The addition of 3D printed models to enhance the teaching and learning of bone spatial anatomy and fractures for undergraduate students: a randomized controlled study
Introduction
Knowledge of skeletal anatomy and bone fractures is a fundamental educational component for every undergraduate medical student. In traditional medical education, cadaveric specimens and radiographic images are used for education and research (1-3) as well as for audio-visual resources. However, cadaveric specimens are only used in a normal skeletal anatomy course.
To teach the morphological changes that are associated with bone fracture or other medical conditions, 3D reconstructed images on PowerPoint and PACS (picture archiving and communication system) are currently the most widely used options (4). There have been ethical issues associated with the acquisition of cadavers, and in many countries, body donation is low (5-8). These circumstances, along with the expense associated with storing the cadaveric specimens, have led to limited use of cadavers (9).
Since 2000, the 3D printing technique has developed rapidly and is widely used in the manufacturing industry (10,11). The 3D printing technique has now been introduced into the medical and biomedical fields (12-15) and has potential value in clinical practice (16-19). 3D printed bone models are promisingly accurate in morphology (20), including the morphology of bone fractures. The price of 3D printing technology has now reached a reasonable cost level (13,21), and it has been introduced to be used for surgical planning (22) and medical teaching (23-25).
Whether the addition of 3D printed models enhances the teaching and learning of bone spatial anatomy and fractures by undergraduate students is still unknown. In this study, we conducted a randomized controlled trial comparing 3D printed models versus radiographic images in the education of undergraduate medical students on bone fractures.
Methods
This study was performed according to the Declaration of Helsinki principles and was approved by the Institutional Review Board (IRB) of The Second Affiliated Hospital and Yuying Children’s Hospital of Wenzhou Medical University (Approval No. 2017-01). Written informed consent was obtained from all participants, and the process was performed according to the approved guidelines.
The original CT data in DICOM format from four patients, each with a different fracture type (one spinal fracture, one pelvic fracture, one upper limb fracture, and one lower limb fracture), were obtained from the Star PACS system (INFINITT, Seoul, South Korea) of our hospital (3). All the data were imported into the software Mimics 16.0 (Materialise, Belgium). The threshold value was set at “Bone (CT)” to reconstruct the 3D images. After the 3D digital images were calculated and reconstructed (26), the data were then saved in STL format and imported into the 3D printer (Liantai, Shanghai) (27). All of the fractures described above were transformed into 3D printed models and preserved for education.
A total of 90 medical students from Wenzhou Medical University were enrolled in this study in March 2017. All students completed the gross anatomy and regional normal anatomy courses. The scores achieved on the final exam for both gross anatomy and local normal anatomy courses were collected and compared to assess the participants’ baseline knowledge of human body anatomy. Then, all of the students were randomly divided into two groups as follows: a traditional radiographic image group (45 students) and a 3D printed model group (45 students) (Figure 1).
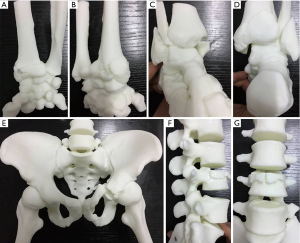
For the traditional radiographic image group, the lecture was presented using PowerPoint and included normal anatomy, the definition of fractures, and the presentation of the fractures on X-ray and CT. Before the presentation to the 3D printed model group, students were paired, and each pair received four different fracture models (one spinal fracture, one pelvic fracture, one upper limb fracture, and one lower limb fracture). Students in this group learned from a combination of PowerPoint and the visible 3D printed models.
Following the presentation to each group, each student answered 5 questions for each type of fracture. Students received two points for each question answered correctly, and a total of 20 questions were posed for the four different site fractures (File S1). Additionally, students completed questions related to a visual analog scale of satisfaction (0–10 points) representing the students’ comprehensive satisfaction with the class from any aspect (0 is very poor, 10 is very good). The results of the test and the test-taking time were assessed and recorded.
Statistical analysis
SPSS software (version 17.0, SPSS Inc., Chicago, IL, USA) was used to analyze the data. The data are described using mean and standard deviation (SD). The t-test was used to compare the two groups, and the level of significance was set at P<0.05.
Results
There were 29 males and 16 females in the traditional radiographic image group and 27 males and 18 females in the 3D printed model group. No significant differences were found in final exam scores for the gross anatomy or the regional normal anatomy courses between the traditional radiographic image group and the 3D printed model group, with P=0.498 for the gross anatomy course and P=0.574 for the regional anatomy course. The details of the summary scores are shown in Table 1. When comparing the scores between the two groups, no significant differences were observed in the scores for the upper limb test or the lower limb test (P=0.500 and P=0.563, respectively); however, the scores for the pelvis and the spine tests for the traditional radiographic image group were significantly lower than those for the 3D printed model group (P<0.001).

Full table
The details of the test-taking times are shown in Table 2. When comparing the test-taking times between the two groups, no significant differences were observed in the test-taking times for the upper limb or lower limb (P=0.603 and P=0.746, respectively); however, the test-taking times for the pelvis and the spine for the traditional radiographic image group were significantly longer than the test-taking times for the 3D printed model group (P<0.001 and P=0.002, respectively).

Full table
The visual analog scale of satisfaction mean score was 7.49±1.38 for the 3D printed model group and 5.80±1.30 for the traditional radiographic image group, with P<0.001 (Table 3).

Full table
Discussion
The 3D printing technique can provide a 3D printed model of almost any shape from a 3D digital model or other electronic data source (10,27). It can also provide an accurate model (20,28) for use in anatomy education (29), which may be especially useful in some countries that lack cadaver donations (5,7). Further, most cadaveric specimens have normal anatomy and are not useful for learning about fractures.
For the traditional radiographic image group, a PowerPoint of X-ray and CT images and 3D anatomical software were used for the medical education. The 3D printed technique may help us to improve medical education. Langridge et al. (30) performed a systematic review of the use of 3D printing in surgical training and assessment. They found the following: previous studies had significant heterogeneity, which precluded any meta-analysis; most studies looked at use of 3D printing in the areas of neurosurgery and otorhinolaryngology; and 3D printing showed better outcomes in most of these studies. For anatomy education, there had been three randomized controlled studies: one about the hepatic segments (31), one about external cardiac anatomy (25), and one about spinal fractures (32).
In a study by Li et al. (32), 3D printed models significantly improved the identification of spinal fractures by medical students; however, only spinal fractures were studied. In the current study, the sites of bone anatomy and fractures included the spine, pelvis, upper limbs and lower limbs. We found that the summary scores were similar between the two groups for the upper limb and lower limb tests, and the scores were significantly higher in the 3D printed model group than in the traditional radiographic image group for the spine and pelvis tests. Compared to the study by Li et al. (32), our present findings suggest that 3D printed models may be helpful for understanding bone anatomy and fractures of the pelvis and spine, but not of the upper and lower limbs.
One factor contributing to the different test results obtained in this study may be that the anatomy of the pelvis and spine is more complex than limb anatomy, thus making it harder for students to understand the spatial anatomy of the pelvis and spine compared to the upper and lower limbs. The mean scores of the pelvis and spine tests were less than 5 among the traditional radiographic image group, whereas students in the 3D printed model group demonstrated higher scores by 1.96 and 2.17 points on the pelvis and spine tests, respectively. The mean scores of the upper and lower limb tests were more than 7 among both groups. Students did not demonstrate a significant benefit from the 3D printed models, suggesting that it is easier to understand the spatial anatomy of upper and lower limbs, and the 3D printed models were not necessary.
Interestingly, we found that the students who had lower test-taking times had higher summary scores, which confirmed that the students in the 3D printed model group benefitted from greater understanding of fractures of the pelvis and spine and that they answered questions at a faster rate.
The mean score on the visual analog scale of satisfaction for the students in the 3D printed model group was 1.69 points (16.9%) higher than the mean score of the traditional radiographic image group (P<0.001), suggesting the 3D printed model method of education improved student satisfaction.
One benefit of using a 3D printed model is that the 3D printed model does not have a significant cost (33). The price of the 3D printing material is different across countries and brands (34,35). In this study, the weight of the spine, the upper limb and the lower limb models ranged from 40 to 70 grams, and the pelvis model weighed approximately 600 grams. The cost of the print material was approximately $0.15 per gram. Therefore, the cost of the spine, the upper limb and the lower limb models was approximately $6–$10.50, and the pelvis model cost approximately $90, which does not include the depreciation of the 3D printer. The printed model can be used multiple times and preserved for a long period of time.
Although it is easier to obtain images of bodies for 3D printing than to obtain a whole body for donation (36), the ethics of 3D printing copies of bodies donated for medical education and research should be considered (37). Informed consent needs to be obtained and protection of individual donor information needs to be ensured.
There were some limitations to this study and to the 3D printing techniques. First, the 3D printed models in this study did not include soft tissue, such as nerves, vessels and muscles around the bone. Second, the 3D printing process takes time, from hours to days, depending on the volume of the printed models. The time requirement may be reduced with further development of 3D print technology. Third, the sample size of this study was not large, and some potential bias or heterogeneity may have influenced the results. We used a randomized method to avoid the potential bias as much possible.
Conclusions
The 3D printed model may improve medical students’ understanding of bone spatial anatomy and fractures in some anatomically complex sites and may improve student satisfaction in medical education.
Supplementary
The questions
Part One
1.What’s name of bone that marked as “A”
- Ulna
- Radius
- Fibula
- Tibia
2. What’s name of bone that marked as “B”
- Ulna
- Radius
- Fibula
- Tibia
3. Which bone involves fracture?
- None of “A” and “B”
- Both of “A” and “B”
- Only “A”
- Only “B”
4. What’s name of the joint that marked as “C”?
- Knee Joint
- Elbow joint
- Ankle joint
- Wrist joint
5. What’s the name of bone that locates at proximal end of “A” and “B”?
- Femur
- Patella
- Humerus
- Scapula
Part Two
1. What’s name of bone that marked as “A”
- Ulna
- Radius
- Fibula
- Tibia
2. What’s name of bone that marked as “B”
- Ulna
- Radius
- Fibula
- Tibia
3. Which bone involves fracture?
- None of “A” and “B”
- Both of “A” and “B”
- Only “A”
- Only “B”
4. What’s name of the joint that marked as “C”?
- Knee Joint
- Elbow joint
- Ankle joint
- Wrist joint
5. What’s the name of bone that locates at distal end of “A” and “B”?
- Calcaneus
- Talus
- Cuboid bone
- Navicular bone
Part Three
1. What’s name of bone that marked as “B”
- Sacrum
- Pubis
- Ilium
- Ischium
2. What’s name of the joint/symphysis that marked as “A”?
- Sacroiliac joint
- Hip joint
- Pubic symphysis
- Sacrococcygeal symphysis
3. Which bone involves fracture?
- Only the bone of Pubis and Ischium.
- Only the bone of pubis, ischium and sacrum
- Only the bone of pubis, ischium and ilium.
- All the bone of pubis, ischium, sacrum and ilium.
4. Did the fracture involve the following joints?
- None of “Sacroiliac joint” and “Hip joint”.
- Both of “Sacroiliac joint” and “Hip joint”.
- Only “Sacroiliac joint”
- Only “Hip joint”.
5. Did the fracture involve the pubic tubercle?
- Yes
- No
Part Four
1. what’s name of bone that marked as “A”
- Spinous process
- Vertebral body
- Vertebral disc
- Sacrum
2. What’s name of the site marked as “B”?
- Transverse foramen
- Intervertebral space
- Pedicle
- Intervertebral foramen
3. Which vertebrae does the fracture involve?
- L3
- L4
- L5
- Both L4 and L5
4. Which following construct does the fracture involve?
- Vertebral body
- Vertebral body and pedicle
- Vertebral body, pedicle and lamina
- Vertebral body, pedicle, lamina and spinous Process
5. Which following construct impacted by the fracture?
- Intervertebral space
- Intervertebral space and vertebral foramen
- Intervertebral space, vertebral foramen and intervertebral foramen
- None of above.
Acknowledgements
Funding: This work was supported by the Higher Education Teaching Reform Project of Wenzhou Medical University (YBJG201826), Wenzhou Municipal Science and Technology Bureau (Y20170389), Wenzhou Leading Talent Innovative Project (RX2016004), Zhejiang Provincial Medical Technology Foundation of China (2018KY129), and the National Natural Science Foundation of China (81501933).
Footnote
Conflicts of Interest: The authors have no conflicts of interest to declare.
Ethical Statement: This study was performed according to the Declaration of Helsinki principles and was approved by the Institutional Review Board (IRB) of The Second Affiliated Hospital and Yuying Children’s Hospital of Wenzhou Medical University (Approval No. 2017-01). Written informed consent was obtained from all participants, and the process was performed according to the approved guidelines.
References
- Ravesloot CJ, van der Schaaf MF, van Schaik JP, et al. Volumetric CT-images improve testing of radiological image interpretation skills. Eur J Radiol 2015;84:856-61. [Crossref] [PubMed]
- Critchley EP, Smirniotopoulos JG. Internet-accessible radiographic database of Vietnam War casualties for medical student education. Mil Med 2003;168:271-3. [Crossref] [PubMed]
- Wu AM, Ni WF, Shao ZX, et al. Percutaneous posterior transdiscal oblique screw fixation with lateral interbody fusion: a radiological and cadaveric study. Eur Spine J 2015;24:852-8. [Crossref] [PubMed]
- Muller-Stich BP, Lob N, Wald D, et al. Regular three-dimensional presentations improve in the identification of surgical liver anatomy - a randomized study. BMC Med Educ 2013;13:131. [Crossref] [PubMed]
- Zhang L, Wang Y, Xiao M, et al. An ethical solution to the challenges in teaching anatomy with dissection in the Chinese culture. Anat Sci Educ 2008;1:56-9. [Crossref] [PubMed]
- Boulware LE, Ratner LE, Cooper LA, et al. Whole body donation for medical science: a population-based study. Clin Anat 2004;17:570-7. [Crossref] [PubMed]
- Halou H, Chalkias A, Mystrioti D, et al. Evaluation of the willingness for cadaveric donation in Greece: a population-based study. Anat Sci Educ 2013;6:48-55. [Crossref] [PubMed]
- Rokade SA, Gaikawad AP. Body donation in India: social awareness, willingness, and associated factors. Anat Sci Educ 2012;5:83-9. [Crossref] [PubMed]
- Gilbody J, Prasthofer A, Ho K, et al. The use and effectiveness of cadaveric workshops in higher surgical training: a systematic review. The Annals of The Royal College of Surgeons of England 2011;93:347-52. [Crossref] [PubMed]
- Horn TJ, Harrysson OL. Overview of current additive manufacturing technologies and selected applications. Sci Prog 2012;95:255-82. [Crossref] [PubMed]
- Haverman TM, Karagozoglu KH, Prins HJ, et al. Ned Tijdschr Tandheelkd 2013;120:136-41. [Rapid prototyping: a very promising method]. [Crossref] [PubMed]
- Baba M, Matsumoto K, Yamasaki N, et al. Development of a Tailored Thyroid Gland Phantom for Fine-Needle Aspiration Cytology by Three-Dimensional Printing. J Surg Educ 2017;74:1039-46. [Crossref] [PubMed]
- Wilcox B, Mobbs RJ, Wu A-M, et al. Systematic review of 3D printing in spinal surgery: the current state of play. J Spine Surg 2017;3:433-43. [Crossref] [PubMed]
- Hoang D, Perrault D, Stevanovic M, et al. Surgical applications of three-dimensional printing: a review of the current literature & how to get started. Ann Transl Med 2016;4:456. [Crossref] [PubMed]
- Sun Z, Liu D. A systematic review of clinical value of three-dimensional printing in renal disease. Quant Imaging Med Surg 2018;8:311-25. [Crossref] [PubMed]
- Maruthappu M, Keogh B. How might 3D printing affect clinical practice. 2014;349:g7709.
- McMenamin PG, Quayle MR, McHenry CR, et al. The production of anatomical teaching resources using three-dimensional (3D) printing technology. Anat Sci Educ 2014;7:479-86. [Crossref] [PubMed]
- Wu AM, Lin JL, Kwan KYH, et al. 3D printing techniques in spine surgery: the future prospects and current challenges. Expert Rev Med Devices 2018;15:399-401. [Crossref] [PubMed]
- Fiz N, Delgado D, Sanchez X, et al. Application of 3D technology and printing for femoral derotation osteotomy: case and technical report. Ann Transl Med 2017;5:400. [Crossref] [PubMed]
- Wu AM, Shao ZX, Wang JS, et al. The accuracy of a method for printing three-dimensional spinal models. PLoS One 2015;10. [Crossref] [PubMed]
- Watson RA. A low-cost surgical application of additive fabrication. J Surg Educ 2014;71:14-7. [Crossref] [PubMed]
- Perica E, Sun Z. Patient-specific three-dimensional printing for pre-surgical planning in hepatocellular carcinoma treatment. Quant Imaging Med Surg 2017;7:668-77. [Crossref] [PubMed]
- Balestrini C, Campo-Celaya T. With the advent of domestic 3-dimensional (3D) printers and their associated reduced cost, is it now time for every medical school to have their own 3D printer? Med Teach 2016;38:312-3. [Crossref] [PubMed]
- Loke YH, Harahsheh AS, Krieger A, et al. Usage of 3D models of tetralogy of Fallot for medical education: impact on learning congenital heart disease. BMC Med Educ 2017;17:54. [Crossref] [PubMed]
- Lim KH, Loo ZY, Goldie SJ, et al. Use of 3D printed models in medical education: A randomized control trial comparing 3D prints versus cadaveric materials for learning external cardiac anatomy. Anat Sci Educ 2016;9:213-21. [Crossref] [PubMed]
- Chen D, Chen CH, Tang L, et al. Three-dimensional reconstructions in spine and screw trajectory simulation on 3D digital images: a step by step approach by using Mimics software. J Spine Surg 2017;3:650-6. [Crossref] [PubMed]
- Rengier F, Mehndiratta A, von Tengg-Kobligk H, et al. 3D printing based on imaging data: review of medical applications. Int J Comput Assist Radiol Surg 2010;5:335-41. [Crossref] [PubMed]
- Mafeld S, Nesbitt C, McCaslin J, et al. Three-dimensional (3D) printed endovascular simulation models: a feasibility study. Ann Transl Med 2017;5:42. [Crossref] [PubMed]
- AbouHashem Y, Dayal M, Savanah S, et al. The application of 3D printing in anatomy education. Med Educ Online 2015;20:29847. [Crossref] [PubMed]
- Langridge B, Momin S, Coumbe B, et al. Systematic Review of the Use of 3-Dimensional Printing in Surgical Teaching and Assessment. J Surg Educ 2018;75:209-21. [Crossref] [PubMed]
- Kong X, Nie L, Zhang H, et al. Do 3D Printing Models Improve Anatomical Teaching About Hepatic Segments to Medical Students? A Randomized Controlled Study. World J Surg 2016;40:1969-76. [Crossref] [PubMed]
- Li Z, Xu R, Li M, et al. Three-dimensional printing models improve understanding of spinal fracture--A randomized controlled study in China. Sci Rep 2015;5:11570. [Crossref] [PubMed]
- Rogers-Vizena CR, Sporn SF, Daniels KM, et al. Cost-Benefit Analysis of Three-Dimensional Craniofacial Models for Midfacial Distraction: A Pilot Study. Cleft Palate Craniofac J 2017;54:612-7. [Crossref] [PubMed]
- Bradshaw S, Bowyer A, Haufe P. The intellectual property implications of low-cost 3D printing. Script-ed 2010;26:1-22.
- Anzalone GC, Zhang C, Wijnen B, et al. A Low-Cost Open-Source Metal 3-D Printer. IEEE Access 2013;1:803-10.
- AbouHashem Y, Dayal M, Serafin S, et al. Students' attitudes toward body image donation for 3D printing. Clin Anat 2017;30:1005-6. [Crossref] [PubMed]
- Cornwall J. The ethics of 3D printing copies of bodies donated for medical education and research: What is there to worry about? Australas Med J 2016;9:8-11. [Crossref] [PubMed]