Classification and differential diagnosis of Wilson’s disease
Introduction
Wilson’s disease often remains undetected due to its numerous symptoms which emerge at different times, as well as its individual character. Conversely, Wilson’s disease can also be misdiagnosed as a result of misinterpreting the medical findings. To begin with, the autosomal recessive defect of the hepatic copper transporter ATP7B (chromosome 13q14.3; ATP7B; WND gene) (1,2) causes biochemical deviations followed by the fluctuating occurrence of hepatic and extrapyramidal symptoms (EPS) as well as the impairment of other organs (3-6).
Taking the clinical manifestation into account, primarily between age 5 and 45 (6), in some cases as early as age one or until age 70 (7-9), differential diagnostic considerations are required, which include Wilson’s disease. At the same time, a nosological classification of Wilson’s disease can be used for the allocation of typical and ruling out of atypical findings, to make a tentative diagnosis (10). In addition to starting treatment at an early stage, it is also necessary, from a therapeutic point of view, to make a correct diagnosis to justify treatment, which involves adverse reactions (5,11). In the case of the exclusion of an autosomal recessive disorder of the hepatic copper transporter ATP7B, the clarification of differential diagnoses based on the existing findings is paramount.
In this overview, classification and differential diagnostic consideration are conducted based on the findings. Significant biochemical parameters and clinical cardinal symptoms of Wilson’s disease are taken into account. Age onset as well as the typical spectrum of hepatic or neurological findings serves isolating relevant differential diagnoses.
Classification of Wilson’s disease
Wilson’s disease is characterized by various manifestations as a result of numerous qualitative and quantitative symptoms, which emerge at different times (10,12). Taking the individuality of each patient into consideration, it is a matter of additionally determining the essentials which are consistent in many patients.
Classification by age of onset
Until puberty, gastrointestinal symptoms with hepatic or haemolytic findings are predominant (6,12,13). Consequently, an indistinct elevation of transaminases and bilirubin, icteric flare-ups, signs of a virus—negative acute hepatitis as well as hepatosplenomegaly should lead to the suspicion (4). Acute liver failure is also possible. Haematologically, the occurrence of a Coombs-negative haemolysis and unclear anaemia, leukopenia as well as thrombocytopaenia, are suspicious (4,14).
Without an exact age limit and after overcoming undetected bland gastrointestinal findings, symptoms affecting the central nervous system appear from puberty onwards with dysarthric, extrapyramidal and mental manifestations (6,12,15-17). Laboratory-chemical involvement of the liver (transaminases, synthesis parameters albumin and coagulation factors, cholinesterase, ammonia), change in the sonographic liver texture and a Kayser-Fleischer ring (KFR) can support the suspicion (4,18).
Classification by cardinal symptoms in clinical forms of progression (clinical variants)
Based on the clinical and pathological-anatomical phenomena, Konovalov probably provided the most precise classification in his monograph published in 1960 (19). Following this, five clinical variants were distinguished (Table 1), modified according to Lössner et al. 1980 (15) and Bachmann et al. 1988 (20) based on clinical aspects.
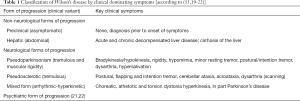
Full table
Some authors additionally divide the mixed form into a dystonic and a choreatic form (1,23). Psychiatric symptoms are also variable and include personality changes, behavioural disorders to criminal activities, cognitive disorders, depression and schizophrenia (6,21,24,25). They correlate more with the occurrence of neurological symptoms than with the existence of hepatic symptoms (22,23). In nearly 10%, Wilson’s disease manifests with psychiatric symptoms (24,26). An exclusive psychiatric presentation of Wilson’s disease is also reported in literature (21,22). Oder et al. [1993] recommend a classification of the neurological form of progression into three subgroups for this purpose based on extrapyramidal symptoms and cognitive as well as psychiatric disorders (27). On the other hand, only the (pragmatic simple) classification in neurological and non-neurological disease course is common in daily clinical practice.
A specific differential diagnosis can be made with an exact classification of Wilson’s disease based on the variable cardinal symptoms (10). Particularly in the case of unclear hepatic, extrapyramidal and psychiatric findings, Wilson’s disease must be included in differential diagnostic considerations at an early stage according to the knowledge of its clinical variants.
A quantitative assessment of the EPS (neurological score, Table 2) serves the documentation of the severity of the disease. Imaging results [cMRI, (18F)FDG-PET, 123J-Beta-CIT-SPECT and 123J-IBZM-SPECT], fine motor skills and electrophysiology support the classification in a neurological and non-neurological form of progression. They serve monitoring progression as well as defining differential diagnoses based on the profile of the diagnostic findings.
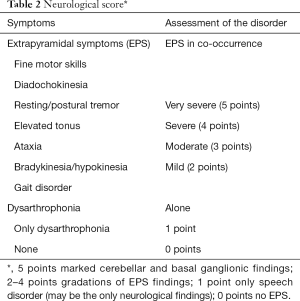
Full table
Differential diagnoses of Wilson’s disease related to findings
Copper metabolism disorders
Deviations in parameters of copper metabolism are not exclusively attributed to Wilson’s disease. Complex biochemical processes are involved in copper homoeostasis. Disruptions in these processes lead to separately described diseases contrary to the ATP7B defect. The following explanations regarding the physiology of copper regulation serve understanding them.
Discussion on standard values
Due to the mutation in the ATP7B gene, the balance of the key regulators for copper balance—the MNK and WND protein—is disrupted (28). The MNK protein (ATP7A, Menkes protein; located on the basal membrane of the small intestinal enterocytes) is responsible for intestinal copper absorption (29,30) and the WND protein (ATP7B; in hepatocytes) for the hepatobiliary excretion as well as copper uptake in the apoceruloplasmin in hepatocytes (31).
In the blood, reabsorbed copper binds loosely to albumin in relation of one atom per albumin molecule. In the process, special importance is attached to the histidine residue from the albumin for the complex formation with the copper atoms (32). Out of the amino acids in plasma, histidine has the highest affinity to copper (32) and transports it itself in small quantities as copper histidine (33). Furthermore, small quantities of copper are bound in the blood by transcuprein and other peptides (33). Ceruloplasmin, which is excreted into the serum, is formed with the covalent bond of 6–7 copper atoms in apoceruloplasmin (32). Approx. 95% of serum copper is transported to the other organs in this manner (34). There is only a small percentage of “free” serum copper.
The standard values in Table 3 apply as a means of orientation for intact ATPase 7B.
Given that ceruloplasmin represents the largest copper fraction in serum, the disruption of its synthesis due to a lack of copper uptake (ATPase 7B—defect) results in a severe decrease of the overall serum copper level. Merely marginal shortfalls of the reference range are not indicative of Wilson’s disease due to its broad spectrum. Free serum copper is higher because it is released from decomposing hepatocytes without a firm bond to other transport proteins. Another value is a matter of an (empirically) calculated value, which on its own is not very meaningful. This diagnostic finding can only be interpreted in a plausible manner in context with other findings.
Ceruloplasmin has a wide reference range and in the case of Wilson’s disease, is decreased to basal values (typically <0.05 g/L) [(36), own data]. The decrease of ceruloplasmin is not 100% sensitive or specific (36). Marginal decreases are often mistakenly considered an indicator for Wilson’s disease. However, these are alimentary, in the case of bile acid synthesis disorders or caused by kidney disorders or individually without pathological significance. Even in the case of heterozygous genetic carriers of the ATP7B mutation, there is still sufficient ceruloplasmin synthesis without the clinical manifestation of Wilson’s disease. Furthermore, aceruloplasminemia (see below) does not cause any symptoms related to Wilson’s disease (37). In conformance with the increase of free copper, daily renal excretion increases to >1 µmol/d. This parameter should be confirmed by being tested twice.
The liver copper content is considered pathological as of a dry weight >250 µg/g (12,38). A representative biopsy specimen is required for a definitive conclusion of the liver biopsy; if applicable, multiple biopsies as a result of non-homogeneous hepatic copper distribution must be conducted (5).
A distinctive pathological profile of the copper metabolism parameter can already be verified prior to the onset of hepatolenticular clinical cardinal symptoms based on the toxic effect of the copper. One single pathological finding with other normal values or merely marginal deviations is not plausible for Wilson’s disease. After clinical manifestation, the correlation to the cardinal symptoms (hepatopathy, EPS, KFR) must be tested, to support the validity of the diagnostic/laboratory tests (36).
Cellular enzymatic level
Bound to albumin, the copper is quickly transported to the liver and reaches the hepatocytes via an energy-dependent mechanism (hCtr1, high-affinity copper transporter) (31). Transport, excretion and storage, to maintain copper homoeostasis, are organized within the liver cells.
At the same time, glutathione is the most important cytosolic chelating agent; over 60% are in form of Cu(I)-GSH (14). Copper glutathione [Cu(I)-GSH] is the donator of copper to metallothionein and superoxide dismutase (31). Hepatic copper metallothionein serves as copper storage as well as copper supplier for other proteins (31). To prevent undesired toxic reactions triggered by copper ions, these are constantly escorted intracellularly by various chaperone proteins (chaperones such as HAH1, Cox17, CCS, Sco1/Sco2) (23,39,40).
Hepatic ATPase 7B acts as a central mechanism for copper homeostasis. It is an intracellular copper sensor with redistribution depending on the level (31). In the case of a low level of intracellular copper, the ATPase is located in the Golgi apparatus and communicates the copper uptake into enzymes containing copper, particularly in apoceruloplasmin (31,39). As soon as the copper level increases, it finds its way to the apical position, to ensure biliary copper excretion into the small ducts of the gall bladder (31,41). This complex function is disrupted by the mutations.
Two other intracellular proteins, XIAP (X-linked inhibitor of apoptosis, caspase inhibitor) and MURR1 (Copper Metabolism MURR1 Domain Protein 1, COMMD1), are involved in copper homeostasis (42,43). While MURR1 provokes vesicular compartmentalization and biliary excretion of copper (43), XIAP accelerates its proteasomal degradation through ubiquitination (42). The exact mechanism of the MURR1 effect is still unknown but an interaction with the ATP7B transporter is being discussed (44,45). MURR1 regulates the folding, stability and the proteasomal degradation of ATP7A, ATP7B as well as other proteins (45).
In regard to human pathogens, to date, the ATPases were known in this complex intracellular modulation. In 2012–2013, three new intracellular copper metabolism disorders were described; the MEDNIK syndrome, the Huppke-Brendel syndrome and chaperone deficiency CCS.
ATPases are enzymes (hydrolases) and simultaneously transport proteins, which use energy released through ATP hydrolysis, on a membrane (inside of a cell membrane, membrane-bound organelles) for active transport against a concentration gradient (46). They are substrate specific through a particular chemical and spatial structure and are distinguished in several types (ABC transporters, F-type, V-type, P-type, A-type, E-type among others) according to the substrate and occurrence in the cell or organisms (prokaryotes and/or eukaryotes, solely for bacteria or plants) (47). The formation of a phosphorylated intermediate is a characteristic feature of the P-type transporter, which also includes ATP7A and ATP7B (48).
The P-type ATPase 7B has substrate specificity for copper ions (47-51). Its structure is characterized by ATP binding sites, 8 transmembrane domains and amino-terminal by 6 copper-binding sequences (48,49). It is particularly found in the liver and kidneys, but to a small extent, also in the brain, lungs, placenta, skeletal muscle and pancreas (1,2,49-51). Its gene is located on the long arm of chromosome 13 (13q14-21-Locus) (52) and approx. 7.5 kilobases long. The encoded part for 1,411 amino acids is 4.3 kilobases allocated to 21 exons (33). Homozygous or compound heterozygous mutations lead to Wilson’s disease.
There is a strong correlation in the functionally significant regions between ATPase 7B and ATPase 7A, also a P-type ATPase with copper ion specificity (48,49,53,54). ATP7A is encoded x-chromosomal (Locus X 13.3). It is located in the intestine on the basal membrane of the enterocytes and responsible for copper absorption (23,49,51). Its defect causes Menkes disease (trichopoliodystrophy), which only occurs in boys (49). Disrupted copper-dependent enzymes in the CNS and connective tissue metabolism are responsible for the symptomology. Occipital horn syndrome (OHS; occurrence of occipital exostoses and calcifications) is considered a milder variant of the ATP7A defect (55) (Table 4).
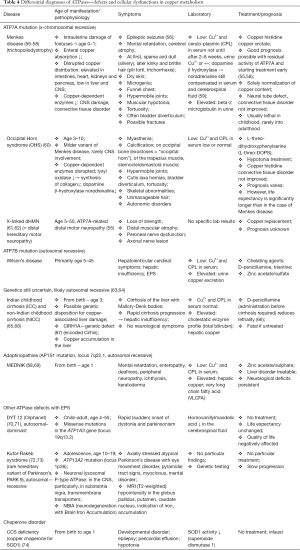
Full table
Remarkably, the ATP7A-associated distal motor neuropathy (X-linked dHMN) does not have any of the clinical or biochemical abnormalities of Menkes disease or the OHS. It resembles Charcot-Marie-Tooth disease type 2. This shows that the ATP7A plays a significant role in maintaining motor neurons. The still unknown pathomechanism of the ATP7A-associated distal motor neuropathy differs from the one in Menkes disease and OHS (55).
From other ATPase—defects, DYT 12 and the Kufor-Rakeb syndrome (PARK 9) with Parkinson’s disease or extrapyramidal symptoms must be considered in differential diagnoses (Table 4).
It was assumed that Indian Childhood Cirrhosis (ICC) was endemic and unique in India. However, this disease has meanwhile been documented in children on non-Indian heritage (Non-Indian Childhood Cirrhosis, NICC) (65). An autosomal recessive defect of the Cirhin encoding CIRH1A gene was described as North American Indian Childhood Cirrhosis (NAIC) (67). Brewer also recognizes the analogy of ICC to Tyrolean Infantile Cirrhosis (TIC), an epidemic liver disease in children in Austria (3).
Adaptor proteins (AP) regulate the transport of transmembrane proteins and the clathrin-coated vesicles between Golgi apparatus, endosomes and plasma membrane. AP1 is responsible for normal ATP7A, ATP7B and clathrin function. A mutation in the AP1 gene—complex subunit sigma 1A (AP1S1), leads to a dysfunction of the ATP7A and ATP7B despite intact enzyme activities (69). Their mislocation results in dysfunctions in copper metabolism, a reduction of copper-dependent enzymes as well as a lack of copper transport. Patients with MEDNIK syndrome (acronym—see Table 4, autosomal recessive inheritance) have combined clinical and biochemical symptoms for Menkes disease as well as Wilson’s disease (68).
The autosomal recessive mutation of the copper chaperone CCS (for SOD1) is another dysfunction of proteins for copper metabolism. CCS is responsible for escorting copper in an intracellular manner and for its delivery to Cu/Zn superoxide dismutase (SOD1) (Table 4). A lack of CCS leads to a defect of the SOD1 with a lethal outcome (74).
Alimentary disorder
Of the 2–4 mg of copper ingested daily with our food, 0.5–1.2 mg in total is reabsorbed in the upper segment of the small intestine; the rest leaves the body through our stool. As an integral part of at least 16 metalloproteins, copper has essential tasks, in particular in connective tissue metabolism, in the CNS and in haematopoiesis (75,76). Copper is also jointly responsible for enteral iron absorption (77).
Insufficient oral intake as well as malabsorption (e.g. as a result of bariatric surgery) are causes for a copper deficiency (78). An increased zinc uptake [through dietary supplements, denture adhesive cream (79)] also decreases the serum copper level (80). Taking valproic acid leads to a copper deficiency via a not yet clarified mechanism (81). A cause for copper deficiency cannot be found in approx. 20% of all patients; it is idiopathic.
Clinical manifestations of hypocupraemia are a sensorimotor polyneuropathy; damage to the optic nerve as well as the myelon with sensory ataxia and pyramidal tract signs (80,82,83). Blood work indicates microcytic hypochromic anaemia and there is cell dysplasia in the bone marrow (80,82,83). Anaemia, a low ceruloplasmin level as well as dysfunctions of the immune system (neutropenia), pigmentary abnormalities and disturbance of growth are also consequences of secondary iron deficiency (84). Moreover, osteoporosis as well as elevated glucose and cholesterol levels can occur.
A copper overload is rare, up to 10 mg/day are tolerated for longer periods of time. Larger doses of copper salts cause nausea, abdominal cramps and diarrhoea (85). High doses on a gram scale lead to liver and kidney damage, haemolysis, brain damage, coma and death (86,87).
Disorders in the formation of ceruloplasmin
Aceruloplasminemia and low ceruloplasmin
Ceruloplasmin (molecular weight of 151 kDa), an alpha-2-glycoprotein, is encoded on the long arm of chromosome 3 (CP gene, 3q23-q24) (33,34). It is primarily formed in the liver but also to a smaller extent in the brain and can transport up to 8 copper ions (Cu2+) per molecule. Approx. 95% of the serum copper is bound to ceruloplasmin and give it its bluish colour (88,89). In addition to the function as a copper transporter, it also has a copper-dependent oxidase activity (ferroxidase) (90). The iron oxidation of Fe2+ to Fe3+ enables its transport by transferrin. As an acute-phase protein, it acts as a strong antioxidant through inhibition of lipid peroxidation (88).
Aceruloplasminemia is a rare autosomal recessive neurodegenerative disease, which usually presents during late adulthood around age 50–60 (91). It belongs to the group of iron accumulation disorders (NBIA, Neurodegeneration with Brain Iron Accumulation) with iron accumulation in the brain, particularly in the basal ganglia and in the liver (92). There is a complete lack of ferroxidase activity in the case of the homozygous mutation (89). The serum indicates a low level of copper and iron with elevated ferritin. Renal copper excretion is normal (93). Neurologically, ataxia, dysarthria, hyperkinesia (tremor, chorea), dystonia as well as Parkinsonism accompanied by depression and cognitive disorders result (94). Anaemia, retinopathy, diabetes mellitus and in some cases, heart failure due to iron overload are internal characteristics (93). The prognosis is determined by heart failure.
Huppke-Brendel syndrome is an autosomal recessive defect of the SLC33A1 gene for an Acetyl-coA transporter. This leads to a dysfunction of posttranslational ceruloplasmin modification and its decreased secretion into the blood. Before reaching age one, this disease presents with developmental delay, hypotonia, hypacusis, cerebral atrophy, cataract and nystagmus without basal ganglia and liver involvement (95,96). Serum copper and ceruloplasmin levels are low (95,96). The prognosis is poor as there currently is no treatment (96).
The serum ceruloplasmin concentration is severely decreased in the case of Wilson’s disease and Menkes disease; also lower in the case of heterozygous trait carriers of Wilson’s disease (97). Homozygote causes a ceruloplasmin synthesis dysfunction in Wilson’s disease, so that there is inactive apoceruloplasmin without ferroxidase activity. On the other hand, the Menkes disease and OHS present a copper absorption disorder as cause for ceruloplasmin deficiency. Ceruloplasmin degradation also occurs in the case of hepatic insufficiency (hepatitis) and protein-losing enteropathy (97). Ceruloplasmin is physiologically low in infancy (98).
Ceruloplasmin elevation
Exogenous causes of ceruloplasmin elevation are copper intoxication, zinc deficiency and taking hormonal contraceptives. Endogenously, ceruloplasmin reacts as an acute-phase protein in the case of inflammation (88) and to hormonal changes (pregnancy). Oestrogens can stimulate its formation (97). Elevations in the serum ceruloplasmin concentration are also reported in the case of lymphoma, Alzheimer’s disease and cholestasis. Clinical disorders caused by ceruloplasmin elevation itself are not described.
Differential diagnoses of typical clinical findings
Differential diagnoses of icterus and anaemia
If hepatic findings are made, in particular pertaining to icterus as well unclear anaemia, diseases with primary or possible manifestation in toddlers up to puberty are, in addition to Wilson’s disease, significant in regard to differential diagnoses (Table 5).
Amongst others, hemoglobinopathies, iron deficiency, lymphatic leukaemia, erythroblastopenia, glucose-6-phosphate dehydrogenase deficiency and autoimmune haemolytic disorders must be taken into consideration in infantile types of anaemia. The occurrence of different forms of anaemia varies greatly. In Europe, iron deficiency anaemia and thalassemia minor represent the majority of all causes for anaemia in children and adolescents (102).
Differential diagnoses of neurological and psychiatric symptoms with manifestation starting in puberty
Unclear symptoms associated with basal ganglia and the cerebellum must be analysed in differential diagnoses for the presence of Wilson’s disease, particularly before age 45, in exceptional cases even beyond that. Knowledge of the clinical forms of progression (clinical variants) of Wilson’s disease helps recognize deviations in clinical findings (10). Table 6 summarizes relevant neurological movement disorders at comparable ages of onset such as Wilson’s disease.
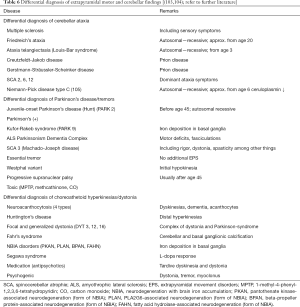
Full table
Particularly unclear cognitive disorders (e.g., worsening of school-based performance) should include the possibility of a primarily psychiatric manifestation of Wilson’s disease at puberty age (3,4). The psychopathological spectrum can include all ranges, so that a localization based on differential diagnoses is not possible a priori. An inclusion of extrapyramidal findings in the neurological status is a decisive factor.
Differential diagnoses of the KFR
The KFR is the macroscopically detectable phenomenon of copper deposition in the Descemet’s membrane of the cornea. They were described as earlier as 1902 by Kayser (106) and therefore, 10 years prior to the publication of progressive lenticular degeneration by Wilson (107). They appear on the edge of the cornea as a brown ring, which is not always closed. They are always mentioned when findings of Wilson’s disease are listed in textbooks and are considered a cardinal symptom (108). Nevertheless, there are numerous different aspects in literature. On the one hand, KFR belong to the initial symptoms (109), on the other hand, they are often not present in the early stages of the disease in children (105). In large populations of patients with Wilson’s disease with hepatic symptoms, the occurrence of KFR was only reported in 44–62% of patients (110,111) and they were usually not present in children with liver disease (112). Furthermore, they are more common in the case of the homozygous H1069Q mutation than in compound heterozygous patients at the time of diagnosis (113).
Initially, KFR was considered pathognomonic for Wilson’s disease, however, they were rarely found in other liver diseases such as primary biliary cholangitis, chronic cholestasis (114,115) or in children with neonatal cholestasis (116). In regard to differential diagnoses, corneal opacities caused by other diseases, such as galactosialidosis (117), are possible. Other authors also refer to this occurrence in “non-Wilson patients” as pseudo-KFR (115,118). Despite these aspects, KFR are considered a classical ophthalmological manifestation of Wilson’s disease, while the sunflower cataract caused by copper accumulation in the lens of the eye is rare (119,120). KFR can fade during decopperising therapy and disappear after years (121).
Differential diagnosis in heavy metal metabolism (manganese storage disease)
The recently recognized manganese storage disease is caused by an autosomal recessive mutation in the manganese transporter gene (SLC30A10) (122). It is considered the “new Wilson’s disease” with the manifestation of a generalized dystonia in childhood (age 2–14) and asymmetrical Parkinson’s disease with early postural instability before age 60 (123,124). The patients also develop liver cirrhosis and in doing so, “mirror” Wilson’s disease (92).
Paraclinical findings include polycythaemia, low ferritin, increased total iron-binding capacity and an elevated serum manganese level (122). The basal ganglia show hyperintense lesions on T1-weighted images (122). Therapeutically, calcium-disodium EDTA infusions are used to boost renal excretion of manganese (92,125). This leads to a good neurological improvement if treatment is started in a timely manner (122,125).
Conclusions
If a clinical tentative diagnosis for Wilson’s disease is made by allocating the current findings based on a classification scheme, considerations based on differential diagnoses must be taken into account until the genetic information has been ensured. Atypical symptoms as well as deviating progressions also require additional diagnosis. It must be taken into account, that a full presentation is not evident at the time of onset of the disease. As a result, differential diagnoses must be included in the respective clinical findings. An extensive understanding of copper metabolism and known disorders, in addition to Wilson’s disease, is significant, in particular for the interpretation of the paraclinical data.
As this overview shows, Wilson’s disease fits into a broad spectrum of internal and neurological disease patterns with icterus, anaemia and EPS. Recently discovered disease patterns pertaining to manganese and copper metabolism are relevant after ruling out an ATP7B mutation. On the one hand, starting treatment for Wilson’s disease early on is required but on the other hand, only a reliable diagnosis justifies treatment, which involves adverse reactions. Starting treatment at an early stage, avoiding wrong medication and the prognostic assessment are also significant for differential diagnoses.
Acknowledgements
None.
Footnote
Conflicts of Interest: The author has no conflicts of interest to declare.
References
- Cuthbert JA. Wilson’s Disease: A new gene and an animal model for an old disease. J Investig Med 1995;43:323-36. [PubMed]
- Maier-Dobersberger T. Morbus Wilson. Diagnosestellung mit konventionellen und molekularbiologischen Methoden. Deutsch med Wschr 1999;124:493-6.
- Brewer GJ. Wilson's disease: A clinician’s guide to recognition, diagnosis, and mangement. Boston Dordrecht London: Kluwer Academic Publishers 2001.
- Hermann W, Huster D. Diagnostik des Morbus Wilson. Nervenarzt 2018;89:115-23. [Crossref] [PubMed]
- Hermann W, Huster D, Ransmayr G, et al. Morbus Wilson. In: Diener H, Weimar C. editors. Leitlinien für Diagnostik und Therapie in der Neurologie. Stuttgart: Thieme 5. Auflage 2012:200-9.
- Roberts EA, Cox DW. Wilson disease. Baillieres Clin Gastroenterol 1998;12:237-56. [Crossref] [PubMed]
- Abuduxikuer K, Li LT, Qiu YL. Wilson disease with hepatic presentation in an eight-month-old boy. World J Gastroenterol 2015;21:8981-4. [Crossref] [PubMed]
- Ala A, Borjigin J, Rochwarger A, et al. Wilson disease in septuagenarian siblings: Raising the bar for diagnosis. Hepatology 2005;41:668-70. [Crossref] [PubMed]
- Ferenci P, Czlonkowska A, Merle U, et al. Late-Onset Wilson’s Disease. Gastroenterology 2007;132:1294-8. [Crossref] [PubMed]
- Hermann W, Tinta C. Klassifikation des Morbus Wilson. Nervenheilkunde 2018;6:426-32.
- Hermann W, Hennig C, Hoffmann J. Fehldiagnose eines Morbus Wilson trotz positiver Genetik. Nervenarzt 2018;89:1408-10. [Crossref] [PubMed]
- Ferenci P. Pathophysiology and clinical features of Wilson disease. Metab Brain Dis 2004;19:229-39. [Crossref] [PubMed]
- Das SK, Ray K. Wilson’s disease: an update. Nat Clin Pract Neurol 2006;2:482-93. [Crossref] [PubMed]
- Attri S, Sharma N, Jahagirdar S, et al. Erythrocyte metabolism and antioxidant status of patients with Wilson disease with hemolytic anemia. Pediatr Res 2006;59:593-7. [Crossref] [PubMed]
- Lössner J, Bachmann H, Biesold D, et al. Untersuchungen zur Wilsonschen Erkrankung in der DDR. Teil II: Pathogenese und Klinik. Z gesamte Inn Med 1980;35:136-41. [PubMed]
- Saito T. Presenting symptoms and natural history of Wilson disease. Eur J Pediatr 1987;146:261-5. [Crossref] [PubMed]
- Scheinberg IH, Sternlieb I. Wilson’s disease. In: Smith LH Jr. editor. Major Problems in Internal Medicine. Philadelphia: WB Saunders 1984:1-171.
- Stremmel W, Niederau C, Strohmeyer G. Genetisch determinierte Lebererkrankungen, Teil II: Morbus Wilson. DIA-GM 1990;10:953.
- Konovalov NV. Gepato-tserebralnaya distrofiya. Moskva: Medgiz, 1960.
- Bachmann H, Lößner J, Biesold D, et al. Aktuelle Erfahrungen und Probleme mit der Langzeitbetreuung von Patienten mit Wilsonscher Erkrankung in der DDR. Z ärztl Fortbild 1988;82:297-304.
- Dening TR, Berrios GE. Wilson’s disease. Psychiatric symptoms in 195 cases. Arch Gen Psychiatry 1989;46:1126-34. [Crossref] [PubMed]
- Rathbun JK. Neuropsychological aspects of Wilson’s disease. Int J Neurosci 1996;85:221-9. [Crossref] [PubMed]
- Cuthbert JA. Wilson’s disease. Update of a systematic disorder with protean manifestations. Gastroenterol Clin North Am 1998;27:655-81. vi-vii. [Crossref] [PubMed]
- Bräunig P, Faig J. Morbus Wilson mit ausschließlich psychopathologischer Initialsymptomatik. In: Huffmann G, Braune HJ, Henn KH. Hrsg. Extrapyramidalmotorische Erkrankungen. Reinbek: Einhorn-Presse Verlag, 1994:545-50.
- Grünberger J, Sluga W, Tschabitscher H. Kriminelle Verhaltensstörung bei Morbus Wilson. Wien Wiener Z Nervenheilk 1967;24:313-25.
- Marsden CD. Wilson’s disease. Q J Med 1987;65:959-66. [PubMed]
- Oder W, Payer L, Grimm G, et al. Wilson’s disease: evidence of subgroups derived from clinical findings and brain lesions. Neurology 1993;43:120-4. [Crossref] [PubMed]
- Mercer JF. Gene regulation by copper and the basis for copper homeostasis. Nutrition 1997;13:48-9. [Crossref] [PubMed]
- Lutsenko S, Gupta A, Burkhead JL, et al. Cellular multitasking: the dual role of human Cu-ATPases in cofactor delivery and intracellular copper balance. Arch Biochem Biophys 2008;476:22-32. [Crossref] [PubMed]
- Valentine JS, Gralla EB. Biochemistry – delivering copper inside yeast and human cells. Science 1997;278:817-8. [Crossref] [PubMed]
- Ferenci P, Zollner G, Trauner M. Hepatic transport systems. J Gastroenterol Hepatol 2002;17:S105-12. [Crossref] [PubMed]
- Gollan JL. Copper metabolism, Wilson’s disease, and hepatic copper toxicosis. In: Zankim D, Boyer TD. Hrsg. Hepatology – A textbook of liver disease. 2nd ed. Philadelphia: Saunders, 1990:1249-72.
- Cox DW. Molecular advances in Wilson disease. Prog Liver Dis 1996;14:245-64. [PubMed]
- Harris ED. Copper transport: an overview Proc Soc Exp Biol Med 1991;196:130-40. (review). [Crossref] [PubMed]
- Thomas L. Labor und Diagnose. Frankfurt/Main: TH-Books Verlagsgesellschaft mbH, 1998.
- Gressner AM, Arndt T. Lexikon der Medizinischen Laboratoriumsdiagnostik. 2nd ed. Berlin Heidelberg: Springer, 2013.
- Yoshida K, Furihata K, Takeda S, et al. A mutation in the coeruloplasmin gene is associated with systemic hemosiderosis in humans. Nat Genet 1995;9:267-72. [Crossref] [PubMed]
- Ferenci P, Steindl-Munda P, Vogel W, et al. Diagnostic value of quantitative hepatic copper determination in patients with Wilson’s disease. Clin Gastroenterol Hepatol 2005;3:811-8. [Crossref] [PubMed]
- Hamza I, Schaefer M, Klomp LW, et al. Interaction of copper chaperone HAH1 with the Wilson disease protein is essential for copper homeostasis. Proc Natl Acad Sci U S A 1999;96:13363-8. [Crossref] [PubMed]
- Harrison MD, Jones CE, Solio M, et al. Intracellular copper routing. The role of copper chaperons. Trends Biochem Sci 2000;25:29-32. [Crossref] [PubMed]
- Roelofsen H, Wolters H, van Luyn MJA, et al. Copper-induced apical trafficking of ATP 7B in polarized hepatoma cells provides a mechanism of biliary copper excretion. Gastroenterology 2000;119:782-93. [Crossref] [PubMed]
- Burstein E, Ganesh L, Dick RD, et al. A novel role for XIAP in copper homeostasis through regulation of MURR1. EMBO J 2004;23:244-54. [Crossref] [PubMed]
- Klomp AEM, van de Sluis B, Klomp LW, et al. The ubiquitously expressed MURR1 is absent in canine copper toxicosis. J Hepatol 2003;39:703-9. [Crossref] [PubMed]
- Tao TY, Liu F, Klomb L, et al. The copper toxicosis gene product MURR1 directly interacts with the Wilson disease protein. J Biol Chem 2003;278:41593-6. [Crossref] [PubMed]
- Vonk WIM, Kakkar V, Bartuzi P, et al. The Copper Metabolism MURR1 Domain Protein 1 (COMMD1) Modulates the Aggregation of Misfolded Protein Species in a Client-Specific Manner. PLoS One 2014;9:e92408. [Crossref] [PubMed]
- Vaupel P, Scheible HG, Mutschler E. Anatomie, Physiologie, Pathophysiologie des Menschen. 7th ed. Stuttgart: Wissenschaftliche Verlagsgesellschaft, 2015.
- Jennifer McDowall/Interpro: ATP Synthase: The ATPase-Family. Available online: http://www.ebi.ac.uk/interpro/potm/2005_12/Page2.htm
- Tsivkovskii R, Eisses JF, Kaplan JH, et al. Functional Properties of the Copper-transporting ATPase ATP7B (The Wilson's Disease Protein) Expressed in Insect Cells. J Biol Chem 2002;277:976-83. [Crossref] [PubMed]
- DiDonato M, Bibudhendra S. Copper transport and its alterations in Menkes and Wilson diseases. Biochim Biophys Acta 1997;1360:3-16. [Crossref] [PubMed]
- Bull PC, Thomas GR, Rommens JM, et al. The Wilson disease gene is a putative copper transporting P-Type ATPase similar to the Menkes gene. Nat Genet 1993;5:327-37. [Crossref] [PubMed]
- Tanzi RE, Petrukhin K, Chernov I, et al. The Wilson disease gene is a copper transporting ATPase with homology to the Menkes disease gene. Nat Genet 1993;5:344-50. [Crossref] [PubMed]
- Bowcock AM, Farrer LA, Hebert JM, et al. Eight closely linked loci place the Wilson disease locus within 13q14-q21. Am J Hum Genet 1988;43:664-74. [PubMed]
- Carafoli E. P-Typ ATPases. Introduction. J Bioenerg Biomembr 1992;24:245-7. [PubMed]
- Vulpe CD, Packman S. Cellular copper transport. Annu Rev Nutr 1995;15:293-322. [Crossref] [PubMed]
- Kaler SG. ATP 7A-Related copper transport diseases –Emerging concepts and future trends. Nat Rev Neurol 2011;7:15-29. [Crossref] [PubMed]
- Bahi-Buisson N, Kaminska A, Nabbout R, et al. Epilepsy in Menkes disease: analysis of clinical stages. Epilepsia 2006;47:380-6. [Crossref] [PubMed]
- Kaler SG. Metabolic and molecular bases of Menkes disease and occipital horn syndrome. Pediatr Dev Pathol 1998;1:85-98. [Crossref] [PubMed]
- Kaler SG, Holmes CS, Goldstein DS, et al. Neonatal diagnosis and treatment of Menkes disease. N Engl J Med 2008;358:605-14. [Crossref] [PubMed]
- Kaler SG, Goldstein DS, Holmes C, et al. Plasma and cerbrospinal fluid neurochemical pattern in Menkes Disease. Ann Neurol 1993;33:171-5. [Crossref] [PubMed]
- Tang J, Robertson SP, Lem KE, et al. Functional copper transport explains neurologic sparing in occipital horn syndrome. Genet Med 2006;8:711-8. [Crossref] [PubMed]
- Kennerson ML, Nicholson GA, Kaler SG, et al. Missense mutations in the copper transporter gene ATP7A cause X-linked distal hereditary motor neuropathy. Am J Hum Genet 2010;86:343-52. [Crossref] [PubMed]
- Yi L, Donsante A, Kennerson ML, et al. Altered intra-cellular localization and valosin-containing protein (p97 VCP) interaction underlie ATP7A-related distal motor neuropathy. Hum Mol Genet 2012;21:1794-807. [Crossref] [PubMed]
- Barthel H, Hermann W, Kluge R, et al. Concordant pre- and postsynaptic deficits of dopaminergic neurotransmission in neurologic Wilson disease. Am J Neuroradiol 2003;24:234-8. [PubMed]
- Barthel H, Sorger D, Kühn HJ, et al. Differential alteration of nigrostriatal dopaminergic system in Wilson’s disease investigated with (123 I)ß-CIT and high resolution SPET. Eur J Nucl Med 2001;28:1656-63. [Crossref] [PubMed]
- Nayak NC, Chitale AR. Indian childhood cirrhosis (ICC) & ICC-like diseases: The changing scenario of facts versus notions. The Indian Journal of Medical Research 2013;137:1029-42. [PubMed]
- Tanner MS. Role of copper in Indian childhood cirrhosis. Am J Clin Nutr 1998;67:1074S-81S. [Crossref] [PubMed]
- Freed EF, Prieto JL, McCann KL, et al. NOL11, Implicated in the Pathogenesis of North American Indian Childhood Cirrhosis, Is Required for Pre-rRNA Transcription and Processing. PLoS Genet 2012;8:e1002892. [Crossref] [PubMed]
- Martinelli D, Travaglini L, Drouin CA, et al. MEDNIK syndrome: a novel defect of copper metabolism treatable by zinc acetate therapy. Brain 2013;136:872-81. [Crossref] [PubMed]
- Montpetit A, Cote S, Brustein E, et al. Disruption of AP1S1, causing a novel neurocutaneous syndrome, perturbs development of the skin and spinal cord. PLoS Genet 2008;4:e1000296. [Crossref] [PubMed]
- Leroy P, Meyer F, Vaessen S, et al. Dystonia 12: A rare and difficult diagnosis. Arch Pediatr 2017;24:637-9. [Crossref] [PubMed]
- Rosewich H, Baethmann M, Ohlenbusch A, et al. A novel ATP1A3 mutation with unique clinical presentation. J Neurol Sci 2014;341:133-5. [Crossref] [PubMed]
- Hampshire DJ, Roberts E, Crow Y, et al. Kufor-Rakeb syndrome, pallido-pyramidal degeneration with supranuclear upgaze paresis and dementia, maps to 1p36. J Med Genet 2001;38:680-2. [Crossref] [PubMed]
- Prashanth LK, Murugan S, Kamath V, et al. First Report of Kufor-Rakeb Syndrome (PARK 9) from India, and a Novel Nonsense Mutation in ATP13A2 Gene. Mov Disord Clin Pract 2015;2:326-7. [Crossref] [PubMed]
- Huppke P, Brendel C, Korenke GC, et al. Molecular and biochemical characterization of a unique mutation in CCS, the human copper chaperone to superoxide dismutase. Hum Mutat 2012;33:1207-15. [Crossref] [PubMed]
- Aspin N, Sass-Kortsak A. Copper. In: Bronner F, Coburn JW. Hrsg. Disorders of mineral metabolism, Vol I: Trace minerals. New York: Academic Press, 1981.
- Linder MC, Hazegh-Azam M. Copper biochemistry and molecular biology. Am J Clin Nutr 1996;63:797S-811S. [PubMed]
- Kreutzig T. Kurzlehrbuch Biochemie. Urban & Fischer-Verlag/Elsevier GmbH 12. Auflage, 2006.
- Kumar N. Neurologic complications of bariatric surgery. Continuum (Minneap Minn) 2014;20:580-97. [Crossref] [PubMed]
- Nations SP, Boyer PJ, Love LA, et al. Denture cream: An unusual source of excess zinc, leading to hypocupremia and neurologic disease. Neurology 2008;71:639-43. [Crossref] [PubMed]
- Rowin J, Lewisaff SL. Copper deficiency myeloneuropathy and pancytopenia secondary to overuse of zinc supplementation. J Neurol Neurosurg Psychiatry 2005;76:750-1. [Crossref] [PubMed]
- Lampon N, Tutor JC. A preliminary investigation on the possible association between diminished copper availability and non-alcoholic fatty liver disease in epileptic patients treated with valproic acid. Ups J Med Sci 2011;116:148-54. [Crossref] [PubMed]
- Prohaska JR. Impact of copper defiency in humans. Ann N Y Acad Sci 2014;1314:1-5. [Crossref] [PubMed]
- Wazir SM, Ghobrial I. Copper deficiency, a new triad: anemia, leucopenia, and myeloneuropathy. J Community Hosp Intern Med Perspect 2017;7:265-8. [Crossref] [PubMed]
- Chen M, Krishnamurthy A, Mohamed AR, et al. Hematological Disorders following Gastric Bypass Surgery: Emerging Concepts of the Interplay between Nutritional Deficiency and Inflammation. Biomed Res Int 2013;2013:205467. [PubMed]
- Pizarro F, Olivares M, Uauy R, et al. Acute gastrointestinal effects of graded levels of copper in drinking water. Environmental Health Perspectives 1999;107:117-21. [Crossref] [PubMed]
- Franchitto N, Gandia-Mailly P, Georges B, et al. Acute copper sulphate poisoning: a case report and literature review. Resuscitation 2008;78:92-6. [Crossref] [PubMed]
- Ludewig R, Lohs KH. Akute Vergiftungen. Ratgeber für toxikologische Notfälle. Jena: VEB Gustav Fischer Verlag, 1966.
- Egger G. Die Akute Entzündung: Grundlagen, Pathophysiologie und klinische Erscheinungsbilder der unspezifischen Immunität. Wien New York: Springer, 2005.
- Hellman NE, Gitlin JD. Ceruloplasmin metabolism and function. Annu Rev Nutr 2002;22:439-58. [Crossref] [PubMed]
- Saenko EL, Yaropolov AI, Harris ED. The biological functions of ceruloplasmin expressed through copperbinding sites and a cellular receptor. J Trace Elem Exp Med 1994;7:69-88.
- Kono S. Aceruloplasminemia: an update. Int Rev Neurobiol 2013;110:125-51. [Crossref] [PubMed]
- Bandmann O, Weiss KH, Kaler SG. Wilson's disease and other neurological copper disorders. Lancet Neurol 2015;14:103-13. [Crossref] [PubMed]
- Ogimoto M, Anzai K, Takenoshita H, et al. Criteria for early identification of aceruloplasminemia. Intern Med 2011;50:1415-8. [Crossref] [PubMed]
- McNeill A, Pandolfo M, Kuhn J, et al. The neurological presentation of ceruloplasmin gene mutations. Eur Neurol 2008;60:200-5. [Crossref] [PubMed]
- Chiplunkar S, Bindu PS, Nagappa M, et al. Huppke-Brendel syndrome in a seven month old boy with a novel 2-bp deletion in SLC33A1. Metabolic Brain Disease 2016;31:1195-8. [Crossref] [PubMed]
- Huppke P, Brendel C, Kalscheuer V, et al. Mutations in SLC33A1 cause a lethal autosomal-recessive disorder with congenital cataracts, hearing loss, and low serum copper and ceruloplasmin. Am J Hum Genet 2012;90:61-8. [Crossref] [PubMed]
- Caspary U, Leuschner S, Zeuzem S. Therapie von Leber- und Gallekrankheiten. 2 Auflage. Berlin Heidelberg: Springer, 2001.
- Fryer AA, Jones P, Strange R, et al. Plasma protein levels in normal human fetuses: 13 to 41 weeks’ gestation. Br J Obstet Gynaecol 1993;100:850-5. [Crossref] [PubMed]
- Manns MP, Schneidewind S. Praxis der Hepatologie. 1 Auflage. Berlin Heidelberg: Springer, 2016.
- Speer CP, Gahr M. Pädiatrie. 4 Auflage. Berlin Heidelberg: Springer, 2013.
- Wiener CM, Fauci AS, Braunwald E, et al. Harrison’s Principles of Internal Medicine. 19th ed. New York: McGraw-Hill Education – Europe, 2017.
- Kunz JB, Kulozik AE. Differentialdiagnose der kindlichen Anämie. Monatsschrift Kinderheilkunde 2012;160:395-406. [Crossref]
- Daroff R, Jankovic J, Mazziotta J, et al. Bradley's Neurology in Clinical Practice. 7th Edition. Amsterdam: Elsevier, 2015.
- Hufschmidt A, Lücking CH, Rauer S, et al. editors. Neurologie compact. 7 Auflage. Stuttgart: Thieme, 2017.
- Roberts EA, Socha P. Wilson disease in children. In: Wilson M, Czlonkowska A, Schilsky ML. editors. Handbook of clinical neurology, 142, 3rd Series. Amsterdam: Elsevier, 2017:141-56.
- Kayser B. Über einen Fall von angeborener Grünlichverfärbung der Cornea. Klin Mbl Augenheilk 1902;40:22-5.
- Wilson SAK. Progressive lenticular degeneration: a familial nervous disease associated with cirrhosis of the liver. Brain 1912;34:295-507. [Crossref]
- Walshe JM. History of Wilson disease: a personal account. In: Wilson M, Czlonkowska A, Schilsky ML. editors. Handbook of clinical neurology, 142, 3rd Series. Amsterdam: Elsevier, 2017:1-7.
- Cocoş R, Sendroiu A, Schipor S, et al. Genotype-phenotype correlations in a mountain population community with high prevalence of Wilson's disease: genetic an clinical homogeneity. PLoS One 2014;9:e98520. [Crossref] [PubMed]
- Merle U, Schaefer M, Ferenci P, et al. Clinical presentation, diagnosis and long-term outcome of Wilson's disease: a cohort study. Gut 2007;56:115-20. [Crossref] [PubMed]
- Steindl P, Ferenci P, Dieners HP, et al. Wilson’s disease in patients presenting with liver disease: a diagnostic challenge. Gastroenterology 1997;113:212-8. [Crossref] [PubMed]
- Sánchez-Albisua I, Garde T, Hierro L, et al. A high index of suspicion: the key to an early diagnosis of Wilson's disease in childhood. J Pediatr Gastroenterol Nutr 1999;28:186-90. [Crossref] [PubMed]
- Folhoffer A, Ferenci P, Csak T, et al. Novel mutations of the ATP7B gene among 109 Hungarian patients with Wilson's disease. Eur J Gastroenterol Hepatol 2007;19:105-11. [Crossref] [PubMed]
- Fleming CR, Dickson ER, Hellenhorst ER, et al. Pigmented corneal rings in a patient with biliary cirrhosis. Gastroenterology 1975;69:220-5. [PubMed]
- Tauber J, Steinert RF. Pseudo-Kayser-Fleischer ring of the cornea associated with non-Wilsonian liver disease. A case report and literature review. Cornea 1993;12:74-7. [Crossref] [PubMed]
- Dunn LL, Annable WL, Kliegman RM. Pigmented corneal rings in neonates with liver disease. J Pediatr 1987;110:771-6. [Crossref] [PubMed]
- Mongalgi MA, Toumi NH, Cheour M, et al. Galactosialidosis with Kayser-Fleischer’s ring. Arch Fr Pediatr 1992;49:193-5. [PubMed]
- Finelli PF. Kayser-Fleischer ring: hepatolenticular degeneration (Wilson's disease). Neurology 1995;45:1261-2. [Crossref] [PubMed]
- Cairns JE, Parry WH, Walshe JM. “Sunflower cataract” in Wilson’s disease. Br Med J 1969;3:95-6. [Crossref] [PubMed]
- Caim JE, Parry WH, Walshe JM. The Kayser Fleisher ring. Trans Ophthalol Soc 1970;90:187-90.
- Lössner A, Lössner J, Bachmann H, et al. The Kayser-Fleischer ring during long-term treatment in Wilson's disease (hepatolenticular degeneration). A follow-up study. Graefes Arch Clin Exp Ophthalmol 1986;224:152-5. [Crossref] [PubMed]
- Stamelou M, Tuschl K, Chong WK, et al. Dystonia with Brain manganese accumulation resulting from SLC30A10 mutations: a new treatable disorder. Mov Disord 2012;27:1317-22. [Crossref] [PubMed]
- Quadri M, Federico A, Zhao T, et al. Mutations in SLC30A10 cause parkinsonism and dystonia with hypermanganesemia, polycythemia, and chronic liver disease. Am J Hum Genet 2012;90:467-77. [Crossref] [PubMed]
- Tuschl K, Clayton PT, Gospe SM Jr, et al. Syndrome of hepatic cirrhosis, dystonia, polycythemia, and hypermanganesemia caused by mutations in SLC30A10, a manganese transporter in man. Am J Hum Genet 2012;90:457-66. [Crossref] [PubMed]
- Di Toro Mammarella L, Mignarri A, Battisti C, et al. Two-year follow-up after chelating therapy in a patient with adult-onset parkinsonism and hypermanganesaemia due to SLC30A10 mutations. J Neurol 2014;261:227-8. [Crossref] [PubMed]