The effect of walnut rolling training on hand function and corticospinal tract
Introduction
In-hand manipulation (IHM) ability is defined as the ability to move an object within a hand. It is necessary for activities of daily living, including tool use and handling small objects (1). Exner [1990] classified IHM into three skills: (I) translation: the ability to move an object from the finger to the palm and from the palm to the finger; (II) shift: the ability to move an object in a linear manner with the finger; and (III) rotation: the ability to turn an object around in the pads of the fingers and thumb (simple rotation) or turning an object from end to end (complex rotation) (1). Hand rolling using a walnut or an iron ball is a kind of IHM training that has been popular for a long time among old people in northeastern Asia. Walnut rolling training is popular for old people to prevent dementia in Korea. A few studies have demonstrated the cortical activation by ball rotation task in hand, using functional MRI (2-4). Among the above studies, one study reported that the walnut rolling task was the most effective for cortical activation compared with wooden ball rolling and hand grasp-release movements (3). However, it is unclear whether the walnut rolling training has an effect on the function of the hand and related neural tract in the brain.
The corticospinal tract (CST) is a major neural tract for motor function in the human brain, especially for fine motor activity of the hand (5-7). Therefore, the CST is assumed to be easily influenced by walnut rolling training, and examination of the CST would be important for evaluation of the effect of walnut rolling training on the brain. The most widely used methods for evaluating the state of the CST include transcranial magnetic stimulation (TMS), functional magnetic resonance imaging (fMRI), and diffusion tensor imaging (DTI) with diffusion tensor tractography (DTT). Compared to TMS and fMRI, DTT, which is derived from DTI, has a unique advantage of visualization and quantitative evaluation of the CST (8,9). Therefore, DTT is commonly used to detect changes of the CST (10,11).
In this study, we hypothesized that walnut rolling training could improve hand function and facilitate the contralateral fiber number (FN) of CST. To test our hypothesis, we investigated the effect of the walnut rolling training for two weeks on the hand function and CST in normal subjects.
Methods
Subjects
Seventeen right-handed healthy normal subjects (8 males, 9 females; mean age: 36.53±9.24 years, range: 25–55) were recruited according to the following criteria: (I) no brain lesion on conventional MRI [T1-weighted, T2-weighted, fluid attenuated inversion recovery (FLAIR), and T2-weighted gradient recall echo (GRE) images]; (II) no history of psychiatric, neurological, or physical illness; (III) right handedness as confirmed by the Edinburgh Handedness Inventory (12). The subjects for this study provided signed, informed consent, and the study protocol was approved by our Institutional Review Board (Ethical Application Ref: YUMC-2017-06-020).
The walnut rolling training
Subjects performed the walnut rolling training with their non-dominant (left) hand. Subjects were allowed to pick two walnuts which suit best to their hand among walnuts with various sizes: the selected walnuts were 2.5 cm in diameter at most. They were asked to roll the walnut in the counterclockwise direction within their non-dominant hand. The walnut rolling training was performed with a steady speed (approximately 0.5 Hz) three times per day, for 30 minutes at a time, over two weeks. To ensure that each participant complied with the rules dedicating 90 minutes per day to the training, a supervisor checked whether the walnut rolling training was performed during the specified time using video telephony (first training: am 8:00 to 8:30, second training: pm 1:00 to 1:30, and third training: pm 5:00 to 5:30).
Clinical evaluation
The Purdue Pegboard Test (PPT), tip pinch and grip strength (GS) were used to evaluate hand function at pre- and post-walnut rolling training. To evaluate the PPT (Lafayette instruments, model-32020), the subjects were asked to place pegs in holes as quickly as possible; the score of the PPT is the number of pegs placed in holes within 30 seconds (13-17). For the tip pinch (Jamar Hydraulic Pinch Gauge, model-J00111), the subjects were asked push with the tip of their index finger and hold the pinch gauge with thumb as forcefully as possible, the parameters of the pinch test indicates the strength of the pinch of thumb and index finger. Finally, for GS, the subjects were asked to sit on a chair in an upright position (hip joint flexed at 90° and shoulder joint in a neutral position, elbow fixed at 90° flexion, forearm in a neutral position, and wrist at 0° to 15° radial deviation), then to grasp the Jamar dynamometer (Jamar Hydraulic Hand Dynamometer, model-5030J1) with maximal strength (the score represents the GS of the hand). The reliability and validity of PPT (16), tip pinch (18) and GS (19) are well established. All of the clinical evaluations were performed three times and average values for each test were used in the statistical analysis.
DTT
DTI data were acquired twice (pre- and post- walnut rolling training) using a 1.5 T Philips Gyroscan Intera system (Philips, Ltd, Best, The Netherlands) equipped with a Synergy-L Sensitivity Encoding (SENSE) head coil using a single-shot, spin-echo planar imaging pulse sequence. For each of the 32 non-collinear diffusion sensitizing gradients, 60 contiguous slices were acquired parallel to the anterior commissure-posterior commissure line. Imaging parameters were as follows: acquisition matrix =96×96, reconstructed to matrix =192×192, field of view =240 mm × 240 mm, TR =10,398 ms, TE =72 ms, parallel imaging reduction factor (SENSE factor) =2, EPI factor =59 and b =1,000 s/mm2, NEX =1, thickness =2.5 mm.
Reconstruction of the CST
Fiber tracking was performed using the fiber assignment continuous tracking algorithm implemented within the DTI task card software (Philips Extended MR WorkSpace 2.6.3). Each DTI replication was intra-registered to the baseline “b0” images to correct for residual eddy-current image distortions and head motion effect, using a diffusion registration package (Philips Medical Systems). The CST was reconstructed using fibers passing through two regions of interest (ROIs) on the color map. The seed ROI was placed at the upper pons (portion of anterior blue color) on the color map with an axial image. The target ROI was placed at the mid pons (portion of anterior blue color) on the color map with an axial image. Termination criteria used for fiber tracking were fractional anisotropy (FA) <0.15, angle <27° (20).
Reconstruction of the transcallosal fiber for the hand motor somatotopy
Diffusion-weighted imaging data were analyzed using the Oxford Centre for Functional Magnetic Resonance Imaging of the Brain (FMRIB) Software Library (FSL; www.fmrib.ox.ac.uk/fsl). Affine multi-scale two-dimensional registration corrected the head motion effect and image distortion due to eddy current. Fiber tracking was performed using a probabilistic tractography method based on a multifiber model, and applied in the current study utilizing tractography routines implemented in FMRIB Diffusion (5,000 streamline samples, 0.5 mm step lengths, curvature thresholds =0.2). The transcallosal fiber (TCF) for the hand somatotopy of the primary motor cortex was identified by selection of fibers passing through both ROIs. Seed ROIs were placed on the precentral knob. A target ROI was placed on the CC on the color map (21). Out of 5,000 samples generated from the seed voxel, results for contact were visualized at a threshold for the TCF for the hand motor somatotopy of 20 streamlined through each voxel for analysis. Values of FA and FN of the reconstructed CSTs and TCF for the hand motor somatotopy were measured.
Statistical analysis
Statistical analyses were performed using SPSS software (SPSS Inc. Released 2009. PASW Statistics for Windows, Version 18.0. Chicago: SPSS Inc.). The paired t-test was used for determination of differences in values of the DTT parameters (FA and FN) and scores of clinical evaluations (PPT, tip pinch, and GS). Pearson correlation coefficients were calculated to assess the strength of association between scores of clinical evaluations (PPT, tip pinch and GS) and DTT parameters of the CST and TCF. Null hypotheses of no difference were rejected if P values were less than 0.05.
Results
The clinical scores of PPT, tip pinch and GS are summarized in Table 1. The clinical scores of PPT, tip pinch and GS increased significantly following training in the left hand compared with pre-training (P<0.05). By contrast, the clinical scores for the right hand did not differ significantly between pre- and post-training (P>0.05).
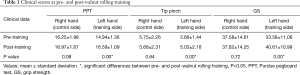
Full table
DTT parameters for the right CST, left CST, and TCF for the hand motor somatotopy are revealed in Table 2. FN of the right CST increased significantly in post-training DTT compared with that of pre-training DTT (P<0.05) (Figure 1A), whereas FA did not change significantly (P>0.05). Both FA and FN of the left CST and TCF for the hand motor somatotopy did not change significantly between pre- and post-training DTTs (P>0.05) (Figure 1B,C).
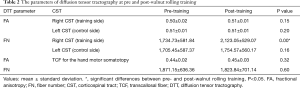
Full table
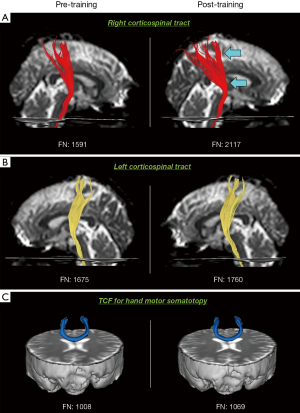
Discussion
In the current study, we investigated the change of the hand function, and the CST and TCF in hand motor somatotopy resulting from two weeks’ walnut rolling training in 17 normal subjects. We found the following results: (I) PPT, tip pinch, and GS increased in the training hand (the left hand) without change in the non-training hand (the right hand); and (II) the contralateral (right) CST that innervates the walnut rolling training side (the left hand) showed increased FN without change of FA value. However, the ipsilateral (left) CST and TCF for the hand motor somatotopy did not change in FA and FN with the walnut rolling training.
In the clinical assessment for change of the hand function, PPT, tip pinch and GS increased in the training side (the left hand) by two week’s walnut rolling training. The increased scores of PPT might be explained by the nature of walnut rolling, which facilitates the fine motor ability of hand. It includes individual movements of the thumb and the remaining four fingers of flexion, extension, abduction, adduction, and opposition. These results suggest that training involving finger movements like walnut rolling are effective for improving fine motor ability of the hand. For tip pinch and GS, which represent forces of the fingers and hand, rotation and holding of the walnut during walnut rolling appeared to contribute to increase these forces. Our results with regard to the change of hand function agree with studies that found a close association between the CST and fine motor ability and gross power of the hand (22-30).
In DTT parameters on pre- and post-training DTTs, only FN of the right CST that innervate the training (left) hand without change of FA value and in other neural tracts (the left CST and TCF for the hand motor somatotopy). FN of the CST indicates the existing FN of the CST (31,32). By contrast, FA value, which indicates the degree of directionality of water diffusion, represents the degree of directionality and integrity of white matter microstructures, such as axon, myelin, and microtubule (9,33). Our observation that FN of the right CST increased without change of FA value suggest that the total FN of the right CST increased with two weeks of walnut rolling training. This agrees with studies that report an association between improvement of motor function and increment of FN of the contralateral CST (34-36). No change of DTT parameters of the left CST and TCF for the hand motor somatotopy means the effect of the walnut rolling training was confined to the contralateral CST (37).
Many neuroimaging studies reported on the effects of IHM. Only a few studies reported an effect of IHM similar to the walnut rolling in this study (2-4). In 1998, Kawashima et al. reported increased cerebral blood flow in the primary motor area, premotor area, and cerebellum during the ball rotation task using position emission tomography in eight normal subjects (2). In 2006, Jang et al. evaluated the differences of the cortical activation by walnut rolling, wooden-ball rolling, and hand grasp-release movement using fMRI in 12 normal subjects (3). They found total activation in the cerebral cortex occurred in order of walnut rolling, wooden ball rolling, and hand grasp-release movement. In 2008, Park et al. investigated the difference of cortical activation during wooden ball rotation task and grasp-release movement of the hand using fMRI in 11 normal subjects (4). They found stronger activation of the primary sensorimotor cortex, premotor area, and the ipsilateral cerebellum during wooden ball rotation task compared with grasp-release movement of the hand. To the best of our knowledge, this is the first study to demonstrate an effect of walnut rolling training on the hand function and the CST on DTT in normal subjects. However, the limitations of this study should be considered. First, this study included a small number of subjects. Second, although DTI is a good anatomic imaging tool, it can produce both false positive and negative results due to the complexity and crossing fiber effect (38). Third, we used the hand opposite from the walnut rolling training hand as a control rather than recruiting a control group.
In conclusion, we demonstrated improvement of the hand function and facilitation of the contralateral CST by two weeks’ walnut rolling training in normal subjects. Our results suggest that walnut rolling training can be used for improvement of hand function and facilitation of the contralateral CST for the patients with brain injury as well as normal subjects. As a result, intensive short-term IHM training (90 minutes per day during two weeks) led to some increase in hand function and facilitation of the contralateral CST. This carries important implications for sport or skill training for normal subjects and neuro-rehabilitation for patients with brain injury.
Acknowledgements
This research was supported by Health Fellowship Foundation.
Footnote
Conflicts of Interest: The authors have no conflicts of interest to declare.
Ethical Statement: The study was approved by our Institutional Review Board (Ethical Application Ref: YUMC-2017-06-020) and written informed consent was obtained from all patients.
References
- Exner CE. The zone of proximal development in in-hand manipulation skills of nondysfunctional 3- and 4-year-old children. Am J Occup Ther 1990;44:884-91. [Crossref] [PubMed]
- Kawashima R, Matsumura M, Sadato N, et al. Regional cerebral blood flow changes in human brain related to ipsilateral and contralateral complex hand movements--a PET study. Eur J Neurosci 1998;10:2254-60. [Crossref] [PubMed]
- Jang SH, Park SK, Kim JW, et al. The cortical effect of walnut rolling motor tast. JKCBOT 2006;14:25-33.
- Park JW, Kwon YH, Lee MY, et al. Brain activation pattern according to exercise complexity: a functional MRI study. NeuroRehabilitation 2008;23:283-8. [PubMed]
- York DH. Review of descending motor pathways involved with transcranial stimulation. Neurosurgery 1987;20:70-3. [Crossref] [PubMed]
- Davidoff RA. The pyramidal tract. Neurology 1990;40:332-9. [Crossref] [PubMed]
- Jang SH. The corticospinal tract from the viewpoint of brain rehabilitation. J Rehabil Med 2014;46:193-9. [Crossref] [PubMed]
- Basser PJ, Pierpaoli C. Microstructural and physiological features of tissues elucidated by quantitative-diffusion-tensor MRI. J Magn Reson B 1996;111:209-19. [Crossref] [PubMed]
- Mori S, Crain BJ, Chacko VP, et al. Three-dimensional tracking of axonal projections in the brain by magnetic resonance imaging. Ann Neurol 1999;45:265-9. [Crossref] [PubMed]
- Jang SH, Yi JH, Choi BY, et al. Changes of the corticospinal tract in the unaffected hemisphere in stroke patients: A diffusion tensor imaging study. Somatosens Mot Res 2016;33:1-7. [Crossref] [PubMed]
- Jang SH, Jang WH. Change of the Corticospinal Tract in the Unaffected Hemisphere by Change of the Dominant Hand Following Stroke: A Cohort Study. Medicine (Baltimore) 2016;95:e2620. [Crossref] [PubMed]
- Oldfield RC. The assessment and analysis of handedness: the Edinburgh inventory. Neuropsychologia 1971;9:97-113. [Crossref] [PubMed]
- Tiffin J, Asher EJ. The Purdue pegboard; norms and studies of reliability and validity. J Appl Psychol 1948;32:234-47. [Crossref] [PubMed]
- Smith RO, Benge MW. Pinch and grasp strength: standardization of terminology and protocol. Am J Occup Ther 1985;39:531-5. [Crossref] [PubMed]
- Reddon JR, Gill DM, Gauk SE, et al. Purdue Pegboard: test-retest estimates. Percept Mot Skills 1988;66:503-6. [Crossref] [PubMed]
- Kim YT, Kang SY, Kim HS, et al. Hand strength and dextricity evaluation with age. J Kor Acad Rehabil Med 1994;18:780-8.
- Kong S, Lee KS, Kim J, et al. The effect of two different hand exercises on grip strength, forearm circumference, and vascular maturation in patients who underwent arteriovenous fistula surgery. Ann Rehabil Med 2014;38:648-57. [Crossref] [PubMed]
- Mathiowetz V, Weber K, Volland G, et al. Reliability and validity of grip and pinch strength evaluations. J Hand Surg Am 1984;9:222-6. [Crossref] [PubMed]
- Hamilton GF, McDonald C, Chenier TC. Measurement of grip strength: validity and reliability of the sphygmomanometer and jamar grip dynamometer. J Orthop Sports Phys Ther 1992;16:215-9. [Crossref] [PubMed]
- Kunimatsu A, Aoki S, Masutani Y, et al. Three-dimensional white matter tractography by diffusion tensor imaging in ischaemic stroke involving the corticospinal tract. Neuroradiology 2003;45:532-5. [Crossref] [PubMed]
- Seo JP, Jang SH. Anatomical location of transcallosal sensorimotor fibers in the human brain: Diffusion tensor tractography study. Transl Neurosci 2013;4:363-7. [Crossref]
- Lemon RN. The G. L. Brown Prize Lecture. Cortical control of the primate hand. Exp Physiol 1993;78:263-301. [Crossref] [PubMed]
- Thickbroom GW, Byrnes ML, Archer SA, et al. Motor outcome after subcortical stroke: MEPs correlate with hand strength but not dexterity. Clin Neurophysiol 2002;113:2025-9. [Crossref] [PubMed]
- Lang CE, Schieber MH. Differential impairment of individuated finger movements in humans after damage to the motor cortex or the corticospinal tract. J Neurophysiol 2003;90:1160-70. [Crossref] [PubMed]
- Sasaki S, Isa T, Pettersson LG, et al. Dexterous finger movements in primate without monosynaptic corticomotoneuronal excitation. J Neurophysiol 2004;92:3142-7. [Crossref] [PubMed]
- Dafotakis M, Grefkes C, Eickhoff SB, et al. Effects of rTMS on grip force control following subcortical stroke. Exp Neurol 2008;211:407-12. [Crossref] [PubMed]
- Lemon RN. Descending pathways in motor control. Annu Rev Neurosci 2008;31:195-218. [Crossref] [PubMed]
- Baker SN. The primate reticulospinal tract, hand function and functional recovery. J Physiol 2011;589:5603-12. [Crossref] [PubMed]
- Wei W, Bai L, Wang J, et al. A longitudinal study of hand motor recovery after sub-acute stroke: a study combined FMRI with diffusion tensor imaging. PLoS One 2013;8:e64154. [Crossref] [PubMed]
- Parikh P, Davare M, McGurrin P, et al. Corticospinal excitability underlying digit force planning for grasping in humans. J Neurophysiol 2014;111:2560-9. [Crossref] [PubMed]
- Pagani E, Agosta F, Rocca MA, et al. Voxel-based analysis derived from fractional anisotropy images of white matter volume changes with aging. Neuroimage 2008;41:657-67. [Crossref] [PubMed]
- Jang SH, Chang CH, Lee J, et al. Functional role of the corticoreticular pathway in chronic stroke patients. Stroke 2013;44:1099-104. [Crossref] [PubMed]
- Assaf Y, Pasternak O.. Diffusion tensor imaging (DTI)-based white matter mapping in brain research: a review. J Mol Neurosci 2008;34:51-61. [Crossref] [PubMed]
- Jang SH, Kim SH, Jang WH. Recovery of corticospinal tract injured by traumatic axonal injury at the subcortical white matter: a case report. Neural Regen Res 2016;11:1527-8. [PubMed]
- Yeo SS, Jang SH. Recvoery of an injured corticospinal tract and injured corticoreticular pathway in a patient with intracerebral hemorrhage. Neurorehabilitation 2013;32:305-9. [PubMed]
- Kwon HG, Choi BY, Chang CH, et al. Recovery of an injured corticospinal tract during a critical period in a patient with intracerebral hemorrhage. NeuroRehabilitation 2013;32:27-32. [PubMed]
- Wahl M, Lauterbach-Soon B, Hattingen E, et al. Human motor corpus callosum: topography, somatotopy, and link between microstructure and function. J Neurosci 2007;27:12132-8. [Crossref] [PubMed]
- Yamada K, Sakai K, Akazawa K, et al. MR tractography: a review of its clinical applications. Magn Reson Med Sci 2009;8:165-74. [Crossref] [PubMed]