Immunotherapeutic strategies in patients with advanced head and neck squamous cell carcinoma
Most head and neck squamous cell carcinoma (HNSCC) patients present with advanced-stage disease often with significant rates of local failure and distant metastases (1). Over the past two decades, the survival rates of HNSCC patients have not been altered significantly by standard therapy (2). Immune dysfunction has been demonstrated in patients with HNSCC and this was correlated with either a defect of their antitumor immune responses and/or tumor progression and relapse (3). Immunotherapy has emerged as a promising treatment approach to improve such immune dysfunction. Interventions at immune checkpoints like CTLA-4, PD-1 and others are aimed at reconstituting immunosurveillance or T-cell mediated elimination of malignant cells. The effector and regulatory T cell compartments are targeted to resurrect an efficient T cells function. The assumption is that specific antigens are targeted and much debate is ongoing on what the most important target antigens may be mutated neo-antigens or constitutive cellular antigens like the cancer testis family of antigens (CTA) which are expressed preferentially in cancer cells like the MAGE family of antigens, NY-ESO-1 and others (4).
The PD-1 pathway, an important immune checkpoint, has been demonstrated to be an effective target in HNSCC (5-8). Pembrolizumab is an anti-PD-1 antibody that was approved for patients with HNSCC (recurrent or metastatic) with disease progression on or after platinum-containing chemotherapy (9). The efficacy and safety outcomes from a phase Ib multi-cohort KEYNOTE-012 trial (ClinicalTrials.gov, NCT01848834) were the basis of approval of pembrolizumab in advanced solid tumors. In this trial study, Tahara et al. (9) recently reported their results from a subset of 26 patients with recurrent/metastatic HNSCC from the Asia-Pacific region. In these patients, durable anti-tumor response was observed with limited side effects. Five patients achieved a confirmed partial response (PR) with an overall response rate (ORR) of 19%; another 8 (31%) patients achieved stable disease (SD); and 12 (46%) patients had progressive disease (PD). No patients achieved a complete response. It is important to mention here that most of these patients (73%) had a history of smoking which is a main cause of somatic mutations in cancer (10) since cigarette smoke has over 60 carcinogens (11). In addition, 92% of the patients received prior platinum treatment such as cisplatin an alkylating agent also known to be a mutagenic compound (12).
Head and neck cancer has a high prevalence of somatic mutations like other cancers such as lung cancer (see Figure 1) (10). “The genetic damage that on the one hand leads to oncogenic outgrowth can also be targeted by the immune system to control malignancies” (13). Today technical advances allow the analysis of patient specific neoantigens generated due to tumor-specific mutations. On the other hand, immune response against these neoantigens can provide an extensive insight into the immune landscape of each patient. Recent studies have evidenced that neoantigen-specific cytotoxic T cells form the major dynamic element of a successful cancer immunotherapy. Therefore, strategies to target the patient tumor-specific neoantigens repertoire have been recently suggested (13). In this, (I) immunotherapy, using checkpoint blockade such as pembrolizumab, may be given in combination with radiotherapy to induce tumor cell destruction and enhance expression of neoantigens (14); (II) potential neoantigens should be identified, and a patient-specific vaccine is produced to be given together with an adjuvant and a checkpoint blockade antibody; (III) or neoantigens-specific T cells should be generated and expanded in vitro and given together with a checkpoint blockade antibody.
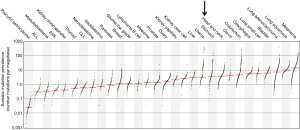
These personalized/precised cancer immunotherapies have been recently explored with promising clinical outcomes (Figure 2). Ott et al. (15) investigated whether vaccination with neoantigens of melanoma patients, will expand pre-existing neoantigen-specific T cells and also induce a repertoire of new T cell populations. They had shown that a vaccine targeting up to 20 personal tumour neoantigens was able to induce polyfunctional CD4+ and CD8+ T cells that discriminated wild-type from mutated antigens and were able to recognize autologous tumour. Importantly, of the 6 vaccinated patients, 4 had no recurrence at 25 months after vaccination, while 2 with recurrent disease were subsequently treated with pembrolizumab and showed complete tumor regression and this was associated with the expansion of the neoantigen-specific T cells repertoire in such patients. Zacharakis et al. (16) have recently reported a patient with metastatic breast cancer who was treated with tumor-infiltrating lymphocytes expanded against mutant versions of 4 proteins (SLC3A2, KIAA0368, CADPS2 and CTSB). They have shown that adoptive transfer of these TILs along with IL-2 and pembrolizumab was able to mediate a complete durable regression of metastatic breast cancer for >22 months and indicating a novel approach for the treatment of metastatic breast cancer patients via immunotherapy.
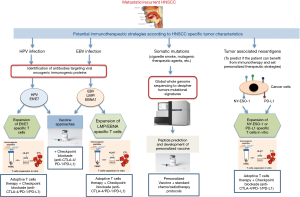
Tahara et al. (9) reported that 8% of the patients included in their trial had human papillomavirus (HPV)-associated disease. It has been demonstrated that comparison of head and neck cancer patients; HPV-positive tumors vs. HPV-negative showed higher response rates (84% vs. 57%) as well as two-year overall survival rates (95% vs. 62%) after chemo-radiation therapy (17). In this setting, the chemo-radiation therapy may induce tumor cell destruction that enhanced exposure to HPV-derived antigens to be recognized by the patients’ immune system. Theoretically, the viral HPV E6 and E7 oncoproteins, constitutively expressed by cancer cells are candidates of tumor regression antigens due to their immunologically foreign status. However, targeting such antigens using therapeutic vaccines have not been effective (18). Nevertheless, incorporating chemotherapy with therapeutic vaccines was able to reduce myeloid-derived suppressor cells and to boost immunogenicity (19). Moreover, the response rates to pembrolizumab appeared to be similar in patients with HPV-positive and HPV-negative HNSCC (5). Indeed, a recent study by Stevanovic et al. (20) revealed that targeting of both viral and non-viral tumor antigens by adoptive transfer of expanded tumor infiltrating lymphocytes led to complete regression of metastatic HPV+ cervical cancer patients. They have shown that instead of viral antigens, the immunodominant T cell responses were directed against cancer germline antigens or mutated neoantigens. Moreover, the T cells that targeted the HPV antigens did not exhibit superior in vivo expansion. Interestingly, they have shown that both viral and non-viral antigen–specific T cells predominantly located in the PD-1–expressing T cell compartment. This suggests that PD-1 blockade may unleash diverse antitumor T cell responses. However, it has been shown that most HNSCC patients did not respond to PD-1 blockade monotherapy which aimed to boost pre-existing anti-tumor immune responses (6,9). Therefore, a new approach of targeting both viral and non-viral antigens may be suggested as a therapeutic vaccine in combination with immune checkpoint inhibitors blockade in HNSCC patient (Figure 2).
It would be interesting to know whether EBV was associated with HNSCC in Tahara et al. (9) trial. EBV has been identified as a key player in the pathogenesis of HNSCC (reviewed in (21) and therefore should be considered as an immunotherapeutic target. In particular, EBV viral antigens; LMP1, LMP2, and EBNA1 have been documented as modulators of major factors that are involved in malignant transformation. Recently, Smith et al. (22) reported on the targeting of LMP1/2 and EBNA1 antigens in HNSCC patients via adoptive T cell therapy. They documented on the strategy of expanding LMP/EBNA1-specific T cells from low frequency precursors using adenovirus AdE1-LMP poly vector. Interesting results were observed with successful generation of autologous LMP/EBNA1-specific T cells exhibiting enhanced frequency/efficacy in EBV positive patients. Furthermore, diseases stabilization was observed in such patients indicating the importance of allogeneic “off-the-shelf” LMP/EBNA1-specific T cells for their clinical use in the treatment of HNSCC. However, the expanded EBV-specific T cells exhibited upregulation of PD-1 antigen. In studies on HNSCC tumor cells, PD-L1 expression has been associated with poor outcome and as such this is a critical factor that needs to be taken into consideration in adoptive T cell therapy as it may affect the anti-tumor activity of EBV specific T cells (23). Therefore, approaches to improve the antitumor activity of EBV-specific T cells such as combination therapies that include CTLA-4 or PD-1/PD-L1 blockade are being developed (Figure 2). Recently, vaccination has recently emerged as a promising and effective cancer immunotherapy modality to treat EBV-associated HNSCC, specifically for nasopharyngeal cancers (NPC). To this end, the role and efficacy of therapeutic vaccines against EBV-associated NPCs have been tested in a number of preclinical and clinical trials that showed promising results [reviewed in (21) and Figure 2]. Interestingly, EBNA1 and LMP2 EBV-associated proteins, due to their ability to cause latent infection and immunological competence, have been identified as the most attractive targets for vaccination strategies in NPC.
Another tumor antigen that can be considered as immunotherapeutic target in HNSCC is the cancer testis antigen NY-ESO-1. This is a highly immunogenic antigen that induces both antigen specific antibody and T cell responses in HNSCC patients (24). As such, it may represent as an attractive target for immunotherapy in HNSCC, due to its high tumor-specificity and immunogenicity. We have recently shown the correlation of the clinical responsiveness to anti-PD-1 (nivolumab) treatment in a patient with recurrent HNSCC and an integrated immune response to the NY-ESO-1 antigen (25). In this, enhanced NY-ESO-1-specific T cell response was observed after anti-PD-1 treatment that declined dramatically after stopping anti-PD-1 treatment. Interestingly, two cytokines/chemokines involved in immune activation (IL-10 and CX3CL) were upregulated after nivolumab treatment; and were reduced at progression. Whereas two other cytokines/chemokines contributing to immune inhibition (IL-6 and IL-8) were downregulated after nivolumab treatment and were increased at progression (25). It has been suggested that the NY-ESO-1 humoral and cellular responses as well as the cytokine/chemokine profile should be able to potentially identify favorable response to PD-1 blockade in HNSCC patients. Possibly, clinical protocols should be developed to expand existing anti-NY-ESO-1-specific T cells to be used in combination with other immune checkpoint inhibitors blockade (Figure 2).
Tahara et al. (9) reported that 85% of the patients included in their trial had tumors that expressed the membrane bound PD-L1 (mPD-L1) molecules. It has been demonstrated that the expression of mPD-L1 was correlated with the soluble form of this marker (sPD-L1) (26). In addition, a recent meta-analysis study confirmed that a high level of sPD-L1 in the peripheral blood was significantly correlated with poor prognosis (27). The result of this study showed that cancer patients with high levels of sPD-L1 had a shorter overall survival than those with low levels of sPD-L1 and suggested that high levels of sPD-L1 may serve as a prognostic biomarker for the identification of patients with worse prognosis. In another study, treatment with immune checkpoint blockade resulted in high frequency of circulating sPD-L1 levels (28). In this, patients with long term or delayed increase in sPD-L1 correspond with clinically beneficial outcomes. It has been established that sPD-L1 is able to maintain its biological activity, and when binding to its PD-1 receptor, can still activate the PD-1/PD-L1 pathway and act as a stop signal for T cell activation. On the basis of such postulations, it has been considered that sPD-L1 in peripheral blood might be able to specifically bind to PD-1 on the tumoricidal cytotoxic T lymphocytes before they reach the tumor site and hence suppressing T cell activity and systemically damp the antitumor immune response (29). Therefore, designing novel strategies to block circulating sPD-L1 before anti-PD-L1 antibody treatment, should preserve the anti-PD-1-mediated immune response and improve the overall survival of cancer patients eligible for such treatment. Recently, Chen et al. (30) reported on the expression level of PD-L1 on circulating extracellular vesicles in melanoma patients during pembroluzimab treatment. The results showed that circulating exosomal PD-L1 was significantly higher in patients who failed to respond to treatment. They have shown that stimulation with IFN-γ was able to increase the levels of PD-L1 on these vesicles that suppress the function of CD8 T cells and facilitate tumour growth (30). Their study offers a foundation for development of circulating exosomal PD-L1 to predict the clinical outcomes of anti-PD-1 therapy and also highlights potential causes for the failure of anti-PD-1 therapy by many patients. Another strategy is to use PD-L1-specific T cells to eliminate PD-L1-expressing tumors. Indeed, it is well documented that PD-L1-specific T cell responses occur at higher frequencies in peripheral blood in cancer patients as compared to healthy donors and to lyse PD-L1-expressing cancer cells (31). Therefore, targeting immune regulation to enhance the immunogenicity of immunotherapeutic agents via expansion of by PD-L1-specific T cells is an attractive approach for therapeutics (32). Furthermore, combined therapy that includes vaccination with PD-1 pathway blockade should be easily implementable and synergistic (Figure 2). In this, antibody blocking of the PD-L1 should make the PD-L1-expressing cancer cells more susceptible targets for vaccine-induced T cells.
Future clinical trials should be explored to compare responders versus non-responders cohort with respect to preexisting humoral and/or cellular immune responses to antigens expressed in tumors.
Acknowledgements
We would like to thank Prof. Karl Richard Alexander Knuth for his input and the critical reviewing and editing this editorial.
Footnote
Conflicts of Interest: The authors have no conflicts of interest to declare.
References
- Rottey S, Madani I, Deron P, et al. Modern treatment for nasopharyngeal carcinoma: current status and prospects. Curr Opin Oncol 2011;23:254-8. [Crossref] [PubMed]
- Hoffmann TK, Bier H, Whiteside TL. Targeting the immune system: novel therapeutic approaches in squamous cell carcinoma of the head and neck. Cancer Immunol Immunother 2004;53:1055-67. [Crossref] [PubMed]
- Economopoulou P, Perisanidis C, Giotakis EI, et al. The emerging role of immunotherapy in head and neck squamous cell carcinoma (HNSCC): anti-tumor immunity and clinical applications. Ann Transl Med 2016;4:173. [Crossref] [PubMed]
- Scanlan MJ, Simpson AJ, Old LJ. The cancer/testis genes: review, standardization, and commentary. Cancer Immun 2004;4:1. [PubMed]
- Seiwert TY, Burtness B, Mehra R, et al. Safety and clinical activity of pembrolizumab for treatment of recurrent or metastatic squamous cell carcinoma of the head and neck (KEYNOTE-012): an open-label, multicentre, phase 1b trial. Lancet Oncol 2016;17:956-65. [Crossref] [PubMed]
- Chow LQM, Haddad R, Gupta S, et al. Antitumor Activity of Pembrolizumab in Biomarker-Unselected Patients With Recurrent and/or Metastatic Head and Neck Squamous Cell Carcinoma: Results From the Phase Ib KEYNOTE-012 Expansion Cohort. J Clin Oncol 2016;34:3838-45. [Crossref] [PubMed]
- Ferris RL, Blumenschein G Jr, Fayette J, et al. Nivolumab for Recurrent Squamous-Cell Carcinoma of the Head and Neck. N Engl J Med 2016;375:1856-67. [Crossref] [PubMed]
- Bauml J, Seiwert TY, Pfister DG, et al. Pembrolizumab for Platinum- and Cetuximab-Refractory Head and Neck Cancer: Results From a Single-Arm, Phase II Study. J Clin Oncol 2017;35:1542-9. [Crossref] [PubMed]
- Tahara M, Muro K, Hasegawa Y, et al. Pembrolizumab in Asia-Pacific patients with advanced head and neck squamous cell carcinoma: Analyses from KEYNOTE-012. Cancer Sci 2018;109:771-6. [Crossref] [PubMed]
- Alexandrov LB, Nik-Zainal S, Wedge DC, et al. Signatures of mutational processes in human cancer. Nature 2013;500:415-21. [Crossref] [PubMed]
- Pfeifer GP, Denissenko MF, Olivier M, et al. Tobacco smoke carcinogens, DNA damage and p53 mutations in smoking-associated cancers. Oncogene 2002;21:7435-51. [Crossref] [PubMed]
- Hunter C, Smith R, Cahill DP, et al. A hypermutation phenotype and somatic MSH6 mutations in recurrent human malignant gliomas after alkylator chemotherapy. Cancer Res 2006;66:3987-91. [Crossref] [PubMed]
- Schumacher TN, Schreiber RD. Neoantigens in cancer immunotherapy. Science 2015;348:69-74. [Crossref] [PubMed]
- Demaria S, Pilones KA, Vanpouille-Box C, et al. The optimal partnership of radiation and immunotherapy: from preclinical studies to clinical translation. Radiat Res 2014;182:170-81. [Crossref] [PubMed]
- Ott PA, Hu Z, Keskin DB, et al. An immunogenic personal neoantigen vaccine for patients with melanoma. Nature 2017;547:217-21. [Crossref] [PubMed]
- Zacharakis N, Chinnasamy H, Black M, et al. Immune recognition of somatic mutations leading to complete durable regression in metastatic breast cancer. Nat Med 2018;24:724-30. [Crossref] [PubMed]
- Fakhry C, Westra WH, Li S, et al. Improved survival of patients with human papillomavirus-positive head and neck squamous cell carcinoma in a prospective clinical trial. J Natl Cancer Inst 2008;100:261-9. [Crossref] [PubMed]
- van Poelgeest MI, Welters MJ, van Esch EM, et al. HPV16 synthetic long peptide (HPV16-SLP) vaccination therapy of patients with advanced or recurrent HPV16-induced gynecological carcinoma, a phase II trial. J Transl Med 2013;11:88. [Crossref] [PubMed]
- Welters MJ, van der Sluis TC, van Meir H, et al. Vaccination during myeloid cell depletion by cancer chemotherapy fosters robust T cell responses. Sci Transl Med 2016;8:334ra52. [Crossref] [PubMed]
- Stevanović S, Pasetto A, Helman SR, et al. Landscape of immunogenic tumor antigens in successful immunotherapy of virally induced epithelial cancer. Science 2017;356:200-5. [Crossref] [PubMed]
- Fernandes Q, Merhi M, Raza A, et al. Role of Epstein–Barr Virus in the Pathogenesis of Head and Neck Cancers and Its Potential as an Immunotherapeutic Target. Frontiers in Oncology 2018;8:257. [Crossref] [PubMed]
- Smith C, Lee V, Schuessler A, et al. Pre-emptive and therapeutic adoptive immunotherapy for nasopharyngeal carcinoma: Phenotype and effector function of T cells impact on clinical response. Oncoimmunology 2017;6:e1273311. [Crossref] [PubMed]
- Zhang J, Fang W, Qin T, et al. Co-expression of PD-1 and PD-L1 predicts poor outcome in nasopharyngeal carcinoma. Med Oncol 2015;32:86. [Crossref] [PubMed]
- Fujita S, Wada H, Jungbluth AA, et al. NY-ESO-1 expression and immunogenicity in esophageal cancer. Clin Cancer Res 2004;10:6551-8. [Crossref] [PubMed]
- Merhi M, Raza A, Inchakalody VP, et al. Squamous Cell Carcinomas of the Head and Neck Cancer Response to Programmed Cell Death Protein-1 Targeting and Differential Expression of Immunological Markers: A Case Report. Front Immunol 2018;9:1769. [Crossref] [PubMed]
- Shigemori T, Toiyama Y, Okugawa Y, et al. Soluble PD-L1 Expression in Circulation as a Predictive Marker for Recurrence and Prognosis in Gastric Cancer: Direct Comparison of the Clinical Burden Between Tissue and Serum PD-L1 Expression. Ann Surg Oncol 2019;26:876-83. [PubMed]
- Wei W, Xu B, Wang Y, et al. Prognostic significance of circulating soluble programmed death ligand-1 in patients with solid tumors: A meta-analysis. Medicine (Baltimore) 2018;97:e9617. [Crossref] [PubMed]
- Zhou J, Mahoney KM, Giobbie-Hurder A, et al. Soluble PD-L1 as a Biomarker in Malignant Melanoma Treated with Checkpoint Blockade. Cancer Immunol Res 2017;5:480-92. [Crossref] [PubMed]
- Freeman GJ, Sharpe AH, Kuchroo VK. Protect the killer: CTLs need defenses against the tumor. Nat Med 2002;8:787-9. [Crossref] [PubMed]
- Chen G, Huang AC, Zhang W, et al. Exosomal PD-L1 contributes to immunosuppression and is associated with anti-PD-1 response. Nature 2018;560:382-6. [Crossref] [PubMed]
- Munir S, Andersen GH, Met O, et al. HLA-restricted CTL that are specific for the immune checkpoint ligand PD-L1 occur with high frequency in cancer patients. Cancer Res 2013;73:1764-76. [Crossref] [PubMed]
- Munir Ahmad S, Martinenaite E, Hansen M, et al. PD-L1 peptide co-stimulation increases immunogenicity of a dendritic cell-based cancer vaccine. Oncoimmunology 2016;5:e1202391. [Crossref] [PubMed]