeration is present (29). All these studies excluded obese patients with body mass index higher than 35 kg/m2. A large randomized controlled trial has been recently completed, investigating the effects of higher PEEP (12 cmH2O) with recruitment manoeuvre compared to lower PEEP (4 cmH2O) without recruitment manoeuvre in obese patients with Body Mass Index higher than 35 kg/m2 and undergoing surgery (30). In this study, it is expected a marked reduction in ΔP with higher PEEP, thus more information about relationships between ΔP and post-operative complications will be available soon in obesity. Future studies could better explore the potential beneficial effects of individually titrated PEEP, not to the minimal ΔP, but to the maximal level of PEEP to avoid further increase in ΔP, and thus overdistension.
In conclusion, higher ΔP during surgery was associated with an increased risk to develop pulmonary and/or systemic complications in the post-operative period. The reduction in VT is the easiest and cheapest way to reduce ΔP potentially improving post-operative outcome. From randomized controlled trials and individual data meta-analysis, the application of PEEP to minimize ΔP, at constant protective VT, does not seem to be associated with a reduction in post-operative complications.
Driving pressure in non-ARDS critically ill patients
Many patients admitted to the intensive care unit requiring invasive mechanical ventilation do not have ARDS (31). A recent study found that patients without ARDS are characterized by higher in-ICU and in-hospital mortality than expected (32). On average roughly 50% of patients without ARDS received a VT higher than 8 mL/kg predicted body weight, and 10% to 20% of them were ventilated with a ΔP higher than 15 cmH2O, mainly in those at risk of ARDS. However, in this study neither a difference in ΔP between patients at risk of ARDS and those not at risk, nor an association between higher ΔP and mortality was found. In a further analysis, only higher peak pressure, but not ΔP or other ventilation variables, were independently associated with higher in-hospital mortality (33). This finding was reproduced in another retrospective analysis which included a cohort of 622 ventilated adult patients without ARDS on day 1 of mechanical ventilation, where ΔP was not associated with hospital mortality in whom compliance was also not associated with mortality (34). There are several possible explanations for the lack of association between ΔP and hospital mortality in patients without ARDS. First, ΔP is a way of representing the VT adjusted for the compliance and can be calculated from these parameters. There is actually no evidence that compliance is a major risk factor for mortality in patients without ARDS; second, most of the patients were receiving assisted ventilation and spontaneous breathing. During an assisted breath, the patient generates different amount of inspiratory efforts and the measured Pplat may be a poor surrogate for the static pressure at end-inspiration of the respiratory system or the lung; moreover, data were derived from a retrospective analysis of large administrative database with all the limitations of this study design. Another study investigating different mechanical ventilation settings and their associations with outcome in patients with and without ARDS is actually ongoing in several intensive care units in Asia (35). This study will provide information about the role of ΔP in middle-income countries. Additionally, a randomized controlled trial is ongoing evaluating the potential effects of restricted versus liberal PEEP, and consequently ΔP, in non-ARDS patients (36). On the other side, a lower VT has been reported in observational trials to be associated with a reduction in respiratory function worsening and improved outcome (37,38). A recent randomized controlled trial (39) has been completed and compared the effects of a low VT strategy (4 to 6 mL/kg predicted body weight) versus intermediate VT (8 to 10 mL/kg predicted body weight) on the number of ventilator-free days and alive at day 28. This study will give new valuable information about the role of VT and ΔP titration on morbidity and outcome in non-ARDS patients.
Driving pressure in ARDS patients
The role of ΔP in ARDS, as a main parameter associated with mortality or used to optimize PEEP and mechanical ventilation, is controversial. A recent post hoc analysis of individual data from patients enrolled in randomised trials, suggested that ΔP may be more important than traditional ventilatory variables in predicting outcome in mechanically ventilated patients with ARDS (40). Further, it was found an association between the magnitude of ΔP variation in response to PEEP and mortality. When PEEP increase resulted in an increased ΔP, mortality increased, when PEEP was associated with no changes in ΔP, mortality did not change, whereas when PEEP was associated with a decrease of ΔP, mortality was lower. The effect of ΔP was even more important than VT or Pplat to determine mortality. However, it should be noted that all the studies included in the analysis limited the level of Pplat at levels below a safety margin of 35 cmH2O. Further, studies using different VT were included in this analysis, making difficult to separate the individual effects of VT and PEEP on outcome. In an attempt to eliminate this confounding factor, ΔP was evaluated only in two ARDS trials in which low VT ventilation was strictly applied in both arms (41). When ventilating patients with low VT, ΔPrs was a risk factor for death in ARDS patients, as it was Pplat or Cst,rs. However, these data originated from trials from which most ARDS patients were excluded due to strict inclusion and exclusion criteria, and thus these findings should be also validated in independent observational studies in patients ventilated with a lung protective strategy. Another study (42) investigated the possible influence of the chest wall compliance on the effects of ΔP of the respiratory system on optimal PEEP setting and mortality. Using PEEP titration to target positive transpulmonary pressure via oesophageal manometry caused both improved compliance and lower ΔP, and strategies leading to decreased respiratory system and transpulmonary ΔP at 24 h were associated with improved 28-day mortality. A retrospective single-centre study (43) found that ΔP was not associated with mortality in obese ARDS patients contrary to non-obese ARDS patients. In a series of ARDS patients receiving extracorporeal membrane oxygenation (ECMO) for refractory hypoxemia, showed that ΔP during ECMO was the only ventilator setting that showed an independent association with in-hospital mortality (44). A recent metanalysis, confirmed an association between higher ΔP and mortality in mechanically ventilated patients with ARDS, with similar ΔP cut-offs (13–15 cmH2O) (45). However, in severe ARDS patients ventilated with protective VT, setting PEEP targeted on minimal ΔP with recruitment manoeuvres resulted in higher mortality in one trial (46), thus not supporting the routine use of this ventilation strategy in patients with ARDS (47). This observation is confirmed by the fact that Pplat is even better associated with mortality than ΔP (48). In Table 1 are shown changes in ΔP, at constant protective VT, reported in randomized controlled trials, investigating the effects of higher versus lower PEEP on mortality in ARDS. No association between changes in ΔP induced by PEEP and mortality was observed.
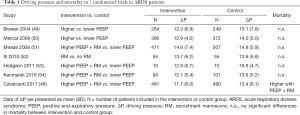
Full table
Conclusions
Optimization of mechanical ventilation is important to minimize ventilator-induced lung injury and improve outcome. ΔP is an indicator of severity of the lung disease, mainly related to VT size and associated with complications and mortality. We recommend the use of ΔP to optimize VT but not PEEP during surgery, and in critically ill patients with and without ARDS. Further information is needed about the possible use of ΔP to optimize ventilator setting during assisted mechanical ventilation.
Acknowledgements
None.
Footnote
Conflicts of Interest: The authors have no conflicts of interest to declare.
References
- Ball L, Costantino F, Orefice G, et al. Intraoperative mechanical ventilation: state of the art. Minerva Anestesiol 2017;83:1075-88. [PubMed]
- Serpa Neto A, Nagtzaam L, Schultz MJ. Ventilation with lower tidal volumes for critically ill patients without the acute respiratory distress syndrome: a systematic translational review and meta-analysis. Curr Opin Crit Care 2014;20:25-32. [Crossref] [PubMed]
- Sutherasan Y, Vargas M, Pelosi P. Protective mechanical ventilation in the non-injured lung: review and meta-analysis. Crit Care 2014;18:211. [Crossref] [PubMed]
- Fan E, Del Sorbo L, Goligher EC, et al. An Official American Thoracic Society/European Society of Intensive Care Medicine/Society of Critical Care Medicine Clinical Practice Guideline: Mechanical Ventilation in Adult Patients with Acute Respiratory Distress Syndrome. Am J Respir Crit Care Med 2017;195:1253-63. [Crossref] [PubMed]
- Güldner A, Pelosi P, Gama de Abreu M. Spontaneous breathing in mild and moderate versus severe acute respiratory distress syndrome. Curr Opin Crit Care 2014;20:69-76. [Crossref] [PubMed]
- Saddy F, Sutherasan Y, Rocco PR, et al. Ventilator-associated lung injury during assisted mechanical ventilation. Semin Respir Crit Care Med 2014;35:409-17. [Crossref] [PubMed]
- Esquinas AM, Benhamou MO, Glossop AJ, Mina B. Noninvasive Mechanical Ventilation in Acute Ventilatory Failure: Rationale and Current Applications. Sleep Med Clin 2017;12:597-606. [Crossref] [PubMed]
- Güldner A, Kiss T, Serpa Neto A, et al. Intraoperative protective mechanical ventilation for prevention of postoperative pulmonary complications: a comprehensive review of the role of tidal volume, positive end-expiratory pressure, and lung recruitment maneuvers. Anesthesiology 2015;123:692-713. [Crossref] [PubMed]
- Mauri T, Yoshida T, Bellani G, et al. Esophageal and transpulmonary pressure in the clinical setting: meaning, usefulness and perspectives. Intensive Care Med 2016;42:1360-73. [Crossref] [PubMed]
- Pelosi P, Goldner M, McKibben A, et al. Recruitment and derecruitment during acute respiratory failure: an experimental study. Am J Respir Crit Care Med 2001;164:122-30. [Crossref] [PubMed]
- Crotti S, Mascheroni D, Caironi P, et al. Recruitment and derecruitment during acute respiratory failure: a clinical study. Am J Respir Crit Care Med 2001;164:131-40. [Crossref] [PubMed]
- Santini A, Votta E, Protti A, et al. Driving airway pressure: should we use a static measure to describe a dynamic phenomenon? Intensive Care Med 2017;43:1544-5. [Crossref] [PubMed]
- Mezidi M, Yonis H, Aublanc M, et al. Effect of end-inspiratory plateau pressure duration on driving pressure. Intensive Care Med 2017;43:587-9. [Crossref] [PubMed]
- Bellani G, Grassi A, Sosio S, et al. Plateau and driving pressure in the presence of spontaneous breathing. Intensive Care Med 2018. [Epub ahead of print]. [Crossref] [PubMed]
- Pesenti A, Pelosi P, Foti G, et al. An interrupter technique for measuring respiratory mechanics and the pressure generated by respiratory muscles during partial ventilatory support. Chest 1992;102:918-23. [Crossref] [PubMed]
- Bellani G, Patroniti N, Weismann D, et al. Measurement of pressure-time product during spontaneous assisted breathing by rapid interrupter technique. Anesthesiology 2007;106:484-90. [Crossref] [PubMed]
- Serpa Neto A, Hemmes SN, Barbas CS, et al. Incidence of mortality and morbidity related to postoperative lung injury in patients who have undergone abdominal or thoracic surgery: a systematic review and meta-analysis. Lancet Respir Med 2014;2:1007-15. [Crossref] [PubMed]
- Serpa Neto A, Hemmes SN, Barbas CS, et al. Protective versus Conventional Ventilation for Surgery: A Systematic Review and Individual Patient Data Meta-analysis. Anesthesiology 2015;123:66-78. [Crossref] [PubMed]
- Ball L, Pelosi P. Intraoperative mechanical ventilation in patients with non-injured lungs: time to talk about tailored protective ventilation? Ann Transl Med 2016;4:17. [PubMed]
- Ladha K, Vidal Melo MF, et al. Intraoperative protective mechanical ventilation and risk of postoperative respiratory complications: hospital based registry study. BMJ 2015;351:h3646. [Crossref] [PubMed]
- Bagchi A, Rudolph MI, Ng PY, et al. The association of postoperative pulmonary complications in 109,360 patients with pressure-controlled or volume-controlled ventilation. Anaesthesia 2017;72:1334-43. [Crossref] [PubMed]
- Neto AS, Hemmes SN, Barbas CS, et al. Association between driving pressure and development of postoperative pulmonary complications in patients undergoing mechanical ventilation for general anaesthesia: a meta-analysis of individual patient data. Lancet Respir Med 2016;4:272-80. [Crossref] [PubMed]
- PROVE Network Investigators for the Clinical Trial Network of the European Society of Anaesthesiology, Hemmes SN, Gama de Abreu M, et al. High versus low positive end-expiratory pressure during general anaesthesia for open abdominal surgery (PROVHILO trial): a multicentre randomised controlled trial. Lancet 2014;384:495-503. [Crossref] [PubMed]
- Ferrando C, Soro M, Unzueta C, et al. Individualised perioperative open-lung approach versus standard protective ventilation in abdominal surgery (iPROVE): a randomised controlled trial. Lancet Respir Med [Internet] 2018 Jan [cited 2018 Feb 16]; Available online: http://linkinghub.elsevier.com/retrieve/pii/S2213260018300249
- Serpa Neto A, Campos PP, Hemmes SN, et al. Kinetics of plasma biomarkers of inflammation and lung injury in surgical patients with or without postoperative pulmonary complications. Eur J Anaesthesiol 2017;34:229-38. [Crossref] [PubMed]
- D’Antini D, Huhle R, Herrmann J, et al. Respiratory System Mechanics During Low Versus High Positive End-Expiratory Pressure in Open Abdominal Surgery: A Substudy of PROVHILO Randomized Controlled Trial. Anesth Analg 2018;126:143-9. [Crossref] [PubMed]
- Pelosi P, Rocco PRM, Gama de Abreu M. Close down the lungs and keep them resting to minimize ventilator-induced lung injury. Crit Care 2018;22:72. [Crossref] [PubMed]
- Pelosi P, Ball L, de Abreu MG, et al. General Anesthesia Closes the Lungs: Keep Them Resting. Turk J Anaesthesiol Reanim 2016;44:163-4. [Crossref] [PubMed]
- Grieco DL, Russo A, Romanò B, et al. Lung volumes, respiratory mechanics and dynamic strain during general anaesthesia. Br J Anaesth [Internet] 2018 Apr [cited 2018 Sep 4]; Available online: https://linkinghub.elsevier.com/retrieve/pii/S0007091218302794
- Bluth T, Teichmann R, Kiss T, et al. Protective intraoperative ventilation with higher versus lower levels of positive end-expiratory pressure in obese patients (PROBESE): study protocol for a randomized controlled trial. Trials 2017;18:202. [Crossref] [PubMed]
- Esteban A, Frutos-Vivar F, Muriel A, et al. Evolution of mortality over time in patients receiving mechanical ventilation. Am J Respir Crit Care Med 2013;188:220-30. [Crossref] [PubMed]
- Neto AS, Barbas CS, Simonis FD, et al. Epidemiological characteristics, practice of ventilation, and clinical outcome in patients at risk of acute respiratory distress syndrome in intensive care units from 16 countries (PRoVENT): an international, multicentre, prospective study. Lancet Respir Med 2016;4:882-93. [Crossref] [PubMed]
- Simonis FD, Barbas CS, Artigas-Raventós A, et al. Potentially modifiable respiratory variables contributing to outcome in ICU patients without ARDS: a secondary analysis of PRoVENT. Ann Intensive Care 2018;8:39. [Crossref] [PubMed]
- Schmidt MF, Amaral AC, Fan E, et al. Driving Pressure and Hospital Mortality in Patients Without ARDS: A Cohort Study. Chest 2018;153:46-54. [Crossref] [PubMed]
- Pisani L, Algera AG, Serpa Neto A, et al. PRactice of VENTilation in Middle-Income Countries (PRoVENT-iMIC): rationale and protocol for a prospective international multicentre observational study in intensive care units in Asia. BMJ Open 2018;8. [Crossref] [PubMed]
- Algera AG, Pisani L, Bergmans DC, et al. RELAx - REstricted versus Liberal positive end-expiratory pressure in patients without ARDS: protocol for a randomized controlled trial. Trials 2018;19:272. [Crossref] [PubMed]
- Serpa Neto A, Simonis FD, Barbas CS, et al. Association between tidal volume size, duration of ventilation, and sedation needs in patients without acute respiratory distress syndrome: an individual patient data meta-analysis. Intensive Care Med 2014;40:950-7. [Crossref] [PubMed]
- Neto AS, Simonis FD, Barbas CS, et al. Lung-Protective Ventilation With Low Tidal Volumes and the Occurrence of Pulmonary Complications in Patients Without Acute Respiratory Distress Syndrome: A Systematic Review and Individual Patient Data Analysis. Crit Care Med 2015;43:2155-63. [Crossref] [PubMed]
- Simonis FD, Binnekade JM, Braber A, et al. PReVENT--protective ventilation in patients without ARDS at start of ventilation: study protocol for a randomized controlled trial. Trials 2015;16:226. [Crossref] [PubMed]
- Amato MB, Meade MO, Slutsky AS, et al. Driving Pressure and Survival in the Acute Respiratory Distress Syndrome. N Engl J Med 2015;372:747-55. [Crossref] [PubMed]
- Guérin C, Papazian L, Reignier J, et al. Effect of driving pressure on mortality in ARDS patients during lung protective mechanical ventilation in two randomized controlled trials. Crit Care 2016;20:384. [Crossref] [PubMed]
- Baedorf Kassis E, Loring SH, Talmor D. Mortality and pulmonary mechanics in relation to respiratory system and transpulmonary driving pressures in ARDS. Intensive Care Med 2016;42:1206-13. [Crossref] [PubMed]
- De Jong A, Cossic J, Verzilli D, et al. Impact of the driving pressure on mortality in obese and non-obese ARDS patients: a retrospective study of 362 cases. Intensive Care Med 2018;44:1106-14. [Crossref] [PubMed]
- Serpa Neto A, Schmidt M, Azevedo LC, et al. Associations between ventilator settings during extracorporeal membrane oxygenation for refractory hypoxemia and outcome in patients with acute respiratory distress syndrome: a pooled individual patient data analysis : Mechanical ventilation during ECMO. Intensive Care Med 2016;42:1672-84. [Crossref] [PubMed]
- Aoyama H, Pettenuzzo T, Aoyama K, et al. Association of Driving Pressure With Mortality Among Ventilated Patients With Acute Respiratory Distress Syndrome: A Systematic Review and Meta-Analysis. Crit Care Med 2018;46:300-6. [Crossref] [PubMed]
- Writing Group for the Alveolar Recruitment for Acute Respiratory Distress Syndrome Trial (ART) Investigators, Cavalcanti AB, Suzumura ÉA, et al. Effect of Lung Recruitment and Titrated Positive End-Expiratory Pressure (PEEP) vs Low PEEP on Mortality in Patients With Acute Respiratory Distress Syndrome: A Randomized Clinical Trial. JAMA 2017;318:1335-45. [Crossref] [PubMed]
- Walkey AJ, Del Sorbo L, Hodgson CL, et al. Higher PEEP versus Lower PEEP Strategies for Patients with Acute Respiratory Distress Syndrome. A Systematic Review and Meta-Analysis. Ann Am Thorac Soc 2017;14:S297-303. [Crossref] [PubMed]
- Villar J, Martín-Rodríguez C, Domínguez-Berrot AM, et al. A Quantile Analysis of Plateau and Driving Pressures: Effects on Mortality in Patients With Acute Respiratory Distress Syndrome Receiving Lung-Protective Ventilation. Crit Care Med 2017;45:843-50. [Crossref] [PubMed]
- Brower RG, Lanken PN, MacIntyre N, et al. Higher versus lower positive end-expiratory pressures in patients with the acute respiratory distress syndrome. N Engl J Med 2004;351:327-36. [Crossref] [PubMed]
- Mercat A, Richard JC, Vielle B, et al. Positive end-expiratory pressure setting in adults with acute lung injury and acute respiratory distress syndrome: a randomized controlled trial. JAMA 2008;299:646-55. [Crossref] [PubMed]
- Meade MO, Cook DJ, Guyatt GH, et al. Ventilation strategy using low tidal volumes, recruitment maneuvers, and high positive end-expiratory pressure for acute lung injury and acute respiratory distress syndrome: a randomized controlled trial. JAMA 2008;299:637-45. [Crossref] [PubMed]
- Xi XM, Jiang L, Zhu B, et al. Clinical efficacy and safety of recruitment maneuver in patients with acute respiratory distress syndrome using low tidal volume ventilation: a multicenter randomized controlled clinical trial. Chin Med J (Engl) 2010;123:3100-5. [PubMed]
- Hodgson CL, Tuxen DV, Davies AR, et al. A randomised controlled trial of an open lung strategy with staircase recruitment, titrated PEEP and targeted low airway pressures in patients with acute respiratory distress syndrome. Crit Care 2011;15:R133. [Crossref] [PubMed]
- Kacmarek RM, Villar J, Sulemanji D, et al. Open Lung Approach for the Acute Respiratory Distress Syndrome: A Pilot, Randomized Controlled Trial. Crit Care Med 2016;44:32-42. [Crossref] [PubMed]