Mechanical ventilation and respiratory monitoring during extracorporeal membrane oxygenation for respiratory support
Introduction
Extracorporeal membrane oxygenation (ECMO) is an extracorporeal technique able to provide temporary gas exchange support in patients with respiratory failure refractory to conventional treatment (1). ECMO is able to support gas exchanges in patients with refractory hypoxia, and one of its main indications is in patients with acute respiratory distress syndrome (ARDS) (2).
The first successful use of ECMO was reported in 1972 in a patient with post-traumatic ARDS (3) and since then, technological improvements facilitated a wider expansion of the technique along with decreased complications and improved outcomes (4).
Over the last decade, literature has widely focused on the indications, timing, complications and the effects on outcome (5-9). Mechanical ventilation (MV) and the ventilator management of the native lung play a central role during ECMO. Nevertheless, surprisingly, this aspect has received little attention (10-16). Most studies report only ventilator setting and respiratory mechanics data before ECMO; few studies report also data regarding the first day of ECMO (Table 1); and very few studies extend the description of ventilator parameters beyond the first day. For these reasons, it is very difficult to recognize and reproduce specific ventilator approaches; there are no enough data to compare different studies, and not enough data supporting a specific ventilatory approach. Even less information are available on how respiratory monitoring is accomplished.
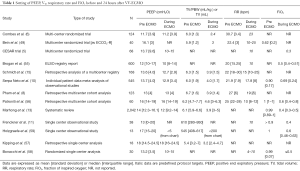
Full table
The aim of this review is to describe the pathophysiological principles of MV during ECMO for respiratory failure in patients with ARDS and to report on applied ventilatory strategies and respiratory monitoring on the basis of the current evidence and available literature. As the veno-venous (VV-ECMO) configuration is the most frequently used for ECMO respiratory support (23) this review will be confined to the use of MV during VV-ECMO.
Conventional ventilatory management of ARDS patients and indications to ECMO
ARDS is defined as a life threatening condition characterized by refractory hypoxemia (2).
Despite several advances in intensive care management over the last decades, mortality and morbility associated with ARDS is still high, with a mortality rate of 34–58% (24-29), and a large amount of survivors harboring permanent physical and neuropsychological impairment with a consequent dramatic loss of productive life-years (29,30).
MV is a mainstay element in the management of patients with respiratory failure as it provides adequate gas exchange while buying time for the lung to heal. However, a growing body of evidence has highlighted that, even though necessary, MV per se could activate inflammation and further worsen lung damage leading to the so-called ventilator-induced lung injury (VILI) (31). VILI is caused by different mechanisms (32,33) which include the application of pressure (stress, barotrauma) (34,35), the distension of the lung (strain, volotrauma) (36,37), the shear force associated to cyclical alveoli opening and closing (atelectrauma) (33), trigger of lung inflammation (biotrauma) (38,39), and oxygen lung toxicity by use of high fraction of inspired oxygen (40,41).
Current approach to MV of ARDS patients aims to provide adequate gas exchange, while minimizing VILI.
Key elements of protective MV are the use of volume and pressure limited ventilation which have shown to be have a benefit on patients’ outcome (42,43). Adjunctive therapies that have shown benefit on mortality in most severe ARDS patients are prone positioning (44,45) and neuromuscular blockade (46,47). In patients with more severe impairment of gas exchange permissive hypercapnia, high levels of PEEP (48), and recruitment maneuvers (RMs) (49) are variably and sometimes forcedly applied.
Indication to respiratory ECMO
In the management of acute respiratory failure and ARDS, use of ECMO has two main indications:
Pathophysiology of gas exchanges during ECMO
During VV-ECMO the artificial oxygenator and the native lung are in series. Venous blood is diverted from the venous compartment towards the artificial oxygenator, which provides blood oxygenation and removal of CO2. The blood is then returned to the patient (1).
Gas transfer exchange through the membrane depends on different factors: the intrinsic performance and characteristics of the membrane, the membrane surface area, and the oxygen and carbon dioxide pressure gradient between the flow and the blood.
The CO2 content of blood is high; 500 mL of venous blood contains around 250 mL/min, which is approximately the entire minute CO2 production, thus allowing CO2 removal even with relatively low ECMO blood flow (BF). The sweep gas flow reduces the partial pressure of CO2 inside the hollow fibers and therefore increases the partial pressure gradient between gas and blood. The sweep gas flow ventilating the artificial lung is then the main determinant of the amount of CO2 removed.
Removal of CO2 through the ML enables to reduce the respiratory minute volume through reduction of both tidal volume (i.e., indirectly the PPLAT), and/or RR, thus maximizing the possibility of protective ventilation (see Figure 1). It also allows to reduce dynamic hyperinflation and uncontrolled hypercapnia during status asthmaticus (55) or chronic obstructive pulmonary disease (COPD) (56) or as a bridge to lung transplant (57).
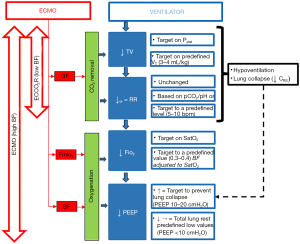
On the other hand, oxygen solubility in plasma is minimal (0.003 mL/mmHg per 100 mL of blood) and the oxygen content in the blood is limited by hemoglobin concentration and saturation. Blood leaves the peripheral tissues with a low content of oxygen. The ECMO circuit withdraws part of the venous blood towards the membrane lung, and the blood returning to the right heart is the result of both well oxygenated extracorporeal blood and deoxygenated venous return. The direct effect of VV-ECMO on oxygenation is increasing of the mixed venous saturation of the blood returning to the patient lung. The amount of delivered oxygen is directly related to the amount of ECMO BF relative to total patient cardiac output, and inversely related to the oxygen saturation of drained BF. As a result for the ventilator setting, oxygenation support provided by ECMO may allow reduction of ventilator FiO2 and airway pressures (PEEP and indirectly PPLAT) (see Figure 1).
Ventilatory strategies during vv-ECMO
It is important to understand that there is a complex interaction between patient, extracorporeal circuit and ventilator.
Artificial lung and native lung both participate to gas exchange. The amount of ECMO BF, and then choice of ECMO equipment and cannula size, depends on the residual gas exchange function of the native lung, which ultimately depend on the ventilator setting we choose. Conversely, the degree of native lung rest we may achieve in terms of minute ventilation and airway pressure will depend on the efficiency of ECMO in delivering oxygen and removing CO2.
Rational of ventilator setting during vv-ECMO for minimizing VILI
As the main objective of ECMO support, after assuring adequate oxygenation, is the prevention of VILI, it is crucial to understand how changes in the ventilator setting relate to mechanisms of VILI.
For instance, reduction of VT and PEEP has important effects on alveolar stress, strain, and shear stress. Alveolar strain, which represents the amount of alveolar distension from a resting volume, is one of the main mechanisms, responsible for volutrauma (36,37). In patients with ARDS undergoing ECMO this is a dramatic concern as these patients have very small area of normally aerated alveoli that receiving most of VT undergo to extreme strains. Assuming functional residual capacity as the resting volume of the lung, during MV the total strain may be separated in two components (36). The static strain, which is related to the lung volume consequent to the application of PEEP, and the dynamic strain which is directly related to VT.
Airway pressures relevant for lung stress are PPLAT (which is directly related to total lung strain) and driving pressure (which is directly related to dynamic strain) (37).
Finally, we should not forget atelectrauma associated to cyclical alveoli opening and closing. This is important considering that ventilator settings leading to lower degree of baro and/or volotrauma may lead to worst atelectrauma.
Terminology for ventilator strategies during ECMO
After initiation of ECMO, different ventilator approaches are possible depending on how VT, RR, PEEP and FiO2 are set.
Expressions like “lung rest” (1,14-16) or “ultra-protective ventilation” (53,54) are variably and sometimes interchangeably used when referring to protective ventilatory strategies. However, such expressions are generic and imprecise and do not refers to specific ventilator settings. As a general orientation, the term “ultra-protective ventilation” (54) has been more often utilized when referring to strategies mainly characterized by decrease of VT, accompanied or not by a decrease in RR, as for example with ECCO2R. The term “lung rest” is more indefinite, and even more imprecise, as it his utilize indifferently to describe a low VT ventilation with or without a decrease in PEEP and mean airway pressure (1,14-16). To indicate a strategy combining ultra-protective ventilation with low level of PEEP, some authors have proposed the expression “total lung rest” (14-16,58).
In a recent international survey conducted among Extracorporeal Life Support Oxygenation (ELSO) registry centers by Marhong et al. (12), when asked about primary goal in setting MV during ECMO, the respondents could choose between four possibility: “lung rest (i.e., avoid ventilator associated lung injury)”, “alveolar recruitment (i.e., opening the lug)”, “varies greatly depending on most responsible intensivist/physician”, and “others”. Not surprisingly, 77% of centers declared lung rest was their primary goal (12). However, the way the question was placed, opposing the concept of lung opening to that of VILI prevention, assume that alveolar recruitment does not play a role in lung protection. Moreover, it does not consider the possibility, widely seen in the clinical practice, of decreasing minute ventilation while setting a level of PEEP sufficient to avoid derecruitment.
MV protocols: international guidelines, recommendations, single center and randomized controlled trials
ELSO guidelines for respiratory failure (59) suggest to target ventilator management to use FiO2PLAT 2O. It is recognized the possibility total consolidation of the lung, and use of “some inflation pressure as high pressures are decreased” is suggested to avoid lung collapse. For the first 24 hours, moderate to heavy sedation and pressure controlled ventilation with PEEP 15 and peak airway pressure of 25 cmH2O is suggested along with a RR of 5 bpm. However, there are no specific limits to the level of PEEP provided that negative hemodynamics effects are avoided. After 24–48 hours, lower levels of pressure (PEEP 10 and peak airway pressure of 20 cmH2O) are recommended, and use of light sedation promoting some spontaneous breathing is suggested. It is important to remind that ELSO guidelines simply describe useful and safe practice and are not intended to define a standard of care. However, few points deserve some discussion. First, in spite of the attention given in the literature, there is no specific recommendation about VT setting, and PPLAT and RR are the only recommended ventilator targets. Second, the step decrease in PEEP down to 10 cmH2O recognizes the danger of too fast decrease in PEEP after ECMO starting. At the same time, however, there is little attention to the need of individualize how fast to reach specific ventilatory targets according to before-ECMO ventilator conditions: especially PEEP, minute ventilation requirements, alveolar recruitability and PEEP response, presence of alveolar plasma leakage and lung infection. Finally, in spite of the important consequences that MV management during ECMO may have on respiratory mechanics and lung function, there is no indication on respiratory monitoring to target ventilator setting and follow its effects.
Review articles, position papers, and expert point of view recognize some of the above points. In particular the role of PEEP in avoiding alveolar derecruitment associate to ultra-protective ventilation and its potential contribution in protecting from VILI is widely recognized (1,14,16,58).
Surprisingly, in the survey by Marhong et al. (12) only 27% of centers declared of having a MV protocol during VV-ECMO. Likely, this may in part be explained with the fact that in clinical practice there is a wide inter-patient variability and ventilator setting needs to be individualized making protocols difficult to define and apply.
In the CESAR trial, ECMO system was designed to provide high BFs capabilities (>5 L/min) and full substitution of pulmonary gas exchange. Ventilator setting was targeted to peak inspiratory pressure of 20 cmH2O, using PEEP of 10 cmH2O, RR of 10 bpm, and FiO2 of 30% (5). Unfortunately, no data are reported on ventilator parameters and time to reach the above targets after ECMO initiation.
In the EOLIA trial VT was targeted to limit PPLAT below 24 cmH2O in conjunction with a PEEP of at least 10 cmH2O and FIO2 of 0.3–0.5 (6). The published study and the available data supplement provide a full report of ventilator parameters, including important respiratory mechanics variables such as respiratory system compliance (Crs), before and during ECMO. This is extremely important as no other study provide such extensive information.
MV practice from prospective and retrospective observational studies
Data retrieved from observational studies are the main source of information on center clinical practices (Table 1) (5,6,8,10,11,13,15,17-19,21,22,57). However, the lack of a standardization in reporting data, and the apparent lack in the use and report of monitoring variables that would be useful to interpret and compare different practices, make these data of scarce utility. Nevertheless, a common trend in clinical practice is recognizable, and supported by physiological background, it is possible to build an indicative flow chart that may guide through different ventilator strategies (see Figure 1).
With no exception, pressure controlled is the preferred mode of ventilation. A common trend after starting ECMO for refractory hypoxia is the decrease of VT and FiO2 with or without decrease in RR. In case of ECCO2R indications, FiO2 is left unchanged. Setting of PEEP depends on the ventilatory strategy (total lung rest vs. prevention of alveolar derecruitment).
Setting of VT
When asked about VT setting, 81% of adult centers declare VT setting lower than 6 mL/kg (34% below 4 mL/kg). This corresponds to what reported in most studies (Table 1). The decrease in VT results in decrease in both PPLAT and driving pressure. Theoretically, a full high flow ECMO may provide enough CO2 removal to virtually eliminate the need for any ventilation and exploit a total rest lung strategy. Nevertheless, no study has explored this possibility. The studies by Serpa Neto et al. (10) and Schmidt et al. (15) are the most detailed font of information. Serpa Neto et al. collected individual data of 545 pts from nine studies. VT was decreased in the first 24 hour after ECMO from 6 to 4 mL/kg. This determined a decrease in PPLAT and driving pressure of almost 5 cmH2O. The only respiratory parameter associated with survival was driving pressure before ECMO.
Schmidt et al. collected data, retrospectively, from three expert centers (2 Australians and 1 French) (15). VT was decreased from 6.3±1.5 to 3.9±1.6. PPLAT and driving pressure decrease by around 5 cmH2O in average.
It must be recognized that, in most reports, VT is not target directly, but rather it is the result of targeting a safe level of PPLAT. This strategy is also supported by recent observation by Pham et al. showing that a high PPLAT on the first day of VV-ECMO for acute respiratory failure was significantly associated with mortality (8). However, in the last years, following the concept of ultra-protective ventilation, some studies have explored the possibility of directly setting a target low VT (3–4 mL/kg). Bein et al. have first investigated the feasibility of ventilating with 3 mL/kg (17). More recently, different authors have explored the possibility of lowering VT to 4 mL/kg using ECMO systems able to run at BFs as low as 300–400 mL/min (54). It is important to remember that this strategy has been proposed and explored in less severe ARDS patients in whom oxygenation is not severely impaired.
RR
Published data show, in the average, a moderate decrease in RR. Two different trends may be observed. Some center target low levels of RR (5–10 bpm) as suggested in ELSO guidelines, probably considering RR an important contributor of VILI. Other centers decrease RR moderately probably to avoid hypocapnia that may result from the CO2 removal efficiency of ECMO. It is worth notice that RR data are often not reported.
Decrease of VT and RR are achievable with all ECMO indication and systems (high BF ECMO and ECCO2R).
FiO2
In case of high BF ECMO providing sufficient oxygenation support also FiO2 and PEEP may be decreased. As for RR, also reduction of FiO2 shows a wide inter-center variability. Except for few centers aiming to levels as low as 0.3 since from the very beginning of ECMO, in most centers FiO2 is reduced more progressively. This difference, likely reflects a different strategy on the native lung. If a total lung rest strategies is employed there is no usefulness in administering high level of FiO2.
PEEP
Differently from VT, declared practice about PEEP setting after ECMO initiation show higher variability, with 47% of centers aiming to PEEP lower than 10 cmH2O (12). This data contrasts with what reported by most studies showing average PEEP values above 10 cmH2O. This reflects the fact that, while there is a common general agreement on the role of lowering VT, RR and FiO2 to maximize protection from VILI, setting of PEEP is still a matter of great debate. The role of PEEP in VILI is multifactorial and controversial. On one side, increase of PEEP determines an increase in static strain, and, if no alveolar recruitment is present, even overdistention with increase in PPLAT and driving pressure. In addition, inappropriate high levels of PEEP have hemodynamic detrimental effects secondary to decreased of venous return and increase of right heart afterload. On the other side, depending on the amount of associate alveolar recruitment, increase of PEEP may be associated with a decrease in driving and PPLAT and dynamic strain. Moreover, PEEP may stabilize alveoli and protect from atelectrauma. These considerations are even more important during VV-ECMO, where the use of very low VT and RR causes alveolar instability and lung collapse. As a consequence, respiratory system compliance decrease, the expected decrease in driving pressure is dumped, and mechanism of atelectrauma exacerbated. In this context, there are convincing evidences that PEEP may play an important protective role (15). The decrease in VT results in a decrease in dynamic strain, PPLAT and driving pressure which are considered the most important determinant of VILI.
In an experimental study on 20 animals, Protti et al. showed that at equal level of injurious total strain, combination of high static and low dynamic strain (i.e., high PEEP and low VT) was associated with less VILI compared to application of low static and high dynamic strain. They concluded that VILI was prevalently associated with dynamic strain, and that static strain may also play a protective role (36).
In the study by Schmidt et al. higher PEEP level in the first 24 hours after ECMO were associated with better survival (15).
When analyzing available data about PEEP setting during ECMO, few points deserve particular attention to avoid over interpretation.
First, published data show a very moderate decrease in the average PEEP (around 1–2 cmH2O) in the first 24 hours of ECMO. This marginal change in the average PEEP across the studies hides a wide interpatient variability in individual PEEP settings. It is likely that PEEP may be decreased or increased in the same center on different patients depending on the individual patient response.
Second, as evident from Table 1, there is a wide range variability in the average PEEP even before ECMO (from 12 to 18 cmH2O), reflecting the center by center differences in PEEP criteria before ECMO. Theoretically, larger alveolar collapse and more detrimental effects on the native lung are expected after bigger and faster reduction in PEEP. This may explain why in studies where high PEEP levels are present before ECMO, there are no changes in PEEP in the first 24 hours of ECMO.
Third, independently from any other ventilator parameter PEEP level is the main determinant of residual native lung function. At low PEEP level, native lung contribution may became minimal and patient oxygenation totally dependent on ECMO. In this condition, any ECMO related complication might put the patient at risk of life.
Finally, extensive lung collapse may lead with time to extreme pulmonary vasoconstriction, refractory pulmonary hypertension, and right heart failure that may require conversion from VV to VA-ECMO.
The historical ECMO center of Karolinska Institute is the unique ECMO center, from available published data, really adopting a total lung rest strategy (11,20). The report by Holzgrafe et al. on their ECMO experience in patient with H1N1 is particularly useful, since it allows to appreciate several aspects of a total lung resting approach (20). First, PEEP was decreased from a median of 17 cmH2O down to 5 cmH2O during ECMO. During ECMO, the contribution of native lung was minimal, and in spite of the high ECMO BF, at maximal oxygen supply arterial oxygen saturation was around 85%. This is a very crucial point, because it reflects an intrinsic limitation of VV-ECMO in providing oxygenation when native lung residual contribution to oxygenation is minimal. Finally, in 4 of 13 patients, VV-ECMO had to be switch to VA-ECMO because pulmonary hypertension and right heart failure. This high rate of conversion from VV-ECMO to VA-ECMO is not reported in any other study and likely reflects the negative effects of a low PEEP-total lung rest strategy on pulmonary circulation and right heart function.
Respiratory monitoring during VV-ECMO
To date very little data exist on respiratory monitoring during VV-ECMO. This is disappointingly as very simple measurements may have a great utility in managing MV during ECMO. Respiratory monitoring during ECMO has three main goals: monitor the function of native lung, guide ventilator setting, monitor possible complications.
Monitor of gas exchange
Traditional blood gas analysis is essential to drive ECMO setting in providing adequate gas exchange. However, it may not be used to assess the native lung function, as the both PaO2 and PaCO2 will depend on the relative contribution of native and membrane lung. Thus, classical indices of oxygenation such as PaO2/FiO2 have little value during VV-ECMO. Given the relatively low VT and minute ventilation capnography may be of limited utility. Still some important information may be derived if daily changes are strictly monitor (60). On the oxygenation side, an interesting approach is to monitor on daily basis the effect of administering FiO2 of 1 on the ventilator side. When the lung function is impaired and the contribution of native lung is low, setting FiO2 to 1 will have minimal or no effects on PaO2. However, as the lung function will improve the administration of a high FiO2 will result in bigger and bigger increase in PaO2. On the same basis, end tidal-CO2 (ETCO2) may be very low during VV-ECMO. However, as the lung function will improve ETCO2 will increase prompting the need to decrease sweep gas on the ECMO side (60).
Monitor of respiratory mechanics
ECMO per se has no direct influence on respiratory mechanics, and the classical simple monitoring variables are still valid during ECMO. VT, PPLAT, driving pressure, and respiratory system compliance should be strictly and frequently measure as these variables are important not only to guide in the ventilator setting, but also to assess the effect of ventilator changes on lung function. In particular, assess of alveolar recruitment/derecruitment and/or overdistension are crucial. Hemodynamic monitoring is of course also important. Pulmonary arterial pressure and right heart function should be strictly monitored, especially when high PEEP levels or, at the opposite, a total lung rest strategy are used. In this context, echocardiography is essential. With femorofemoral cannulation approach, use of Pulmonary Artery Catheter is feasible and a continuous monitor of pulmonary arterial pressure and intrapulmonary shunt are possible (60).
Advanced respiratory monitoring
In the last years, new promising monitor tools have been proposed.
Given the difficulties in obtain frequent thoracic computed tomographic assessment during ECMO, less invasive imaging tools have recently been tested. Franchineau et al. have recently assessed the possibility of titrating PEEP level during ECMO by electrical impedance tomography (EIT) (61). Fifteen patients underwent to a decremental PEEP trial from 20 to 0 cmH2O. EIT derived parameters were used to quantify at each PEEP level the degree of collapse and overdistention. They found a wide variability in the optimal PEEP level and concluded on the need of individualization of ventilator setting during ECMO.
PPLAT and driving airway pressure are a measure of the stress applied to all respiratory system. Depending on chest wall compliance, the amount of airway pressure effectively applied to the lung is unknown unless transpulmonary pressure is measured (62-64). Grasso et al. assessed the possibility of identify patients requiring ECMO by measuring transpulmonary pressure in 14 patients with severe ARDS referred for ECMO (65). In seven cases, the transpulmonary pressure was lower than 25 cmH2O (considered a safe level). In these patients PEEP was raised from 17.9±1.2 to 22.3±1.4 cmH2O to obtain a target transpulmonary pressure of 25 cmH2O. As the oxygenation improved, these patients were successfully managed without ECMO. On other seven patients, transpulmonary pressure was higher than 25 cmH2O and all patients received ECMO.
Conclusions and recommendations
MV during ECMO plays an important role on patients’ complications and outcome and often the lack of standardized protocols has influenced the results of outcomes of patients undergoing ECMO support.
The optimal ventilator strategy during ECMO is still debated, with great variability among the centers. Obtaining lung protective ventilation appears to be feasible with extracorporeal support but the optimal targets and the timing to achieve and maintain these parameters are not clear. A number of ventilator strategies may potentially be used to avoid VILI. Based on available data the most reasonable approach is reduction of VT to safe level of PPLAT, reduction of RR to moderate levels (10–15 bpm) and maintain moderate respiratory levels of PEEP targeted in preventing alveolar derecruitment and avoiding overdistention. To guide on this process respiratory monitoring is essential. While advanced tools, such EIT and transpulmonary pressure are promising, classical respiratory mechanics variables such plateau and driving airway pressure, and respiratory system compliance are easily implementable and may allow frequent assessment.
Acknowledgements
None.
Footnote
Conflicts of Interest: The authors have no conflicts of interest to declare.
References
- Brodie D, Bacchetta M. Extracorporeal membrane oxygenation for ARDS in adults. N Engl J Med 2011;365:1905-14. [Crossref] [PubMed]
- ARDS Definition Task Force, Ranieri V, Rubenfeld G, et al. Acute respiratory distress syndrome: the Berlin Definition. JAMA 2012;307:2526-33. [PubMed]
- Hill JD, O’Brien TG, Murray JJ, et al. Prolonged extracorporeal oxygenation for acute post-traumatic respiratory failure (shock-lung syndrome). Use of the Bramson membrane lung. N Engl J Med 1972;286:629-34. [Crossref] [PubMed]
- Lee JJ, Hwang SM, Ko JH, et al. Efficacy of veno-venous extracorporeal membrane oxygenation in severe acute respiratory failure. Yonsei Med J 2015;56:212-9. [Crossref] [PubMed]
- Peek GJ, Mugford M, Tiruvoipati R, et al. Efficacy and economic assessment of conventional ventilatory support versus extracorporeal membrane oxygenation for severe adult respiratory failure (CESAR): a multicentre randomised controlled trial. Lancet 2009;374:1351-63. [Crossref] [PubMed]
- Combes A, Hajage D, Capellier G, et al. Extracorporeal Membrane Oxygenation for Severe Acute Respiratory Distress Syndrome (EOLIA). N Engl J Med 2018;378:1965-75. [Crossref] [PubMed]
- Combes A, Bacchetta M, Brodie D, et al. Extracorporeal membrane oxygenation for respiratory failure in adults. Curr Opin Crit Care 2012;18:99-104. [Crossref] [PubMed]
- Pham T, Combes A, Roze H, et al. Extracorporeal membrane oxygenation for pandemic influenza A(H1N1)-induced acute respiratory distress syndrome: a cohort study and propensity-matched analysis. Am J Respir Crit Care Med 2013;187:276-85. [Crossref] [PubMed]
- Noah MA, Peek GJ, Finney SJ, et al. Referral to an extracorporeal membrane oxygenation center and mortality among patients with severe 2009 influenza A(H1N1). JAMA 2011;306:1659-68. [Crossref] [PubMed]
- Serpa Neto A, Schmidt M, Azevedo LCP, et al. Associations between ventilator settings during extracorporeal membrane oxygenation for refractory hypoxemia and outcome in patients with acute respiratory distress syndrome: a pooled individual patient data analysis. Intensive Care Med 2016;42:1672-84. [Crossref] [PubMed]
- Frenckner B, Palmér P, Lindén V. Extracorporeal respiratory support and minimally invasive ventilation in severe ARDS. Minerva Anestesiol 2002;68:381-6. [PubMed]
- Marhong JD, Telesnicki T, Munshi L, et al. Mechanical ventilation during extracorporeal membrane oxygenation an international survey. Ann Am Thorac Soc 2014;11:956-61. [Crossref] [PubMed]
- Marhong JD, Munshi L, Detsky M, et al. Mechanical ventilation during extracorporeal life support (ECLS): a systematic review. Intensive Care Med 2015;41:994-1003. [Crossref] [PubMed]
- Schmidt M, Pellegrino V, Combes A, et al. Mechanical ventilation during extracorporeal membrane oxygenation. Crit Care 2014;18:203. [Crossref] [PubMed]
- Schmidt M, Stewart C, Bailey M, et al. Mechanical ventilation management during extracorporeal membrane oxygenation for acute respiratory distress syndrome: a retrospective international multicenter study. Crit Care Med 2015;43:654-64. [Crossref] [PubMed]
- Pesenti A, Carlesso E, Langer T, et al. Ventilation during extracorporeal support. Med Klin Intensivmed Notfmed 2018;113:26-30. [Crossref] [PubMed]
- Bein T, Weber-Carstens S, Goldmann A, et al. Lower tidal volume strategy (≈3 ml/kg) combined with extracorporeal CO2removal versus ‘conventional’ protective ventilation (6 ml/kg) in severe ARDS: The prospective randomized Xtravent-study. Intensive Care Med 2013;39:847-56. [Crossref] [PubMed]
- Brogan TV, Thiagarajan RR, Rycus PT, et al. Extracorporeal membrane oxygenation in adults with severe respiratory failure: a multi-center database. Intensive Care Med 2009;35:2105-14. [Crossref] [PubMed]
- Patroniti N, Zangrillo A, Pappalardo F, et al. The Italian ECMO network experience during the 2009 influenza A (H1N1) pandemic: preparation for severe respiratory emergency outbreaks. Intensive Care Med 2011;37:1447-57. [Crossref] [PubMed]
- Holzgraefe B, Broomé M, Kalzén H, et al. Extracorporeal membrane oxygenation for pandemic H1N1 2009 respiratory failure. Minerva Anestesiol 2010;76:1043-51. [PubMed]
- Kipping V, Weber-Carstens S, Lojewski C, et al. Prone position during ECMO is safe and improves oxygenation. Int J Artif Organs 2013;36:821-32. [Crossref] [PubMed]
- Bonacchi M, Harmelin G, Peris A, et al. A novel strategy to improve systemic oxygenation in venovenous extracorporeal membrane oxygenation: the ‘‘v-configuration’’. J Thorac Cardiovasc Surg 2011;142:1197-204. [Crossref] [PubMed]
- Kon ZN, Bittle GJ, Pasrija C, et al. Venovenous Versus Venoarterial Extracorporeal Membrane Oxygenation for Adult Patients With Acute Respiratory Distress Syndrome Requiring Precannulation Hemodynamic Support: A Review of the ELSO Registry. Ann Thorac Surg 2017;104:645-9. [Crossref] [PubMed]
- Hopkins RO, Weaver LK, Collingridge D, et al. Two-year cognitive, emotional, and quality-of-life outcomes in acute respiratory distress syndrome. Am J Respir Crit Care Med 2005;171:340-7. [Crossref] [PubMed]
- Brun-Buisson C, Pimentel J, Lewandowski K, et al. Epidemiology and outcome of acute lung injury in European intensive care units. Results from the ALIVE study. Intensive Care Med 2004;30:51-61. [Crossref] [PubMed]
- Brown SM, Wilson EL, Presson AP, et al. Understanding patient outcomes after acute respiratory distress syndrome: identifying subtypes of physical, cognitive and mental health outcomes. Thorax 2017;72:1094-103. [Crossref] [PubMed]
- Davydow DS, Desai SV, Needham DM, et al. Psychiatric morbidity in survivors of the acute respiratory distress syndrome: a systematic review. Psychosom Med 2008;70:512-9. [Crossref] [PubMed]
- Pfoh ER, Chan KS, Dinglas VD, et al. Cognitive screening among acute respiratory failure survivors: a cross-sectional evaluation of the Mini-Mental State Examination. Crit Care 2015;19:220. [Crossref] [PubMed]
- Herridge MS, Tansey CM, Matte A, et al. Functional disability 5 years after acute respiratory distress syndrome. N Engl J Med 2011;364:1293-304. [Crossref] [PubMed]
- Rubenfeld GD, Herridge MS. Epidemiology and outcomes of acute lung injury. Chest 2007;131:554-62. [Crossref] [PubMed]
- Slutsky AS, Ranieri VM. Ventilator-induced lung injury. N Engl J Med 2013;369:2126-36. [Crossref] [PubMed]
- Gattinoni L, Tonetti T, Cressoni M, et al. Ventilator-related causes of lung injury: the mechanical power. Intensive Care Med 2016;42:1567-75. [Crossref] [PubMed]
- Tremblay LN, Slutsky AS. Ventilator-induced injury: from barotrauma to biotrauma. Proc Assoc Am Physicians 1998;110:482-8. [PubMed]
- Tonetti T, Vasques F, Rapetti F, et al. Driving pressure and mechanical power: new targets for VILI prevention. Ann Transl Med 2017;5:286. [Crossref] [PubMed]
- Amato MB, Meade MO, Slutsky AS, et al. Driving Pressure and Survival in the Acute Respiratory Distress Syndrome. N Engl J Med 2015;372:747-55. [Crossref] [PubMed]
- Protti A, Andreis DT, Monti M, et al. Lung stress and strain during mechanical ventilation: Any difference between statics and dynamics? Crit Care Med 2013;41:1046-55. [Crossref] [PubMed]
- Chiumello D, Carlesso E, Cadringher P, et al. Lung stress and strain during mechanical ventilation for acute respiratory distress syndrome. Am J Respir Crit Care Med 2008;178:346-55. [Crossref] [PubMed]
- Ranieri VM, Giunta F, Suter PM, et al. Mechanical ventilation as a mediator of multisystem organ failure in acute respiratory distress syndrome. JAMA 2000;284:43-4. [Crossref] [PubMed]
- Parsons PE, Eisner MD, Thompson BT, et al. Lower tidal volume ventilation and plasma cytokine markers of inflammation in patients with acute lung injury. Crit Care Med 2005;33:1-6; discussion 230-2. [Crossref] [PubMed]
- Munshi L, Kiss A, Cypel M, et al. Oxygen thresholds and mortality during extracorporeal life support in adult patients. Crit Care Med 2017;45:1997-2005. [Crossref] [PubMed]
- Cashen K, Reeder R, Dalton HJ, et al. Hyperoxia and hypocapnia during pediatric extracorporeal membrane oxygenation: associations with complications, mortality, and functional status among survivors. Pediatr Crit Care Med 2018;19:245-53. [Crossref] [PubMed]
- Acute Respiratory Distress Syndrome Network, Brower RG, Matthay MA, et al. Ventilation with lower tidal volumes as compared with traditional tidal volumes for acute lung injury and the acute respiratory distress syndrome. The Acute Respiratory Distress Syndrome Network. N Engl J Med 2000;342:1301-8. [Crossref] [PubMed]
- Del Sorbo L, Goligher EC, McAuley DF, et al. Mechanical ventilation in adults with acute respiratory distress syndrome. Summary of the experimental evidence for the clinical practice guideline. Ann Am Thorac Soc 2017;14:S261-S270. [Crossref] [PubMed]
- Munshi L, Del Sorbo L, Adhikari NKJ, et al. Prone position for Acute Respiratory Distress Syndrome. A systematic review and meta-analysis. Ann Am Thorac Soc 2017;14:S280-8. [Crossref] [PubMed]
- Guérin C, Reignier J, Richard JC. Prone positioning in severe acute respiratory distress syndrome. N Engl J Med 2013;368:2159-68. [Crossref] [PubMed]
- Papazian L, Forel JM, Gacouin A, et al. Neuromuscular blockers in early acute respiratory distress syndrome. N Engl J Med 2010;363:1107-16. [Crossref] [PubMed]
- Forel JM, Roch A, Marin V, et al. Neuromuscular blocking agents decrease inflammatory response in patients presenting with acute respiratory distress syndrome. Crit Care Med 2006;34:2749-57. [Crossref] [PubMed]
- Walkey AJ, Del Sorbo L, Hodgson CL, et al. Higher PEEP versus lower PEEP strategies for patients with acute respiratory distress syndrome: A systematic review and meta-analysis. Ann Am Thorac Soc 2017;14:S297-S303. [Crossref] [PubMed]
- Goligher EC, Hodgson CL, Adhikari NKJ, et al. Lung Recruitment Maneuvers for Adult Patients with Acute Respiratory Distress Syndrome. A Systematic Review and Meta-Analysis. Ann Am Thorac Soc 2017;14:S304-11. [Crossref] [PubMed]
- Zapol WM, Snider MT, Hill JD, et al. Extracorporeal membrane oxygenation in severe acute respiratory failure. A randomized prospective study. JAMA 1979;242:2193-6. [Crossref] [PubMed]
- Morris AH, Wallace CJ, Menlove RL, et al. Randomized clinical trial of pressure-controlled inverse ratio ventilation and extracorporeal CO2 removal for adult respiratory distress syndrome. Am J Respir Crit Care Med 1994;149:295-305. [Crossref] [PubMed]
- Bein T, Zimmermann M, Hergeth K, et al. Pumpless extracorporeal removal of carbon dioxide combined with ventilation using low tidal volume and high positive end-expiratory pressure in a patient with severe acute respiratory distress syndrome. Anaesthesia 2009;64:195-8. [Crossref] [PubMed]
- Terragni PP, Del Sorbo L, Mascia L, et al. Tidal volume lower than 6 ml/kg enhances lung protection: role of extracorporeal carbon dioxide removal. Anesthesiology 2009;111:826-35. [Crossref] [PubMed]
- Fanelli V, Ranieri MV, Mancebo J, et al. Feasibility and safety of low-flow extracorporeal carbon dioxide removal to facilitate ultra-protective ventilation in patients with moderate acute respiratory distress sindrome. Crit Care 2016;20:36. [Crossref] [PubMed]
- Brenner K, Abrams DC, Agerstrand CL, et al. Extracorporeal carbon dioxide removal for refractory status asthmaticus: experience in distinct exacerbation phenotypes. Perfusion 2014;29:26-8. [Crossref] [PubMed]
- Burki NK, Mani RK, Herth FJF, et al. A novel extracorporeal CO2 removal system. Chest 2013;143:678-86. [Crossref] [PubMed]
- Javidfar J, Bacchetta M. Bridge to lung transplantation with extracorporeal membrane oxygenation support. Curr Opin Organ Transplant 2012;17:496-502. [Crossref] [PubMed]
- Gattinoni L, Tonetti T, Quintel M. How best to set the ventilator on extracorporeal membrane lung oxygenation. Curr Opin Crit Care 2017;23:66-72. [Crossref] [PubMed]
- ELSO. Extracorporeal Life Support Organization (ELSO) Guidelines for Adult Respiratory Failure v1.4. 2017. Available online: https//www.elso.org
- Zanella A, Mojoli F, Castagna L, et al. Respiratory Monitoring of the ECMO Patient. In: Sangalli F, Patroniti N, Pesenti A. editors. ECMO-Extracorporeal Life Support in Adults. Italia: Springer-Verlag, 2014.
- Franchineau G, Brechot N, Lebreton G, et al. Bedside contribution of electrical impedance tomography to setting positive end-expiratory pressure for extracorporeal membrane oxygenation-treated patients with severe acute respiratory distress syndrome. Am J Respir Crit Care Med 2017;196:447-57. [Crossref] [PubMed]
- Talmor D, Sarge T, Malhotra A, et al. Mechanical ventilation guided by esophageal pressure in acute lung injury. N Engl J Med 2008;359:2095-104. [Crossref] [PubMed]
- Akoumianaki E, Maggiore SM, Valenza F, et al. The application of esophageal pressure measurement in patients with respiratory failure. Am J Respir Crit Care Med 2014;189:520-31. [Crossref] [PubMed]
- Yoshida T, Amato MBP, Grieco DL, et al. Esophageal Manometry and Regional Transpulmonary Pressure in Lung Injury. Am J Respir Crit Care Med 2018;197:1018-26. [Crossref] [PubMed]
- Grasso S, Terragni P, Birocco A, et al. ECMO criteria for influenza A (H1N1)-associated ARDS: role of transpulmonary pressure. Intensive Care Med 2012;38:395-403. [Crossref] [PubMed]